Introduction
Fresh meat tenderness and stability have been studied and are considered the most important qualitative components (Alexandre et al., 2021). The quality of fresh meat decreases even when refrigerated (Kim et al., 2020). To increase meat tenderness and improve flavor, wet-aging technology has been widely used (Hwang and Hong, 2020).
Wet-aging can produce tender meat products since the meat structure is decomposed during aging, thereby affecting tenderness (Hwang et al., 2018). However, the aging time and abundant water in wet-aged meat product can accelerate deteriorations, thereby inducing protein and lipid oxidation during cooking or storage (Ali et al., 2021; Ismail et al., 2008). There are various techniques to inhibit or delay meat oxidation, such as addition of antioxidant, packaging methods, and application of coating (Kim et al., 2013; Zhang et al., 2013). The plant antioxidants like essential oil are preferred by consumer compared with synthetic antioxidants, and effectively inhibited oxidation. However, their distinct scent differed from meaty aroma can lead to the resistance to intake (Pateiro et al., 2018). Thus, the adequate process for preventing oxidation without compromise of quality is needed.
The coating can act as a barrier to oxygen and water, without negatively affecting the structure and aroma of meat. The coating solution can be made of polysaccharide, protein, and lipid; the material of coating solution influences the trait (Shin et al., 2022). Gelatin has high film forming ability, hence is suitable as edible coating on meat products (Jridi et al., 2018) and can reduce purge loss, color deterioration, and lipid oxidation of meat, thereby extending the shelf-life (Antoniewski and Barringer, 2010). For the application of coating to the meat, the concentration of coating solution is important, because it can influence the adherence to the meat surface, stability, and visible attributes (Bhagath and Manjula, 2019).
Recently, the use of high-intensity ultrasound to provoke changes in the chemical and physical properties of meat has emerged (Delgado et al., 2008). This technology can improve tenderness via microstructure changes, with minimal damage to meat, although varying results have been reported (Alarcon-Rojo et al., 2015). Specifically, there are mainly two conditions of ultrasound in the food industry: First, ultrasound was used at high frequencies (2–10 MHz) and low power (up to 10 W), a method used in the food market as it is non-destructive to the cell walls, although the effectivity rate was low (Soria and Villamiel, 2010). Second, ultrasound was used at low frequencies (20–100 kHz) and high power (100–10,000 W), which accelerates certain chemical reactions via microstructure alterations (Jayasooriya et al., 2004; Jayasooriya et al., 2007). In previous research, the sonication before the aging of meat has accelerated the proteolysis during the aging, and it has improved the tenderness (Stadnik et al., 2014).
There is little research on the effects of gelatin coating and sonication on the quality of wet-aged meat. Also, the proper concentration of gelation coating for wet-aged meat is not yet identified. Therefore, the objectives of this study are to determine the most suitable concentration combinations of gelatin and the effect of ultrasonic processing for manufacturing high quality wet-aged meat. Changes in microstructure influence meat quality and texture properties and can be demonstrated by correlating gelatin coatings with ultrasound.
Materials and Methods
Pork loins (longissimus lumborum) from castrated boars (Landrace×Yorkshire×Duroc) were obtained from a local processor, 48 h post-mortem. The pork loin was trimmed of all visible fat and cut into a regular form (thickness 3 cm) vertically to the pork loin muscle fiber direction. A total of six different treatments were then produced; the experimental design and formulation of each sample are listed in Table 1. The control wet-aged pork loin (G0) was formulated without a gelatin coating and sonication. The G0S treatment included only sonication. The other experimental treatments of wet-aged pork loins were formulated with gelatin coating. Gelatin coating and sonication were used as follows: G1, with 1% gelatin coating; G1S, with 1% gelatin coating and sonication; G5, with 5% gelatin coating; G5S, with 5% gelatin coating and sonication. First, the G1, G1S, G5, and G5S were soaked in 1% or 5% gelatin solution for 1 min, and surface of coated samples was dried at 25°C for 10 min. After that, all the slice of pork loins, including G0 and G0S, was vacuum packed. G0S, G1S, and G5S were sonicated using ultrasonic bath (CPX5900H-E, Emerson, Danbury, CT, USA), with 40 kHz and 160 W, for 30 min. After all these progresses, treatments were wet-aged for five days at a temperature below 10°C. The wet-aging of 30 meat blocks was conducted as triplicate.
G0 | G0S | G1 | G1S | G5 | G5S | |
---|---|---|---|---|---|---|
Pork loin | 100 | 100 | 100 | 100 | 100 | 100 |
Gelatin coating (%) | - | - | 1 | 1 | 5 | 5 |
Sonication | X | O | X | O | X | O |
The moisture content of the samples was analyzed via the AOAC methodology (950.46B; AOAC, 2000), using a drying oven (SAB-150, Haneul Science, Taebaek, Korea). The pH values of the samples were determined on a homogenate prepared with wet-aged pork loin sample (5 g) and 20 mL distilled water using a pH meter (Model 360, Mettler-Toledo, Schwerzenbach, Switzerland). The color (CIE L*, CIE a*, and CIE b*) of the wet-aged pork loin sample was determined using a colorimeter (Minolta Chroma meter CR-410, Konica Minolta, Osaka, Japan) that was calibrated with a white plate (CIE L*=+97.83, CIE a* =–0.43, CIE b*=+1.98, illuminate C).
Initially, the raw samples were weighed prior to the aging process, following which the wet-aging was progressed for 5 days. The wet-aged samples were re-weighed after a gentle swipe on the surface to remove the drip and coating solution. The aging loss percentage (%) was calculated using the weight difference between the raw and aged samples.
The weighed samples after the measurement of aging loss were put into unsealed thermostable plastic bag and cooked in water bath at 75°C for 1 h. After cooking, the cooking loss (%) was determined by the weight difference between uncooked and cooked wet-aged samples. WHC (%) was estimated by determining the expressible juice according to the filter paper press method (Kim et al., 2019). WHC was determined by calculating the released moisture content.
The samples were analyzed for TBARS using the methods established by Tarladgis et al. (1960) with minor modifications. Samples (10 g) were homogenized in distilled water (50 mL) for 2 min and transferred to a round flask with distilled water added (47.5 mL); 4 N HCl (2.5 mL) was added to the round flask with an antifoam agent and silicone o/w and distilled to a 50 mL distillate. A 0.02 M 2-thiobarbituric acid in 90% acetic acid (5 mL) was mixed with the distillate (5 mL) in a vial, capped, and heated (100°C, 30 min) to develop chromogen; the absorbance was determined at 538 nm. The value of TBARS was expressed as mg malondialdehyde/kg meat and it was calculated using conversion factor with 1,1,3,3-tetraethoxypropane and its recovery (Merlo et al., 2020; Tarladgis et al., 1960).
The MFI was determined as previously reported (Culler et al., 1978). Samples were extracted with MFI buffer solution, and the absorbance of the extract (0.5 mg protein/mL) was determined at 540 nm. The Warner–Bratzler shear force was determined using a TA-XT2i (Stable Micro System, Scarsdale, NY, USA) equipped with a Warner–Bratzler blade operating at a crosshead speed of 150 mm/min.
The protein solubility (sarcoplasmic protein, myofibrillar protein, and total protein) of the wet-aged pork loin samples was determined using Kim et al. (2022) methods, while the sarcoplasmic protein solubility was determined using the Biuret method (Gornall et al., 1949). The wet-aged pork loin sample (2 g) and ice-cold 25 mM potassium phosphate buffer (pH 7.2; 20 mL) were homogenized on ice and left to stand on a shaker overnight at 4°C. The mixtures were then centrifuged at 1,500×g for 20 min and the protein concentrations of the supernatants were determined. Total protein solubility was determined by homogenizing the wet-aged pork loin sample (2 g) in ice-cold 1.1 mol/L potassium iodide in a 100 mmol/L phosphate buffer (pH 7.2; 20 mL). Homogenization, shaking, centrifugation, and protein determination procedures are described as previous sarcoplasmic protein solubility. Myofibrillar protein solubility was determined using the difference between the total and sarcoplasmic protein solubilities.
All experimental data were analyzed with the SPSS statistics 20 software (SPSS, Chicago, IL, USA) using a completely randomized design. The Duncan’s range test was performed to determine the significant differences (p<0.05) and general linear model procedure was applied for identifying the interaction effect of coating and sonication. All experiments were proceeded at least three replicates. To compare the effects of wet-aging method, it was considered a fixed effect, and each replicate was considered a random effect.
Results and Discussion
Table 2 presents the moisture, pH, and color results of wet-aged pork loin with sonication and a gelatin coating. The moisture content of wet-aged pork loin with sonication and gelatin coating was the highest in the G5S sample (p<0.05), while the moisture content with sonication was higher (p<0.05) than that without sonication. Ultrasound treatment can improve moisture transport (Ozuna et al., 2013). Therefore, the moisture of coating solution may be transported to the meat via sonication. Jridi et al. (2018) have reported that gelatin coating of beef meat prevent moisture loss, while Antoniewski and Barringer (2010) indicated that the gelatin coating of fresh meat products decreased purge and purge loss in fresh meat since it acts as a water barrier. Chang et al. (2015) have reported that the water loss rate of sonicated beef increased with increasing ultrasound exposure. The results of this study indicated that the moisture content of control was not significantly different with 1% gelatin-coated wet-aged pork loin, but that moisture loss could be reduced when coated with 5% gelatin or sonication to uncoated pork loin.
The pH of wet-aged pork loin was increased by 1% gelatin coating (p<0.05), while the pH of wet-aged pork loin with 5% gelatin coating decreased. The ultrasonic treatment decreased the pH values in uncoated and 1% gelatin coated meat, however it enhanced the pH of 5% gelatin coated sample. Hereby, the gelatin concentration of coating and sonication are important factors to determine pH of meat. Jridi et al. (2018) have reported that the pH of beef meat with gelatin coating maintains pH values for eight days, while Stadnik and Dolatowski (2011) found that the pH of beef did not significantly differ between those treated with and without ultrasound, and that the pH of beef treated with ultrasound tended to increase with increasing aging time. In our study, the gelatin molecules in coating solution might immersed into the muscle by sonication and it can influence the pH decline. The sonication treatment can induce change of pH by decomposing the part of gelatin in coating solution and meat protein in pork loin (Jamalabadi et al., 2019). The pH variation by sonication can be affected by muscle type or sonication time (Valenzuela et al., 2021), thus it showed different tendency with previous study.
The CIE L* and CIE a* of wet-aged pork loin with a gelatin coating were lower (p<0.05) than that of G0, while the CIE L* of wet-aged pork loin with sonication was higher (p<0.05) than that of the samples without sonication. However, the CIE a* of wet-aged pork loin with sonication was lower (p<0.05) than that of the samples without sonication. The decreased CIE a* of sonicated treatments is caused by the metmyoglobin formation (Tang et al., 2006). The CIE a* of meat was usually decreased during the aging (Kim et al., 2018). Thus, the color preservation strategy might be needed for application of ultrasonic treatment. The CIE b* of wet-aged pork loin with sonication was higher (p<0.05) than that of the samples without sonication. The 5% gelatin coating was sharply decreased the CIE b* of wet-aged pork loin. Jridi et al. (2018) reported that the CIE b* of beef meat coated with gelatin was higher than uncoated sample after 3 days of storage. This difference can be also caused by the penetration of small molecules of gelatin in the high concentration of coating solution. Consequently, chromaticity of samples in this study might have been affected during wet-aging by gelatin coating and ultrasound.
As shown in Table 3, there was no significant difference between samples if only the proportion of coating or state of sonication was different (p>0.05). The low moisture permeability caused by aging methods decreased water evaporation during aging (Shi et al., 2020). A previous study found that the sonication process before aging did not significantly affect the drip loss of meat (Jayasooriya et al., 2004).
Significance of coating, sonication, and their interactions | ||||||
---|---|---|---|---|---|---|
Aging loss | Cooking loss | WHC | ||||
Coating | NS | *** | *** | |||
Sonication | NS | *** | *** | |||
Interaction effect (coating×sonication) | NS | NS | *** |
The cooking loss of wet-aged pork loin is also shown in Table 3. The cooking loss of G5S (wet-aged pork loin with 5% gelatin coating and sonication) was highest among all samples. Meanwhile, the cooking loss of the sample without any coating and sonication was the lowest. This might be the reason that the gelatin molecules which absorbed during aging were released out from the meat structure by heating. During the heating, the molecular mobility of gelatin was increased and the hydrogen bonding between the meat structure and absorbed gelatin was weaken (Anvari and Chung, 2016). Therefore, the gelatin molecules were released from the meat with the water molecules, which bonded with gelatin. The sonication promoted protein degradation in muscle structure which can increase water loss upon cooking (Purslow et al., 2016). Nevertheless, protein degradation is directly associated with the tenderness of the meat, and one of the major purposes of aging (Wang et al., 2018). In summary, the increased cooking loss of pork loin was induced by gelatin coating and protein degradation via sonication during aging.
The WHC of the wet-aged pork loin was the lowest in the 5% gelatin-coated and sonicated sample (Table 3). The WHC is highly related to the cooking loss of meat as it is associated with proteolysis (Kim et al., 2018). During aging, myofibrils are degraded by enzymes, while intracellular water is leaked into the extracellular space. Meanwhile, the wet-aged pork loin coated with 1% gelatin without sonication showed the highest WHC. This might be due to the high pH of G1, which was far from the isoelectric pH of protein. Boakye and Mittal (1993) also reported a positive correlation between pH and WHC during aging. Thus, the high cooking loss and low WHC of G5S were attributed to protein degradation, while the high WHC of G1 was attributed to the high pH.
TBARS have been used to evaluate the lipid oxidation of a meat product, and can affect the color, flavor, and texture of foods. During aging, structural changes in muscle can induce the production of malondialdehyde (Ali and Nam, 2021). The TBARS value of wet-aged pork loin was significantly decreased with coating (p<0.05) and not affected by sonication (p>0.05; Fig. 1, Table 4). Although all the treatments were identically vacuum packed, the coating significantly prevent the lipid oxidation of meat. The remained oxygen in package, even low concentration, might trigger the lipid oxidation of meat during the aging for 5 days. Also the cooking process of this study was performed in aerobic condition, thus the wet-aged meat was possible to oxidize during cooking. The coating of meat products is an effective method to prevent contact with water and oxygen (Duran and Kahve, 2020). The gelatin coating of pork meat investigated by previous studies showed high antioxidant activity (Gallego et al., 2020). Sonication treatments can generate free radicals, thereby accelerating lipid oxidation (Pinton et al., 2019). However, the ultrasound setting used in this study did not increase lipid oxidation. The similar result has been represented in previous study, where the ultrasound treatment did not affect the protein and lipid oxidation of meat emulsion (Cichoski et al., 2019). The protein structural changes derived from the sonication can determine the lipid oxidation (Cichoski et al., 2019). In this study, the coating with sonication might hinder the sonication-inducted transformation of protein structure, thus lipid oxidation of wet-aged pork loin can inhibit. Therefore, the gelatin coating with sonication of wet-aged pork loin effectively prevented lipid oxidation during aging.
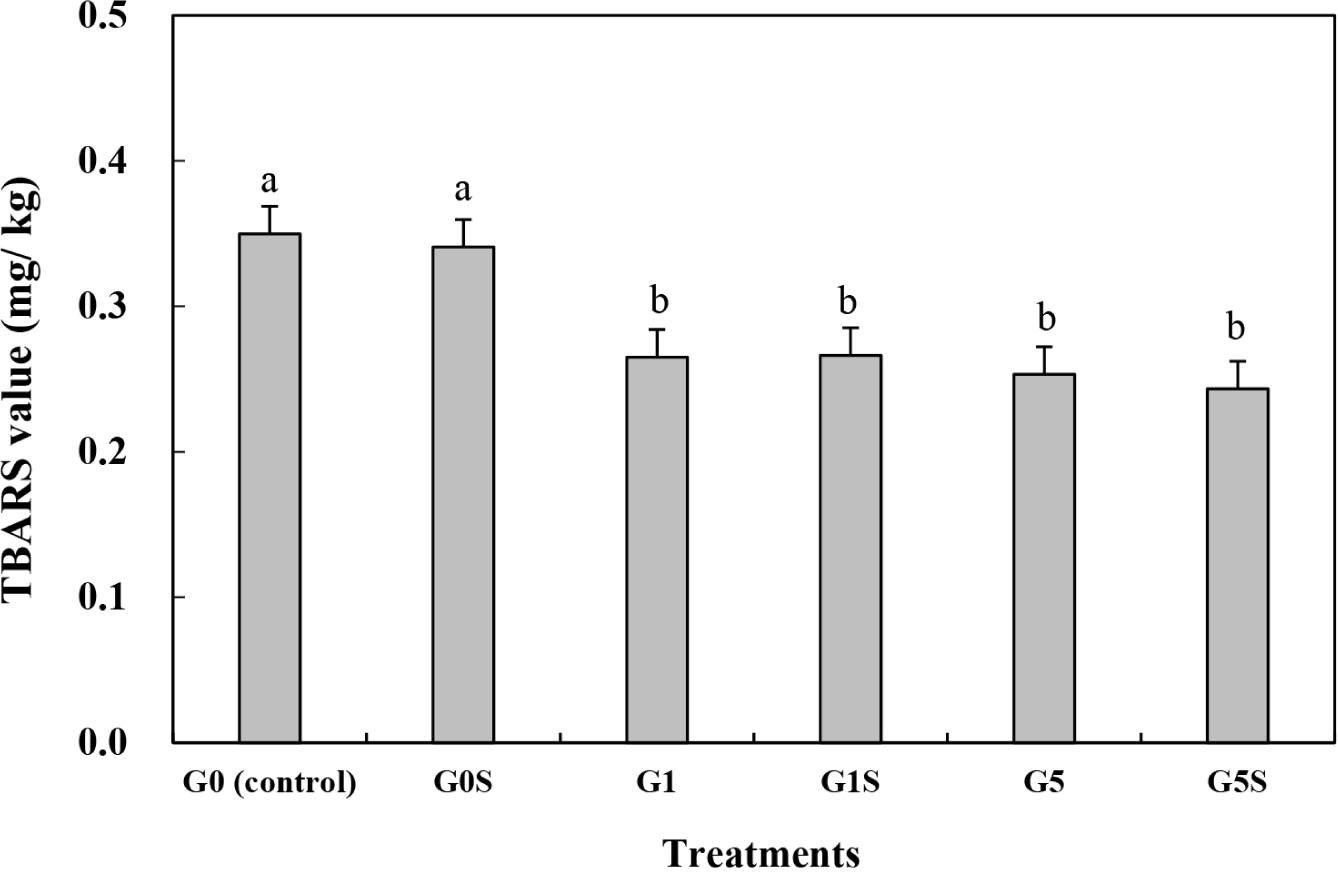
Myofibrillar protein fragmentation was affected by proteolysis during aging (Ali and Nam, 2021) and correlated with meat tenderization (Ilian et al., 2004). The MFI of wet-aged pork loin and the interaction effect of coating and sonication on MFI are shown in Fig. 2 and Table 4. The gelatin coating and sonication treatment significantly increased the MFI of the samples (p<0.05), regardless of coating proportion. Ultrasound treatment can break muscle cells and enhance enzymatic activity, thereby decreasing muscle fiber diameter (Alarcon-Rojo et al., 2015). Previous studies have reported similar results, wherein the ultrasound treatment to the pork loin increased the MFI, depending on treatment time (Yeung and Huang, 2017). Thus, the MFI results showed that the coated and sonicated wet-aged pork loin was considerably degraded.
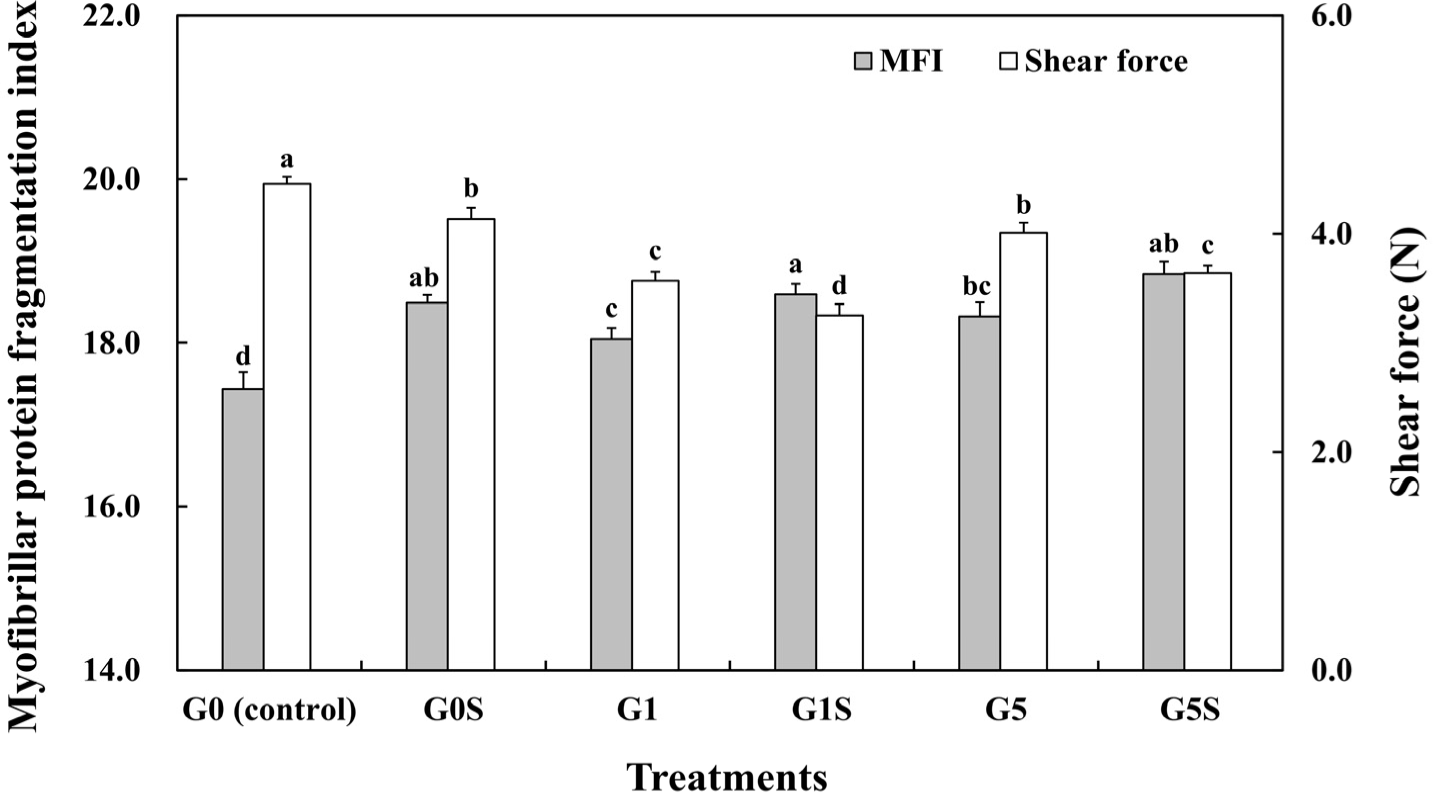
Shear force of wet-aged pork loin was significantly decreased as samples were coated and sonicated, although a shear force of 5% gelatin-coated samples was higher than that of 1% gelatin-coated samples (Fig. 2, Table 4, p<0.05). The shear force of meat was negatively correlated with myofibril fragmentation (Karumendu et al., 2009), which was induced by the low integrity of myofibril structure (Marino et al., 2013). In our study, the MFI was significantly increased by sonication (p<0.05), while previous findings have demonstrated that ultrasonic treatment could reduce the shear force values of muscles (Barekat and Soltanizadeh, 2017). Meanwhile, the reduced meat tenderness by the increased coating proportion in our study might have been attributed to water loss during cooking (Alexandre et al., 2021). The loss of water during cooking increased the stiffness of meat (Hughes et al., 2014), while high proportion coated samples showed high cooking loss and low water retention capacity compared to low proportion coated samples. Therefore, sonication with 1% gelatin coating significantly improved the tenderness of wet-aged pork loin.
The protein solubility of wet-aged pork loin and the interaction effect of coating and sonication on protein solubility are presented in Fig. 3 and Table 4. The myofibrillar and total protein solubilities were not significantly different among the samples (p>0.05), although the sarcoplasmic protein solubility of G1 was higher than G0 (0.05). Sarcoplasmic proteins have no structural function, however influence water retention capacity (Marino et al., 2014). Using NMR, Li et al. (2018) observed that sarcoplasmic protein solubility positively correlated with immobilized water and negatively correlated with free water in muscle. Thus, an increase in sarcoplasmic protein solubility could have influenced the WHC of G1. Protein solubility can also be affected by denaturation, oxidation, and aggregation of proteins (Khan et al., 2016). The sonication treatment, which increased MFI in this study, did not improve the protein solubility. According to packaging methods, a significant difference in protein solubility of pork during storage has been previously reported (Chen et al., 2015). Therefore, the 1% gelatin coating of wet-aged pork loin increased the sarcoplasmic protein solubility, while sonication treatment had no significant effect on protein solubility.
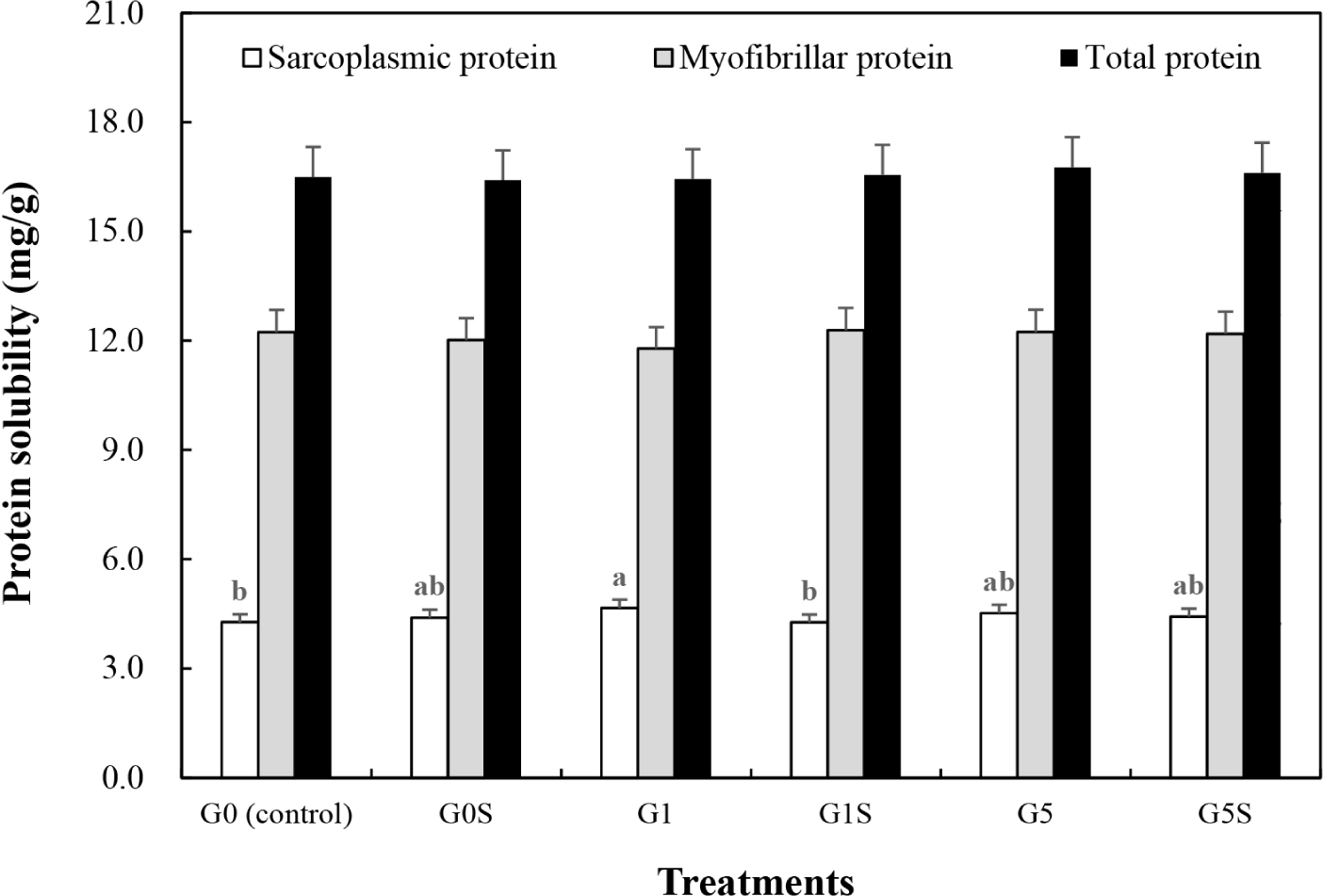
Conclusion
In this study, gelatin coating and sonication of wet-aged pork loin affected the physicochemical properties, WHC, lipid oxidation, myofibril fragmentation, tenderness, and protein solubility. Gelatin coating improved meat quality by increasing the tenderness and decreasing lipid oxidation. However, the high proportion of coating (5%) can increase the cooking loss and shear force of wet-aged pork loin, by decreasing water retention capacity. The sonication improved the tenderness by accelerating the proteolysis and did not affect lipid oxidation. The G1 showed higher moisture content and WHC than G1S, however G1S represented the lowest shear force among the treatment which was influenced by MFI. Therefore, a 1% gelatin coating is certainly effective way to improve the quality of wet-aged pork loin, and the sonication treatment can be useful for enhancing the tenderness without oxidative damages.