Introduction
The mucosal immunity serves as the first defense line against pathogenic organisms that can penetrate the physical barrier (Takahashi et al., 2021). Gut-associated lymphoid tissue (GALT), a specialized secondary lymphoid tissue around the intestine, is often referred as a model tissue to visualize the critical function of mucosal immunity because the interaction between gut bacteria and immune cells in GALT can affect both local mucosal immunity and systemic immunity (Mörbe et al., 2021).
Immunoglobulin A (IgA) is produced when immune cells within GALT are activated by foreign antigens (Mörbe et al., 2021). It has been proposed that initial production of IgA is caused by exposure to commensal bacteria soon after birth (Chen et al., 2020). IgA provides many beneficial aspects in the mucosal immunity against foreign antigens mainly by exhibiting neutralizing activities against gut bacteria or their soluble factors (Chen et al., 2020). Supporting this idea, IgA deficiency in humans is associated with various diseases including infection, autoimmune disease, allergy, and cancer (Swain et al., 2019).
Recent evidence suggests that administration of certain strain of lactic acid bacteria (LAB) such as Lacticaseibacillus rhamnosus GG can facilitate IgA secretion by stimulating immune cells within GALT (Jin et al., 2021). Moreover, IgA induction by Lactiplantibacillus pentosus b240 can make mice become more resistant to influenza virus or Streptococcus pneumoniae infection (Kiso et al., 2013; Tanaka et al., 2011). These results implied that produced IgA after stimulation with LAB in GALT could protect infection against microbial pathogens through systemic circulation. Therefore, the oral intake of certain LAB might benefit the host through increased protection against certain microbial pathogens by enhancing mucosal immunity. However, most of study describing the enhancement of IgA production by LAB just focused on the efficacy of LAB without any mechanical insights.
In this study, we screened 25 different strains of LAB isolated from traditional fermented Korean foods based on their abilities to induce IgA secretion in vitro and in vivo. Results clearly indicated that some of LAB stimulate lamina propria cells (LPCs) from Peyer’s patch to secret IgA via toll-like receptor (TLR) / interleuckin-6 (IL-6) axis. Further, we demonstrated that oral stimulation by these LAB increased systemic IgA levels including levels of GALT IgA, bronchus-associated lymphoid tissue (BALT) IgA and serum IgA in mice.
Materials and Methods
Twenty-five different strains of LAB were isolated from several traditional fermented Korean foods according to previously established method with a slight modification (Lee et al., 2011). Briefly, modified MRS agar plates (pH 5.0; BD Biosciences, San Diego, CA, USA) were used for culturing all samples at 37°C for 48 h under anaerobic conditions to enrich LAB. Single colonies were selected from these plates, cultured again on MRS broth (BD Biosciences), and characterized by Gram-staining and utilization of carbohydrate source. For species identification, 16S rDNA of each strain of LAB was sequenced and aligned using an established DNA database (http://www.ncbi.nlm.nih.gov/BLAST; Lee et al., 2011). Identified species and strain names are listed in Table 1. Each strain of LAB was sub-cultured more than three times before experiments.
2) Positive, growth or survival; negative, no growth or survival compared with initial inoculums. Initial inoculums at approximately 1×108 CFU/mL.
Growths of each strain of LAB under low pH and bile content were measured according to previously established method (Lee et al., 2011). Briefly, each strain of LAB (1.0×108 CFU/mL) was cultured in acidic MRS broth (final pH 2.5) containing 1,000 units/mL of pepsin (Sigma-Aldrich, St. Louis, MO, USA) for 3 h at 37°C or MRS broth containing 0.3% oxgall (Sigma-Aldrich) for 24 h at 37°C. After culture, 100 μL of broth was plated in triplicate onto MRS agar to measure final CFU.
For adhesion assay, 4×104 cells/well of HT-29 cells (human epithelial cells; American Type Culture Collection, Manassas, VA, USA) in RPMI-1640 (Sigma-Aldrich) supplemented with 10% fetal bovine serum (Sigma-Aldrich) were plated into 24-well tissue culture plates. These cells were then incubated with 1×108 CFU/mL of each LAB strain at 37°C. After 3 h, cells were washed with PBS six times. After washing, bacteria that attached to HT-29 cells were harvested by repeatedly pipetting with chilled sterile water and cultured on MRS agar plates to measure final CFU. Sources, probiotic properties of identified species and strain names are summarized in Table 1. Each strain of LAB was sub-cultured more than three times prior to in vitro experimental analysis.
For measuring hemolytic activity, each strain of LAB was inoculated on BD BBLTM prepared plated medium (TrypticaseTM soy agar with 5% sheep blood; Thermo Fisher Scientific, Waltham, MA, USA) and incubated at 37°C for 48 h. After 48 h, the colonies on plates were characterized to observe the hemolytic pattern (Fu et al., 2022). To detect urease activity, each strain of LAB was inoculated on RemelTM urea agar base (Thermo Fisher Scientific) and incubated at 37°C for 48 h. After 48 h, the color changes of plates were monitored to observe the urease activity (Christensen, 1946). For gelatin liquefaction test, each strain of LAB (5.0×106 CFU/mL) was cultured in MRS gelatin broth containing 12% (w/v) of gelatin for 48 h at 37°C. After 48 h, the cultured MRS gelatin broths were incubated at 4°C to monitor gelatin liquefaction (Fugaban et al., 2022).
BALB/c, C3H/HeJ, and C3H/HeN mice (female, 6–8 weeks old) were purchased from Central Lab Animal Incorporation (Seoul, Korea) and acclimated for one week prior to experiments. Mice were fed a standard rodent diet with purified water ad libitum and kept at 20°C–24°C with 40%–60% humidity in Korea University animal facility under a 12 h/12 h light-dark cycle. Mice received proper care in accordance with a protocol approved by the Institutional Animal Care and Use Committee (IACUC) of Korea University (protocol numbers: KUIACUC-2016-160 and KUIACUC-2017-107).
LPCs from Peyer’s patches were isolated from the small intestine of BALB/c, C3H/HeJ, or C3H/HeN mouse according to previously established method (Kikuchi et al., 2014). Isolated LPCs (2.5×105 cells/well) were then mixed with each heat-killed LAB (5×106 CFU/mL) and seeded into 96 well plates in 200 μL of RPMI 1640 medium supplemented with 10% (v/v) FBS and 1% penicillin/streptomycin. After 7 days of incubation, LAB-stimulated LPCs were analyzed for TLR2 and TLR4 expression by quantitative real-time polymerase chain reaction (PCR) and visualization of IgA positive B cells (IgA+B220+ cells) by flow cytometry analysis. Culture supernatant of each sample after co-culture for 7 days was harvested. Sandwich enzyme‐linked immunosorbent assays (ELISAs) were performed to detect IgA (Cat # 88-50450-22, Thermo Fisher Scientific), IL-6 (Cat # BMS603-2, Thermo Fisher Scientific), poly Ig receptor (pIgR; Cat # SEK50119, Sino Biological, Wayne, PA, USA), and transforming growth factor-β (TGF-β; Cat # BMS608-4, Thermo Fisher Scientific) using ELISA kits according to each manufacturer’s instructions. For blocking TLR2 signaling, neutralizing antibody against mouse TLR2 (Cat # 121802, BioLegend, San Diego, CA, USA) was added into the LPC culture.
Total RNAs were isolated from cultured LPCs and then cDNAs were synthesized using total RNAs, oligo-dT primers, and a First-Strand cDNA Synthesis Kit (SuperScript RT; Thermo Fisher Scientific). Quantitative real-time PCR was then performed with each cDNA as a template using a QGreenTM 2×qPCR Master Mix (GenDEPOT, Katy, TX, USA) on a Bio-Rad CFX96 Real-Time Detection System (Bio-Rad Laboratories, Hercules, CA, USA). PCR primer sequences for mouse Tlr2 (GenBank accession NM_011905) were as follows: 5’-TTGCTCCTGCGAACTCCTATCC-3’ (sense), 5’-AGTCACAC AGGTAGCTGTCTGG-3’ (anti-sense), resulting in a RT-PCR product of 89 bp in length. PCR primer sequences of mouse Tlr4 (GenBank accession JX878359.1) were as follows: 5’-GCCGGAAGGTTATTGTGGTAGTG-3’ (sense), 5’-GGACAAT GA AGATGATGCCAGAGC-3’ (anti-sense), resulting in a 123 bp RT-PCR product. Relative expression levels of analyzed genes were normalized to mouse Gapdh level. PCR primer sequences of mouse Gapdh (GenBank accession NM_008084) were as follows: 5’-ATGGTGAAGGTCGGTGTGAA-3’ (sense), 5’-GGTCGTTGATGGCAACAATCTC-3’ (anti-sense), resulting in a 100 bp RT-PCR product.
To visualize IgA positive B cells (IgA+B220+ cells), a single cell suspension from cultured LPCs was stained with FITC-conjugated anti-IgA (Cat # 559354, BD Biosciences, San Jose, CA, USA) and PE-conjugated anti-B220 (Cat # 553090, BD Biosciences). After staining, cells were washed, resuspended in PBS, and analyzed by flow cytometry using a FACSCalibur with CellQuest software (BD Biosciences; Choi et al., 2017).
Sixty Balb/c mice were randomly separated into ten groups (n=6 mice per group): Two control (PBS) groups and eight test (LAB) groups. For the two control groups, each group of mice was orally administered 400 μL of PBS every day for 7 days or 21 days, respectively. For the eight test groups, each group of mice was orally administered 400 μL of PBS containing four different strains of LAB (CJW55-10, CJW18-6, CJW56-11, or CJN2696) every day for 7 days or 21 days, respectively. At 7 or 21 days after oral administration, mice were sacrificed to analyze levels of BALT IgA, GALT IgA, serum IgA, and serum IgG using sandwich ELISA kits (Cat # 88-50400-22, Thermo Fisher Scientific). For preparing samples of LAB in animal studies, each strain of cultured LAB was freeze-dried and keep at –80°C. Each sample of LAB (1×1010 CFU) was then dissolved in 400 μL of PBS just before oral administration.
To measure serum IgA and IgG levels in experimental animals, blood specimens from experimental mice were collected from infraorbital veins using capillary tubes on day 7 or 21 after the first oral administration of each strain of LAB. To obtain serum samples, each blood sample was incubated on ice for 1 h and centrifuged at 8,000×g for 10 min. Each serum sample was then diluted 1:100 with PBS for measuring IgA and IgG levels (Choi et al., 2017). Sandwich ELISA kits were used to measure total serum IgA (Cat # 88-50450-22, Thermo Fisher Scientific) and IgG (Cat # 88-50400-22, Thermo Fisher Scientific) levels according to the manufacturer’s instructions.
To measure BALT IgA, bronchoalveolar lavage was performed with 2 mL PBS after exposing the tracheae of sacrificed mice. Bronchoalveolar lavage fluids were then centrifuged at 800×g for 5 min at 4°C (Choi et al., 2018). Levels of IgA in bronchoalveolar lavage fluids were quantitated using sandwich ELISA kits (Cat # 88-50450-22, Thermo Fisher Scientific).
To measure GALT IgA, LPCs from Peyer’s patches were isolated from the small intestine of each sacrificed mouse according to previously established method (Kikuchi et al., 2014). Isolated LPCs (2.5×105 cells/well) were then mixed with each heat-killed LAB (5×106 CFU/mL) and seeded into 96-well plates in 200 μL of RPMI 1640 medium supplemented with 10% (v/v) FBS and 1% penicillin/streptomycin. After 2 days of incubation, culture supernatants of each sample were harvested and sandwich ELISAs were performed to detect IgA levels using ELISA kit (Cat # 88-50450-22, Thermo Fisher Scientific).
Results
Twenty-five different strains of LAB were isolated from traditional fermented Korean foods and identified at species level by 16S rDNA sequencing (Table 1). Subsequently, these LAB were characterized for their basic properties as probiotics such as pH tolerance, bile tolerance, and adherence to intestinal epithelial cells (HT-29 cells). All LAB failed to survive or proliferate after three-hour incubation at pH 2.5 (Table 1). However, bile tolerance over 24 h was observed for several strains (Table 1). Also, several strains exhibited adhesive properties to human intestinal epithelial cells (HT-29 cells) after three-hour co-culture (Table 1). Next, hemolytic pattern, urease activity and gelatinase activity of these LAB were determined for the preliminary safety evaluation. All the tested strains exhibited γ-hemolytic pattern without activities of both urease and gelatinase (Table 1). To examine immunomodulatory activity, these LAB were examined for their abilities to stimulate LPCs from Peyer’s patches to produce IgA. Among them, Lactiplantibacillus plantarum CJW55-10, L. pentosus CJW18-6, L. pentosus CJW56-11, and Pediococcus acidilactici CJN2696 were found to be strong IgA inducers because soluble IgA levels were significantly increased when LPCs were co-cultured with these LAB (Table 1). Supporting this result, IgA secreting B cells (IgA+B220+ cells) were significantly increased when these LAB were co-cultured with LPCs (Fig. 1A). However, total B cells (B220+ cells) of LPCs were not significantly changed after co-culture with these LAB (Fig. 1B).
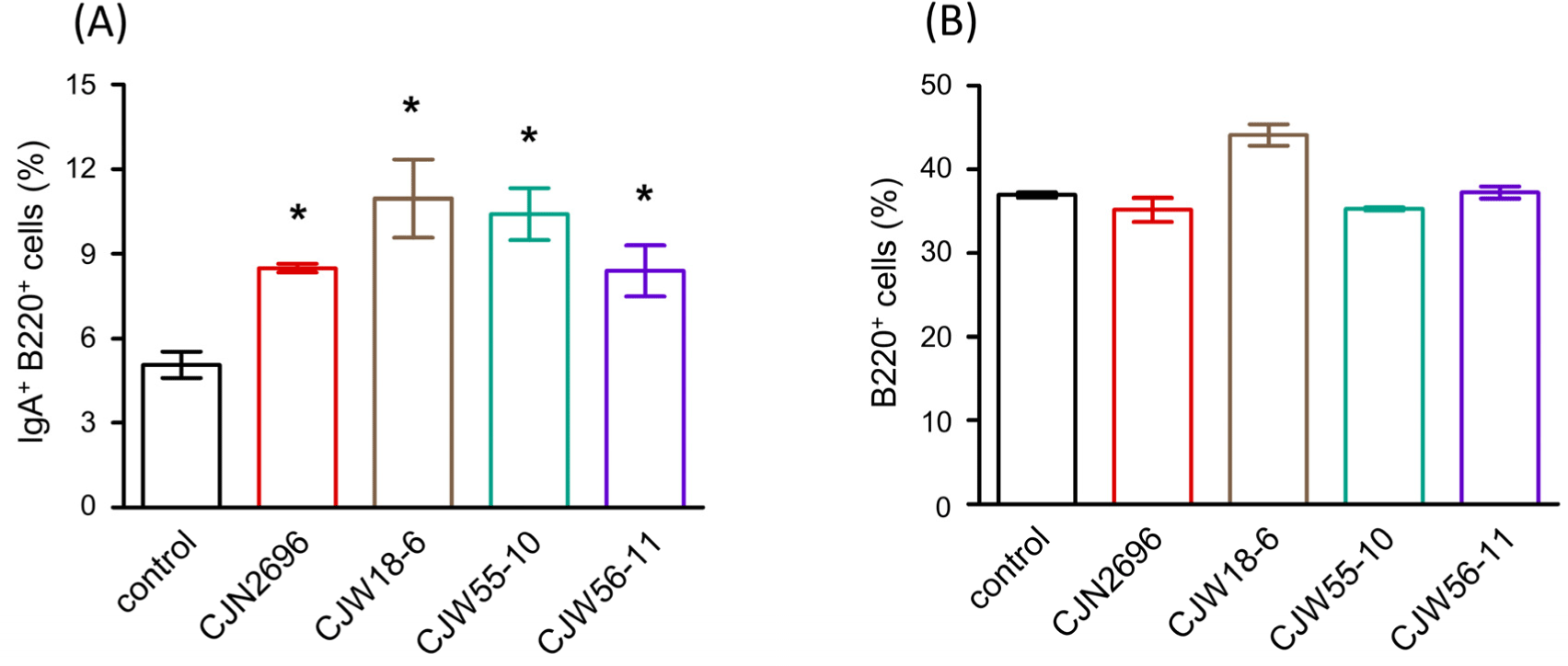
To determine how these LAB could stimulate IgA secretion by LPCs, relative expression levels of TLR2 and TLR4 of LPCs stimulated with these LAB were monitored by quantitative real-time PCR analyses because previous observation indicated that TLR2- and TLR4- mediated signaling could enhance IgA immune response during bacterial infection (Li et al., 2019). As shown in Fig. 2A and B, relative expression levels of TLR2 and TLR4 of LPCs stimulated with these LAB were significantly increased compared to those of unstimulated control (control). Subsequently, ELISAs were performed to determine IL-6 and TGF-β levels in culture supernatants from LPCs stimulated with these LAB. Levels of IL-6 were found to be significantly elevated in culture supernatants from LPCs stimulated with these LAB compared to those in unstimulated control LPCs (control; Fig. 2C). However, TGF-β levels in culture supernatants from LPCs stimulated with these LAB were not significantly different from those in unstimulated control LPCs (Fig. 2D). We also monitored expression levels of pIgR in culture supernatants from LPCs stimulated with these LAB. Results indicated that pIgR expression levels in LPCs stimulated with these LAB were not significantly different from those in unstimulated LPCs (Fig. 2E).
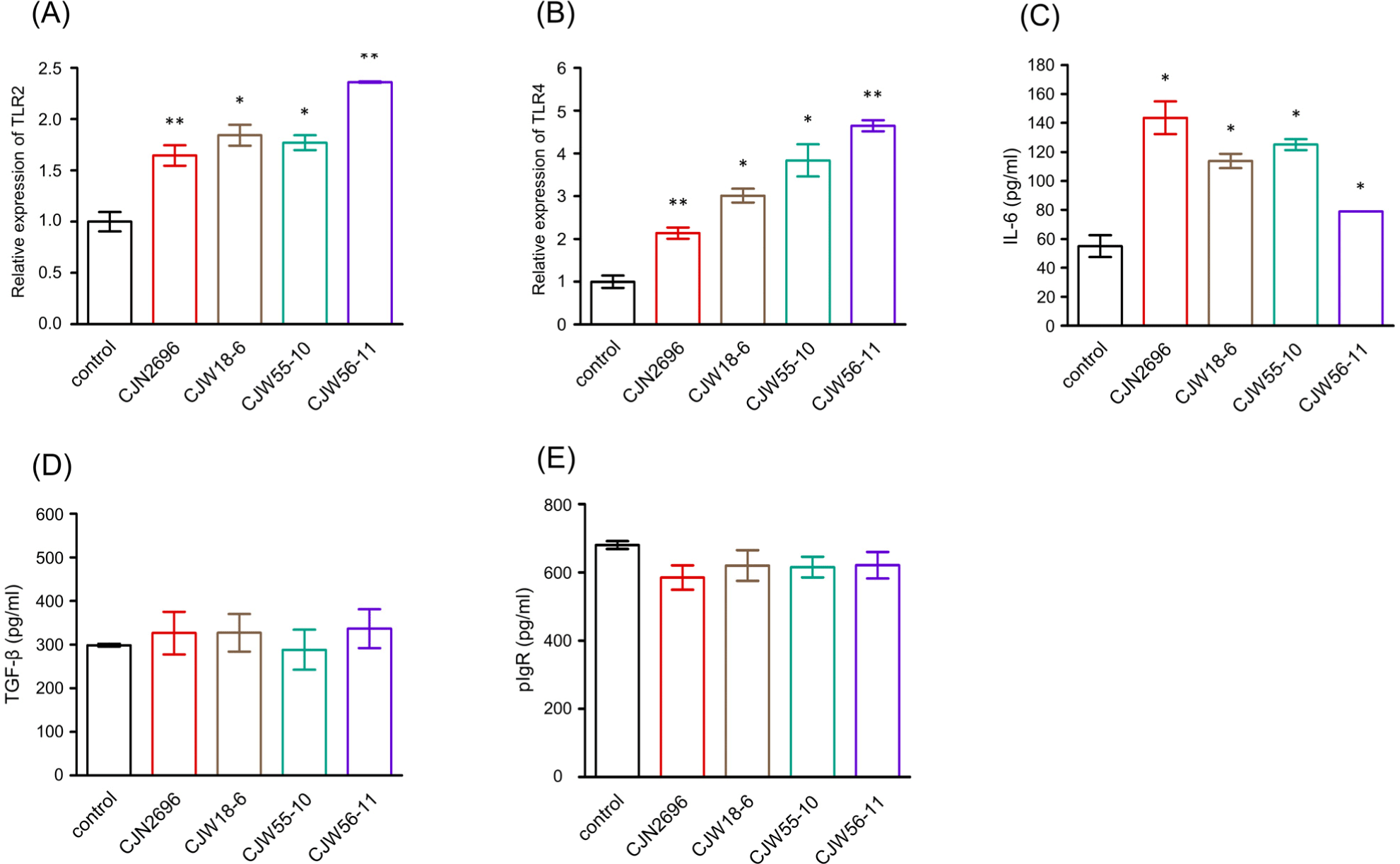
To determine whether TLR2 or TLR4 signaling affected IgA production in LPCs stimulated by these LAB, LPCs from Peyer’s patches of TLR4 null mice (C3H/HeJ) or wild-type TLR4 (C3H/HeN) mice were isolated and stimulated with CJN2696 in the presence of neutralizing antibody against TLR2 (Hagberg et al., 1984). Seven days after co-culture, levels of IgA and the frequency of IgA secreting B cells (IgA+B220+ cells) were measured using ELISAs and flow cytometry analyses, respectively. There was no significant difference in IgA production or frequency of IgA+B220+ cells between CJN2696-stimulated LPCs from C3H/HeJ or those from C3H/HeN mice (Figs. 3A and B). However, anti-TLR2 antibody treatment severely impaired IgA production in both CJN2696-stimulated LPCs from C3H/HeJ and those from C3H/HeN mice (Fig. 3A). Supporting this result, frequencies of IgA+B220+ cells from CJN2696-stimulated LPCs of both C3H/HeJ and C3H/HeN mice were significantly decreased in the presence of anti-TLR2 antibody (Fig. 3B). Therefore, CJN2696 may induce IgA and IL-6 secretion through TLR2.
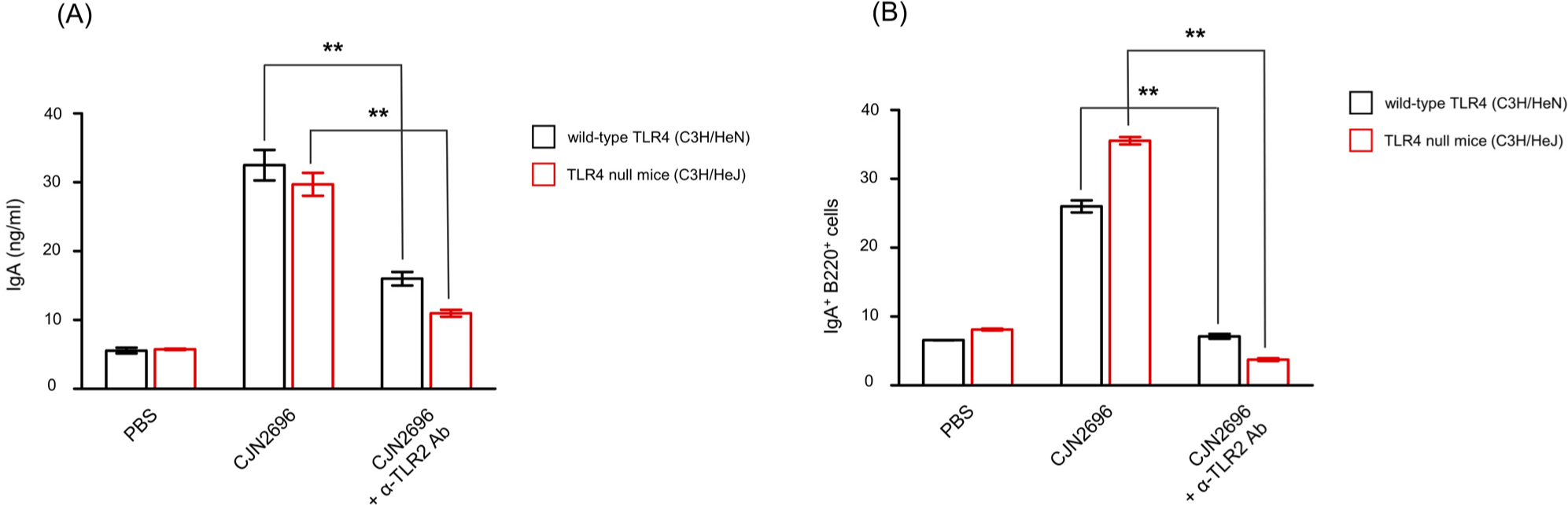
Further, we tested whether oral uptake of selected LAB increased IgA production in vivo. After 7 days of daily oral administration, IgA levels in GALTs and BALTs of CJN2696, CJW18-6, and CJW55-10 feed animals were elevated compared to those in control mice (Figs. 4A and B). IgA levels in GALTs of these LAB feed mice were comparable between one-week and three-weeks of oral administration (Fig. 4A). Interestingly, IgA levels in BALTs of these LAB feed mice after three-weeks of daily oral administration were slightly decreased compared to those of one-week feed mice (Fig. 4B). Serum IgA levels were increased in CJN2696 feed mice after one-week or three-week administration and in CJW55-10 feed mice after three-week administration compared to those in control mice (Fig. 4C). However, serum IgG levels were not significantly changed before or after administration of these LAB (Fig. 4D). These results indicate that 7 day administration of selected LAB is enough to increase not only mucosal IgA, but also serum IgA in mice.
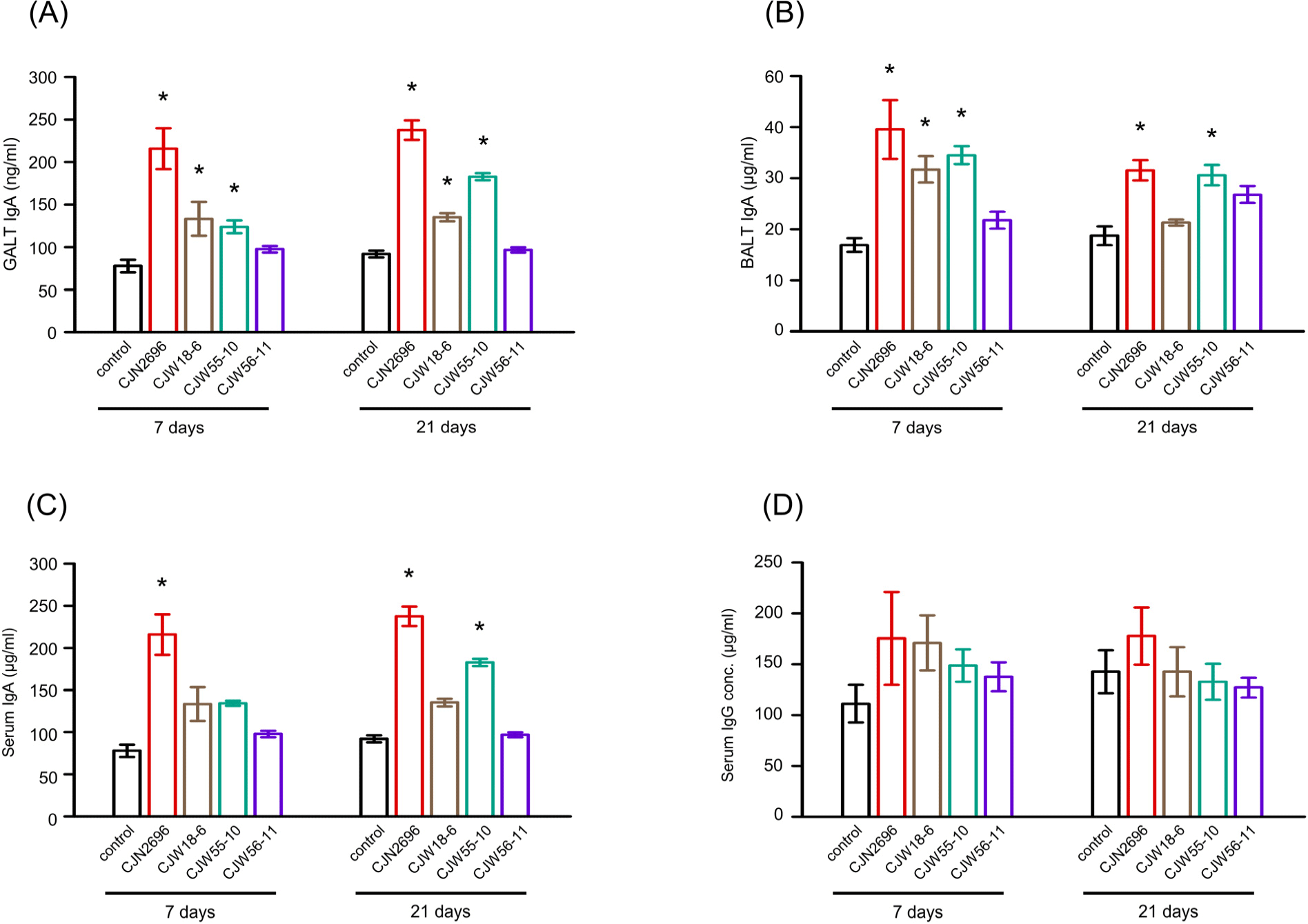
Discussion
In this study, we screened various strains of LAB isolated from traditional fermented Korean foods based on their abilities to enhance IgA production of LPCs from Peyer’s patch and measured the efficacy of IgA production after oral administration of selected LAB. Most of these LAB isolated from traditional fermented Korean foods were Levilactobacillus brevis, Lacticaseibacillus casei, L. pentosus, and L. plantarum. Since all identified species of LAB are widely used as probiotics, traditional fermented Korean foods might provide a good probiotic repertoire as a functional food supplement to modulate human physiology (De Filippis et al., 2022).
When LPCs from Peyer’s patch were co-cultured with these LAB, L. plantarum CJW55-10, L. pentosus CJW18-6, L. pentosus CJW56-11, and P. acidilactici CJN2696 induced strong IgA secretions by increased production of IL-6 and elevated number of IgA secreting B cells (IgA+B220+ cells). Among various cytokines, IL-6 is essential for IgA production of mucosal B cells and TGF-β is involved in mediation of antibody isotype switch to IgA (Ramsay et al., 1994). Especially, IL-6 is indispensable for mucosal IgA production since IL-6 deficient mice exhibit significantly decreased level of IgA with much reduced number of IgA secreting B cells (IgA+B220+ cells) in MALT. However, TGF-β is not essential for the production of IgA since the proper number of IgA secreting B cells (IgA+B220+ cells) were still present in MALT of mice lacking the TGF-β receptor in B cells (Borsutzky et al., 2004). Consistent with these observations, our results demonstrated that only IL-6, but not TGF-β, was increased when LPCs were co-cultured with these LAB. Notably, the proportion of IgA secreting B cells (IgA+B220+ cells) was increased while the proportion of total B cells (B220+ cells) was not changed when LPCs were co-cultured with these LAB. This result might suggest that some molecular patterns derived from these LAB increased the proportion of IgA secreting B cells (IgA+B220+ cells) instead of facilitating the proliferation of whole LP B cells. Supporting this idea, the number of immature B cells (IgM+B220+ cells) in MALT were significantly elevated in IL-6 deficient mice compared to those in wild-type mice (Ramsay et al., 1994). Thus, combined evidence may suggest that IL-6 function is involved in terminal differentiation into IgA secreting B cells, rather than maintenance or proliferation of B cells in MALT (Ramsay et al., 1994).
TLR2 signaling is well-known to trigger host cell responses against Gram-positive bacteria because peptidoglycan and lipoteichoic acid derived from these bacteria are recognized by TLR2 (Schwandner et al., 1999). TLR4 can initiate host responsiveness against Gram-negative bacteria since TLR4 can recognize lipopolysaccharide (Tapping et al., 2000). However, both TLR2 and TLR4 are up-regulated in LPCs stimulated with selected LAB in our study. Previous observation has also indicated that TLR4 signaling may participate in the responsiveness against Gram-positive bacteria in vitro (Takeuchi et al., 1999). Interestingly, previous observation showed that TLR4 mRNA expression in human monocytes was substantially decreased by tumor necrosis factor-α treatment, but dramatically increased by IL-6 treatment (Tamandl et al., 2003). Therefore, it is possible that the elevated level of TLR4 expression in LPCs is due to the exposure of IL-6 in the culture supernatant. Since both TLR2 and TLR4 signaling trigger the production of IL-6 through activation of transcription factors such as nuclear factor-kB or interferon-regulatory factors (Zakeri and Russo, 2018), we further examined the effect of TLR2 or TLR4 signaling on the production of IgA by CJN2696 stimulated LPCs. Results clearly indicated that CJN2696 could facilitate IgA and IL-6 production through TLR2. Similar mechanism has been reported for L. pentosus b240 in stimulating IgA secretion (Kotani et al., 2014). In addition, it is known that pIgR makes a complex with IgA produced within LP and facilitates a transport of IgA to luminal side of mucosal barrier (Kaetzel, 2005). However, the level of pIgR was not changed when LPCs were co-cultured with these LAB.
Based on our study, P. acidilactici CJN2696 is identified as the most powerful IgA stimulant among all LAB tested in this study. P. acidilactici is a facultative anaerobic Gram-positive coccus commonly found in fermented vegetables, fermented dairy products, and meat (Papagianni and Anastasiadou, 2009). Although the usage of P. acidilactici strain is limited as a human supplementary diet, P. acidilactici has been used as an animal feed additive to improve growth performance (Arsène et al., 2021). Also, some strains of P. acidilactici can produce pediocin, a powerful bacteriocin that can effectively kill a broad range of pathogenic bacteria (Fugaban et al., 2022). Therefore, selected strains of P. acidilactici have the potential as probiotics to improve human health.
In our molecular model, some molecular patterns derived from selected LAB are recognized by pattern recognition receptors such as TLR2 or TLR4. Then, TLR signaling triggers induction of IL-6. Promptly, TLR / IL-6 axis enhance IgA secretion by increasing the proportion of IgA secreting B cells (IgA+B220+ cells). Furthermore, we demonstrated that daily oral intake of selected LAB after 7 days could increase systemic IgA levels including serum and mucosal IgA found in GALT as well as BALT.
Further study is needed to for structural identification of cell wall components of these LAB for stimulating IgA production and examining protective roles of selected LAB during infection of certain microbial pathogens. Also, additional studies required to monitor changes of gut microbiome populations after the consumption of selected LAB to find out any specific gut microbiome-related linkage for immune modulation. Finally, whole-genome analyses or safety assessment of these LAB needs to be performed because previous studies have clearly shown that LAB even if traditionally were considered as safe, some specific strains can be caring virulence genes and be of potential health hazard (Wang et al., 2021).
Conclusion
In this study, we identified four different strains of LAB which induce IgA secretion by LPCs via TLR / IL-6 signaling pathway in Peyer’s patch. Further, we provided evidence that oral administration by these strains of LAB led to increase the levels of GALT IgA, BALT IgA, and serum IgA in mice. Therefore, oral intake of selected strains of LAB can increase systemic IgA secretion in mice.