Introduction
Meat consumption per capita and total meat consumption are increasing worldwide (Baek et al., 2020b). Meat and meat products are a good environment for the growth of microorganisms (Heredia and García, 2018), and can be easily contaminated with food-borne pathogens during their manufacture, storage, and distribution (Yoo et al., 2021). Contamination of meats can cause diseases such as food poisoning, which is caused by the ingestion of pathogens and microbial toxins. The symptoms of food poisoning include abdominal pain, diarrhea, vomiting, and fever; in severe cases, it can even cause death (Gourama, 2020). Therefore, to consume meat products safely, food safety must be ensured, for which technology to effectively control microorganisms is required.
Thermal treatment is one of the representative technologies for inactivating foodborne pathogens and ensuring food safety. However, it degrades the nutritional and sensory qualities of food (Heo et al., 2021). Therefore, there is a need for technology that can efficiently control pathogenic bacteria and minimize the negative effects on the nutritional and sensory quality of meat products. Non-thermal treatment, unlike thermal technology, can minimize the nutritional and sensory quality degradation of food (Jadhav et al., 2021). Non-thermal technologies include physical techniques, such as ultrasonication, plasma technology, irradiation, pulsed electric field, and high-pressure processing (Jadhav et al., 2021; Osae et al., 2020), as well as chemical methods, such as the use of chlorine-based sanitizers, organic acids, and bacteriocins (Demirok et al., 2013; Mani-López et al., 2012; Min et al., 2007).
Among these technologies, nisin is a peptide produced by a specific strain of Lactococcus lactis, which has been designated generally recognized as safe by the Food and Drug Administration (FDA). It exhibits microbial inactivation properties, and is the most commonly used bacteriocin (Zhao et al., 2020). Nisin causes leakage of intracellular bacterial components, leading to the death of bacterial cells (Tong et al., 2014). It exhibits high antibacterial activity against gram-positive bacteria. However, the lipopolysaccharide layer in the outer membrane of gram-negative bacteria confers resistance to nisin, limiting its efficacy against gram-negative bacteria (Liang et al., 2020). This limitation can be overcome by combining it with other technologies that can destroy the outer membrane (Wang et al., 2018). Some studies have been conducted to improve the antibacterial activity of nisin against gram-negative bacteria by damaging the integrity of the outer membrane in combination with an antibacterial agent (Zhao et al., 2020) or physical technology, such as electric field, high-pressure processing, ultrasound, and plasma (Costello et al., 2021; Gallo et al., 2007; Li et al., 2016; Liao et al., 2018; López-Pedemonte et al., 2003; Mok et al., 2020; Novickij et al., 2018; Pokhrel et al., 2019; Ukuku et al., 2019).
Cold atmospheric pressure plasma (APP) is a non-thermal sterilization technology that use ionized gas under quasi-neutral conditions (Lee et al., 2011). According to Yong et al. (2015), in can be seen that plasma can effectively inactivate Escherichia coli (E. coli), Salmonella Typhimurium, and Listeria monocytogenes (L. monocytogenes). APP generates various UV photons, electrons, positive and negative ions, atomic species, and free radicals (Kim et al., 2020). In particular, reactive species such as superoxide anions, hydroxyl radicals, nitric oxide, and ozone generated through plasma (Yong et al., 2015) cause cell membrane damage via physical and chemical changes, such as lipid peroxidation of cell membranes, which ultimately induces cell leakage and lead to cell death (Zhao et al., 2022). In addition, owing to structural differences, such as the peptidoglycan layer of gram-positive and gram-negative bacteria, APP exhibits a greater bactericidal effect against gram-negative bacteria than gram-positive bacteria (Yoo et al., 2021). Therefore, the bactericidal effect of APP seems to overcome the problems related to the resistance of gram-negative bacteria to nisin. In addition, there are few studies on improving the bactericidal effect of the combination of plasma and nisin treatment (Costello et al., 2021; Ukuku et al., 2019). However, this phenomenon needs to be investigated in more detail.
Therefore, in this study, combined treatment with APP and nisin was performed to determine their bactericidal effect on meat products. To achieve this, the effect of APP and nisin combination on the reduction of E. coli O157:H7 and L. monocytogenes was evaluated. The APP and nisin combination was then applied beef jerky and sliced ham inoculated with E. coli O157:H7 to confirm its bactericidal effect.
Materials and Methods
The encapsulated atmospheric pressure dielectric barrier discharge plasma described by Kang et al. (2022) was used. Briefly, the plasma device was fabricated by attaching copper electrodes and a polytetrafluoroethylene sheet to a rectangular plastic container (137×104×53 mm) and a lid. The sample (bacterial solution or inoculated meat product) was placed in a glass dish in the plasma device, and discharge was performed at 2.2 kHz and 8.4 kV using atmospheric air.
E. coli O157:H7 (NCCP 15739) and L. monocytogenes (ATCC 19111) were obtained from the National Culture Collection for Pathogens (Cheongju, Korea) and Korean Culture Center of Microorganisms (Seoul, Korea), respectively. Single colonies of E. coli O157:H7 and L. monocytogenes were transferred into 25 mL tryptic soy broth (TSB) and TSB containing yeast extract (YE), respectively, and cultured twice at 37°C for 24 and 18 h using orbital agitation at 120 rpm. Then, each broth was transferred to a 50 mL centrifuge tube and centrifuged at 4,001×g at 4°C for 10 min. The supernatant was discarded and cell pellet was completely diluted with 0.85% NaCl to adjust the initial concentration of each bacterial strain from 107 to 108 CFU/mL by measuring the optical density at 600 nm (OD600=0.2).
To quantify the number of bacteria in samples, serial dilutions were performed using 0.85% NaCl. After mixing reacting the bacterial solution with the treatment solution, 1 mL of the solution was transferred to a glass tube containing 9 mL of 0.85% NaCl, followed by serial dilution. The appropriately diluted solution was spread on tryptic soy agar (TSA) and TSA+YE to count Escherichia coli O157:H7 and L. monocytogenes, respectively. The media was incubated at 37°C for 48 h. The number of colonies is expressed as Log CFU/mL or g.
A stock solution (2,500 ppm) of nisin (N5764-5G, Sigma-Aldrich, St. Louis, MO, USA) was prepared by dissolving 125 mg of nisin in 50 mL of 20 mM hydrochloric acid (7647-01-0, Duksan Pure Chem, Ansan, Korea). The stock solution was diluted with deionized (DI) water to prepare 25, 50, and 100 ppm nisin solutions. Then, 2.7 mL of each concentration of nisin solution and 0.3 mL of the bacterial solution were placed in a centrifuge tube. The centrifuge tube was vortexed for 5 s and incubated for 30 min at room temperature (25±2°C). For control, 2.7 mL DI water and 0.3 mL of the bacterial solution were used. In all treatment groups, the mixture of 2.7 mL treatment solution and 0.3 mL bacterial solution was considered as the 100 state. Microbial analysis was performed after dilution with 0.85% NaCl to an appropriate concentration.
The scheme for preparing nisin (Nisin) only, APP only, and combination of APP and nisin (APP+Nisin) is shown in Fig. 1A. Control and Nisin were prepared using a bacterial solution (0.3 mL) with DI water (2.7 mL) and 100 ppm nisin solution (2.7 mL), respectively. For APP, DI water (2.7 mL) was added to the APP-treated bacterial solution (0.3 mL), which was discharged for 5 min. To determine the synergistic effect of APP+Nisin, APP was treated to the bacterial solution (0.3 mL), and 100 ppm nisin solution (2.7 mL) was added. All treated solutions were transferred to a 50 mL centrifuge tube, vortexed for 5 s, and incubated for 30 min at room temperature (25±2°C) to match the reaction time with the Nisin. The solution in the centrifuge tube was regarded as 100 state. Microbial analysis was performed as described above after dilution with 0.85% NaCl to an appropriate concentration.
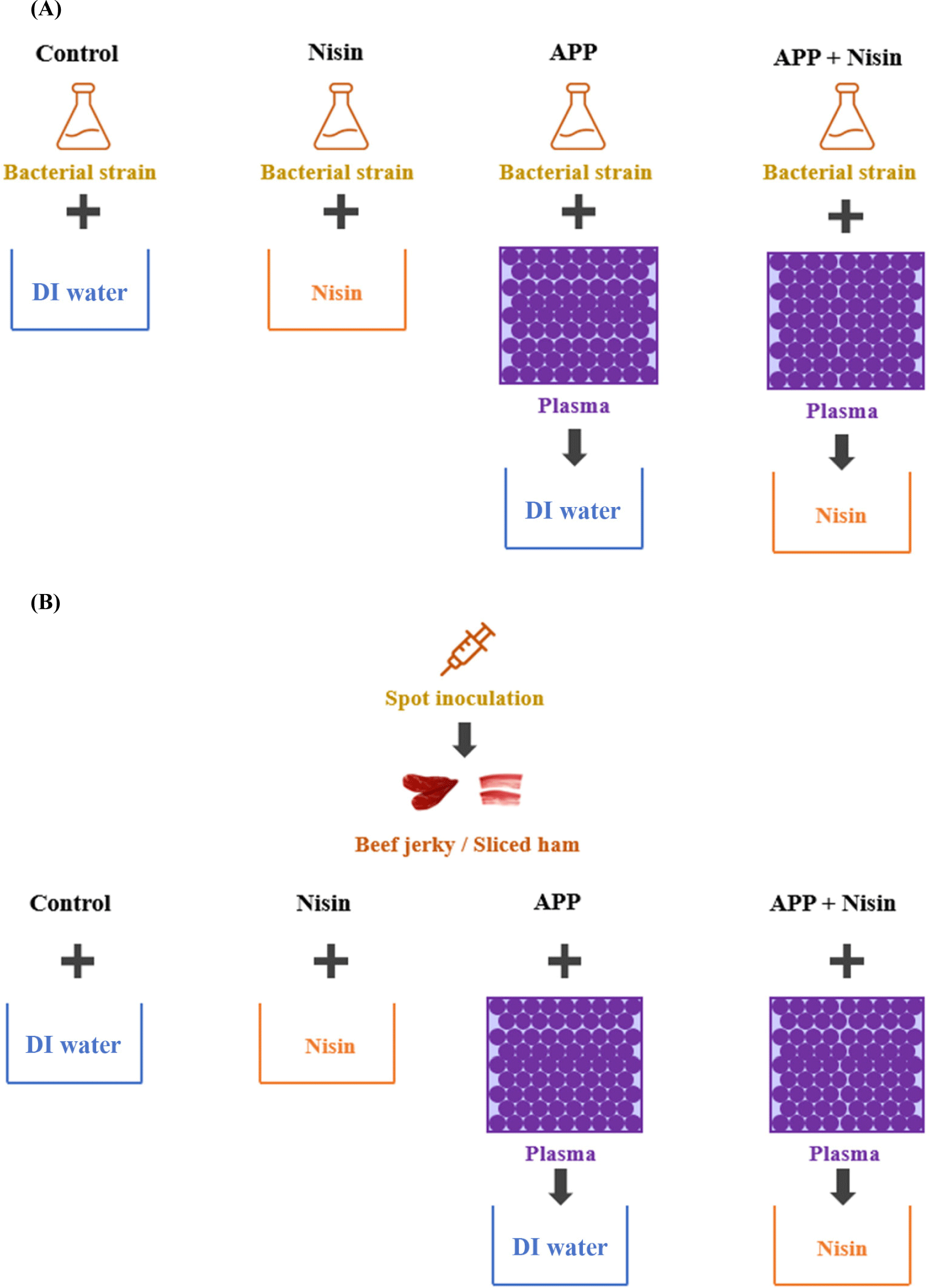
Beef jerky and sliced ham were purchased from a local market (Seoul, Korea). All samples were exposed to UV light for 30 min to remove surface microorganisms prior to cutting the samples and inoculating them with E. coli O157:H7. Beef jerky and sliced ham were cut into 5 g (35×35 mm, n=2) and 3 g (40×40 mm, n=3) pieces, respectively, and 100 μL and 50 μL of E. coli O157:H7 (OD600=0.20), respectively, were spot-inoculated on the surface. The inoculated samples were then dried at room temperature (25±2°C) for 30 min. The scheme of preparing the Nisin, APP, and APP+Nisin for inoculated beef jerky and sliced ham is shown in Fig. 1B. The Nisin involved dipping the samples in nisin solution (100 ppm) for 30 min. For the APP, beef jerky and sliced ham were treated with APP for 5 and 9 min, respectively, and immersed in 45 and 27 mL of DI water, respectively. They were then vortexed for 5 s and allowed to react for 30 min at room temperature (25±2°C). For APP+Nisin, APP was directly applied to the beef jerky and sliced ham for 5 min and 9 min, respectively. Both samples were then dipped in 45 and 27 mL of nisin solution (100 ppm), respectively, vortexed for 5 s, and incubated at room temperature (25±2°C) for 30 min. After completion of the reaction, the beef jerky and sliced ham were transferred to a centrifuge tube containing 0.85% NaCl and vortexed for 2 min to detach the bacterial strain present in the sample, which was considered as the 100 state. Microbial analysis was performed as described above.
All the experiments were performed in triplicate, except for those involving beef jerky, which was replicated. All data were assessed using SAS (version 9.4, SAS Institute, Cary, NC, USA) with statistical significance set at p<0.05. Statistical analysis was conducted using one-way analysis of variance and Tukey’s multiple comparison test.
Results and Discussion
Fig. 2A shows the bactericidal effect of nisin on E. coli O157:H7 and L. monocytogenes. The initial count of E. coli O157:H7 was 7.11 Log CFU/mL. The number of viable E. coli O157:H7 cells at 25, 50, and 100 ppm nisin decreased by 0.04, 0.04, and 0.01 Log CFU/mL, respectively, compared with that at 0 ppm nisin. However, this difference was not statistically significant. This suggests that nisin concentration below 100 ppm has no bactericidal effect on E. coli O157:H7.
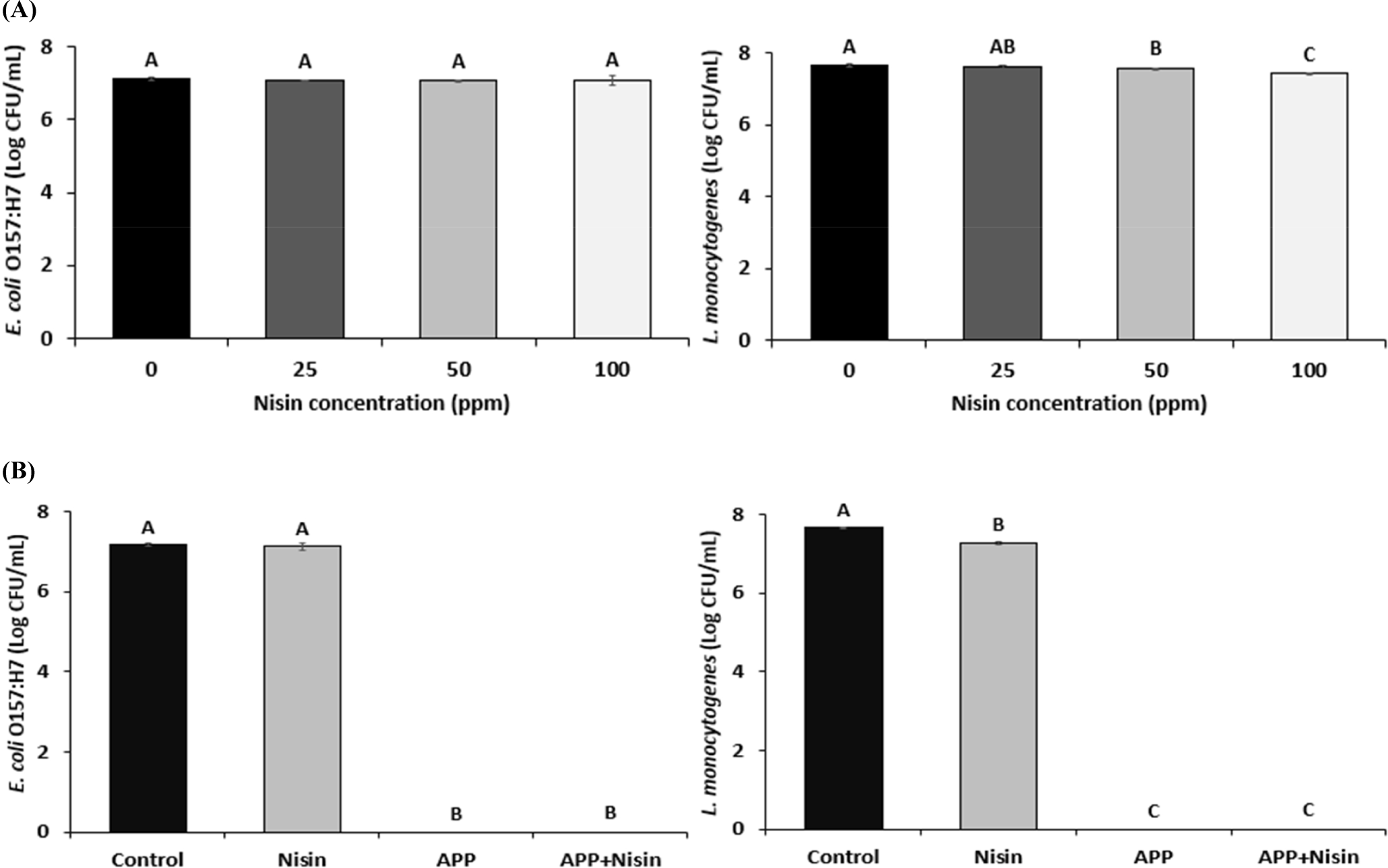
The initial count of L. monocytogenes was 7.66 Log CFU/mL. The number of viable L. monocytogenes cells at 50 and 100 ppm nisin decreased by 0.09, and 0.24 Log CFU/mL, respectively, compared to that at 0 ppm nisin (p<0.05). In addition, the 100 ppm treatment had the highest bactericidal effect among the nisin concentrations tested (p<0.05). When compared the result of the bactericidal effect of nisin at 100 ppm on E. coli O157:H7 and L. monocytogenes, there was a higher reduction rate in L. monocytogenes than in E. coli O157:H7 (3.13% vs. 0.14%). This result confirmed that nisin has a lower bactericidal effect on gram-negative bacteria (E. coli O157:H7) than gam-positive bacteria (L. monocytogenes). Unlike gram-positive bacteria, gram-negative bacteria have an outer membrane composed of phospholipids, proteins, and lipopolysaccharides (Abee et al., 1995). These outer membranes exhibited impermeability to nisin. As nisin is greater than 3 kDa, it is unable to react the cytoplasmic membrane (Abee et al., 1995; Stiles and Hastings, 1991). Therefore, to improve the bactericidal effect of nisin on E. coli O157:H7, the APP+Nisin was evaluated. Based on the bactericidal effect of nisin on the bacterial solution, a concentration of 100 ppm was selected for the APP+Nisin.
The synergistic effect of APP+Nisin against E. coli O157:H7 and L. monocytogenes was evaluated (Fig. 2B). The Nisin exhibited the lowest reduction of E. coli O157:H7 among all treatments (p<0.05), and there was no difference compared to the control. No viable cells were detected in E. coli O157:H7 cells treated with APP. Furthermore, no viable E. coli O157:H7 cells in the APP+Nisin were detected.
Similar to the result for E. coli O157:H7, Nisin exhibited the lowest bactericidal effect among all the treatment groups against L. monocytogenes (p<0.05). No viable L. monocytogenes cells were detected after treatment with either APP or APP+Nisin.
In this study, APP treatment (APP and APP+Nisin) exhibited significantly higher bactericidal effects than Nisin on E. coli O157:H7 and L. monocytogenes (p<0.05). However, the bactericidal effect of APP was too strong to evaluate the synergistic effects of APP and nisin. Unlike the bactericidal effect of Nisin on E. coli O157:H7 and L. monocytogenes, APP exhibited the same bactericidal effect on both pathogens. This is because during APP, the outer membrane of E. coli O157:H7 is damaged by reactive species capable of destroying the DNA, proteins, lipids, and cell membranes of bacterial cells (Kim et al., 2013), resulting in increased permeability of the lipopolysaccharide layer, which would enhance the activity of nisin against bacterial cells. Similarly, according to Yoo et al. (2021), APP of E. coli O157:H7 for 4 min reduced viable cells by 22.31% compared to the control group. Furthermore, E. coli O157:H7 and L. monocytogenes exhibited a 100% reduction in cell viability compared with the control group when treated with APP for 90 s and 10 min, respectively (Yong et al., 2015).
The effect of the APP+Nisin on beef jerky and sliced ham was assessed by inoculation with E. coli O157:H7 (Fig. 3). The Nisin, APP, and APP+Nisin decreased the number of viable cells of E. coli O157:H7 in beef jerky by 0.19, 0.38, and 0.80 Log CFU/mL with a reduction rate of 3.37%, 6.75%, and 14.21%, respectively, compared to the control. Nisin and APP treatment in beef jerky did not exhibit significant differences when compared with the control. In contrast, the APP+Nisin exhibited the highest bactericidal effect among the treatments (p<0.05).
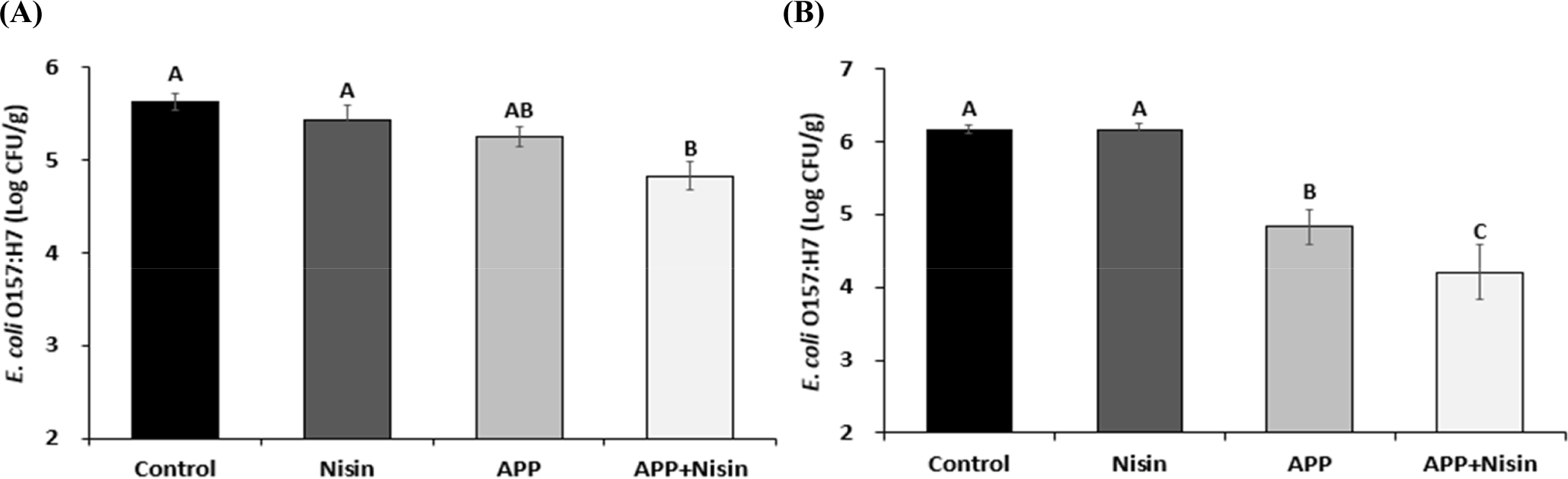
Similarly, Nisin did not decrease the number of E. coli O157:H7 in sliced ham. However, APP and the APP+Nisin induced a decrease in E. coli O157:H7 in sliced ham at 1.34 and 1.96 Log CFU/g with a reduction rate of 21.72% and 31.77%, respectively, compared to the control. Both the APP and the APP+Nisin exhibited significantly higher bactericidal effects than the control and Nisin; in particular, the APP+Nisin exhibited a higher bactericidal effect than the APP (p<0.05).
The APP+Nisin exhibited synergistic bactericidal effects in both beef jerky and sliced ham inoculated with E. coli O157:H7. However, the bactericidal effect of APP and the APP+Nisin on E. coli O157:H7 in meat products was lower than that in bacterial solutions. This is because changes in the physicochemical properties of plasma occurred during APP treatment for organic matters, such as beef jerky and sliced ham. Specifically, the various reactive species generated via APP could react with organic matter, which would reduce the concentration of reactive species capable of responding to bacterial cells. In addition, during the reaction with organic matters, pH may increase and oxidation-reduction potential may decrease, which may also affect the bactericidal effect of APP (Baek et al., 2020a; Xiang et al., 2019). For this reason, the degree of damage that APP can induce on the outer membrane of E. coli O157:H7 may be reduced, which may be the cause of the lower bactericidal effects of meat products compared to bacterial cells.
In addition, the degree of reduction rate of the APP+Nisin against E. coli O157:H7 on beef jerky and sliced ham were 14.21% and 31.77%, respectively. Differences in appearance, such as surface shape and thickness of the samples, can affect the degree of destruction of the bacterial cell membrane by APP. An irregular surface of a sample can create a physical barrier to protect the bacterial strain, which require more energy and time for agents to exert their antibacterial effect (Fernández et al., 2013). In other words, compared to sliced ham, beef jerky with its irregular surface, makes it more difficult for the APP+Nisin to exert its bactericidal effect, compared to that in solutions on E. coli O157:H7. This could be responsible for the lower bactericidal effect of APP on beef jerky.
Conclusion
The APP+Nisin exhibited a synergistic bactericidal effect against E. coli O157:H7 (gram-negative bacteria). In addition, their bactericidal effect was demonstrated in beef jerky and sliced ham inoculated with E. coli O157:H7. This shows that the APP+Nisin has the potential to efficiently control microorganisms on the surfaces of meat and meat products. Further research is needed to establish the optimal conditions for the APP+Nisin applicable for various meat products.