Introduction
Pulsed electric field (PEF) treatment is a non-thermal technology used in food processing, which is characterized by low heat generation, short treatment duration, and low energy consumption (Knorr and Angersbach, 1998). Unlike other electric-based technologies such as ohmic heating, PEF selectively affects the cell membrane during microsecond pulses in a square wave form (Gavahian and Farahnaky, 2018; Kumar et al., 2015). These properties allow for gentle food processing without the adverse effects of heat generation (Barsotti and Cheftel, 1999). Therefore, PEF offers advantages in preserving food characteristics that are generally associated with freshness such as flavor, taste, color, and nutrients.
Various non-thermal technologies have been extensively used in the food industry, including gamma irradiation, plasma, UV-light, pulsed light (PL), and high-pressure processing (HPP). From a bactericidal perspective, gamma irradiation is considered an ideal technology, albeit with some limitations, such as negative public perception and the development of persistent rancidity in high-fat foods (Farkas et al., 2002). Furthermore, the bactericidal effects of plasma, UV-light, and PL are limited to surface-attached microbes (Levy et al., 2012; Soro et al., 2023; Sruthi et al., 2022). HPP is among the most widely commercialized non-thermal technologies and can be applied to both liquid and solid foods, even after packaging (Denoya et al., 2015; Perera et al., 2010). This technology also offers advantages in minimizing heat-induced quality deterioration and has a short treatment duration (Nath et al., 2023). However, HPP has several limitations compared to PEF in terms of productivity because it operates only in batch mode and requires a huge installation space and costs (Balasubramaniam et al., 2015; Sampedro et al., 2014). PEF, on the other hand, can be used in continuous mode and can easily switch to batch mode while retaining the advantages of HPP. Therefore, PEF has emerged as a next-generation non-thermal technology.
PEF can be employed for various purposes, such as controlling texture, facilitating mass transfer, aiding in extraction, and pasteurization, depending on the treatment intensity (Naliyadhara et al., 2022; Zare et al., 2023; Zhang et al., 2023). While most previous research on PEF has focused on liquid and plant-based foods, studies on its application to meat have been relatively limited. The limited application of PEF to meat compared to plant-based products is largely due to the high fat and protein content in meat. Nevertheless, the meat industry continually seeks novel technologies to ensure high-quality meat products. Meat tenderization and safety are key factors influencing meat quality and consumer acceptability. However, the effects of PEF on meat remains controversial due to variations in outcomes depending on the treatment conditions and evaluation methods. Therefore, this review sought to classify findings related to meat tenderization and safety and discuss the discrepancies between these findings.
General Description of Pulsed Electric Field
The tenderizing and bactericidal effects of PEF are generally attributed to cell membrane breakdown induced by electroporation (Zimmermann, 1996). When a cell is exposed to an electric field, micropores are simultaneously created in the phospholipid bilayer of the cell membrane, resulting in increased cell membrane permeability. The cell membrane, which consists of a phospholipid bilayer naturally has a specific transmembrane potential of approximately 10 mV between its inner and outer layers. When the membrane is exposed to an external electric field, the potential induced by the electric field increases until it reaches a critical value. As the potential increases, the membrane reorganizes the charges on both sides, leading to a viscoelastic deformation of the membrane due to the attraction of charges with opposite polarity. This process continues until the critical value is reached. If the external electric field surpasses the critical value of the transmembrane potential, an electro-compression force is generated on both sides of the membrane, initiating the formation of pores.
Detailed information on the PEF system and its components is provided in Fig. 1A. The PEF system typically consists of two main components: the pulse generator and the treatment chamber. The inclusion of other subcomponents depends on whether the system operates in continuous mode (for both liquid and solid samples) or batch mode (single batch). In the continuous mode, a pump or conveyor is necessary to transport the sample to the treatment chamber. Treatment chambers come in three common types: parallel plates, co-axial, and co-linear chambers (Arshad et al., 2020). For the treatment of liquid food in continuous mode, heat exchangers are additionally used to preheat the sample before treatment and cool it down afterward (Toepfl et al., 2014). In contrast, the single batch mode does not require these additional components. The batch chamber is similar to the parallel plates chamber but lacks the flow of the sample. This type of chamber is typically used for solid foods and is suitable for bench-scale applications. PEF treatment for solid foods is conducted in the batch chamber, which is filled with a low-conductive medium such as tap water. Unlike continuous chambers, batch chambers have been modified in various forms (Jeong et al., 2023; Li et al., 2020; Mok et al., 2017). The adjustable chamber illustrated in Fig. 1A was designed to allow for the adjustment of electrode gap (Jeong et al., 2023). This setup can be used to treat samples without the need for a medium and enables direct contact with the chamber without space between the electrode and the sample. While the use of batch chambers is advantageous for tailoring conditions at the bench scale, it is important to note that, in contrast to the continuous mode, treatment in batch mode is more susceptible to raising the sample temperature due to the high electric field strength (Toepfl et al., 2014). Therefore, batch treatment should be carried out at an acceptable level of PEF intensity to minimize heating.
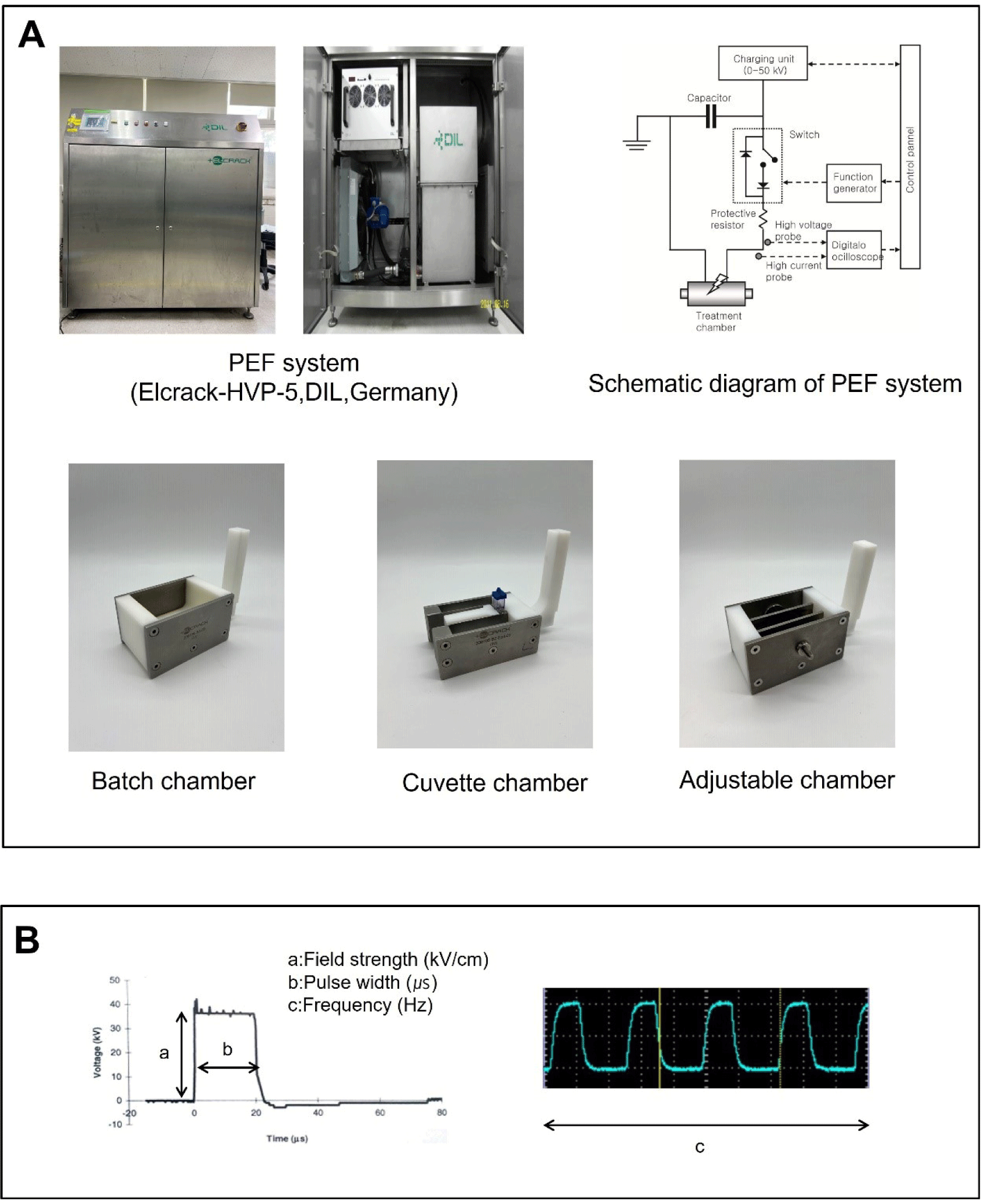
The effect of PEF on tissue is determined by both the properties of the sample and the PEF parameters. From the perspective of suitability for PEF processing, the electrical conductivity of the samples is a crucial factor because it influences temperature increases (Athmaselvi et al., 2014). The parameters in the PEF system are detailed in Fig. 1B. The electric field strength (E, kV/cm) is a primary factor that determines the extent of the PEF effect. The electric field strength is calculated using the following Eq. (1):
A sufficient field strength is necessary to reach the threshold for entering an electroporated state (irreversible state). The required level varies for each sample and depends on the cell size. Solid foods with large cells typically require a relatively lower field strength for electroporation compared to microbes. The pulse width, measured in microseconds, represents the duration during which the high voltage is maintained for each pulse in the form of a square wave. Short pulse widths are beneficial in preventing temperature increases during PEF treatment. The pulse frequency is a factor that determines the treatment time in terms of the number of pulses. These parameters can be expressed as a specific energy (Q, kJ/kg) using the following Eq. (2) (Zhang et al., 1995):
where V is the output voltage (kV), t is the treatment time (number of pulses/frequency), R is the resistance (ohm), and m is the mass of sample (kg). In the case of PEF treatments for solid foods, field strength is commonly used as a variable rather than specific energy, as the pulse width and treatment time are often held at fixed values.
Action of Pulsed Electric Field on the Tissue
The mechanism of electroporation can be theoretically explained; however, the evidence of cell membrane permeability is not entirely clear at the cellular level. This uncertainty arises because, while we can observe the irreversible state of the cell disrupted by PEF, we cannot easily observe the reversible state. At the tissue level, such as in vegetables or meat with a heterogeneous distribution of cell sizes, observing the reversible state can be quite challenging. The effects of PEF on tissues are observed as macroscopic phenomena, unlike at the cellular level, and these phenomena are dependent on both PEF intensity and tissue properties. When tissue is exposed to PEF, some cells within the tissue are damaged by the electric field. The distribution of damaged cells varies depending on the intensity and cell size. In other words, damaged and intact cells coexist heterogeneously. The ratio of damaged cells can be assessed by measuring an electric signal that results from ion release from the cells. This signal change is expressed in terms of electric conductivity (S/m). The PEF treatment selectively affects the cell membrane inside the tissue, leading to changes in ion release. Morphologically, it can be challenging to distinguish between intact and treated tissue. For example, in the microstructure of PEF-treated tissue, the cell wall may appear intact, whereas the cell membrane and organelles may exhibit some degree of damage (Kim et al., 2019). Similarly, in muscle tissue where there is no cell wall, morphological differences can be difficult to discern. This selective effect can result in a more flexible texture, caused by the release of turgor pressure within the cell. Therefore, PEF can soften the texture and improve processing suitability. Furthermore, the damaged cell membrane can create porosity in the tissue, increasing mass transport channels and accelerating mass transfer. Damaged organelles, such as vacuoles, can also facilitate the extraction of bio compounds.
Lebovka et al. (2002) introduced an effective tool for assessing the degree of tissue rupture using conductivity. The authors proposed evaluating the degree of rupture caused by PEF treatment through the cell disintegration index Z (CDI), which compares intact cells with completely ruptured cells. This tool has gained widespread acceptance in evaluating tissue permeability in numerous studies. Similar to the CDI concept, other evaluation tools have been developed to assess relative disintegration of mass transfer, texture, and acoustics. These methods provide a representation of macroscopic change of the tissue rather than focusing on the cellular level.
The underlying mechanisms through which PEF influences microscopic phenomena, as well as at the cellular level, remain unclear. However, recent efforts have been made to substantiate existing hypotheses. Genovese et al. (2021) utilized non-destructive MRI analysis to observe changes in moisture distribution in plant tissues after PEF treatment. Plant tissues exhibit heterogeneous moisture distribution due to variations in cell size. This heterogeneity leads to varying effects of PEF on each cell. Irreversible deformation induced by PEF results in a homogeneous distribution of moisture, which is confirmed through T2 mapping and MRI tomography (Genovese et al., 2023). Some ruptured cells release cytoplasm into intracellular voids, leading to a homogeneous moisture distribution as the cytoplasm fills the voids. This phenomenon alters the osmotic pressure in adjacent cells. This non-destructive analysis significantly enhances our understanding of cell permeabilization and complements conductivity analysis.
Tenderization Effects of Pulsed Electric Field Treatment
Tenderness is a critical factor when evaluating sensory quality, and the primary effect of PEF on meat is its tenderization. The degree of tenderization at a macroscopic scale is typically assessed through mechanical measurements such as shear force or meat hardness. However, various studies utilizing different meat materials, PEF intensities, and sample sizes have produced conflicting results regarding the tenderization effects of PEF. Tables 1 and 2 categorize these studies based on the presence or absence of a tenderization effect following PEF treatment. The studies listed in Table 1 demonstrate that PEF treatment did not lead to a significant tenderization effect. These studies share common characteristics, including the use of commercial PEF systems and direct contact with the electrodes. Interestingly, low PEF intensity did not result in a tenderization effect (Bhat et al., 2019a; Khan et al., 2017). Similarly, studies employing high-intensity PEF treatment, which should be sufficient for cell disruption, did not impact meat tenderness (Arroyo et al., 2015a; Arroyo et al., 2015b; Faridnia et al., 2014; Faridnia et al., 2015; McDonnell et al., 2014; O’Dowd et al., 2013). In contrast, in the case of plant tissue, treatment with an electric field strength of approximately 1.0 kV/cm resulted in increased flexibility and softness in texture (Fauster et al., 2018; Lee et al., 2022). These results may be because the PEF effect is masked when using excessively large sample sizes for treatment and measuring texture at excessively high temperatures.
Meat materials | PEF condition | Sample status | Findings | References |
---|---|---|---|---|
Semimembranosus (beef) | 20 μs, 90 Hz, 0.36 kV/cm 20 μs, 20 Hz, 0.60 kV/cm The treatment time or number of pulses were not provided |
Post-rigor, sample size was trimmed fitted in the batch chamber (13×8×5 cm), direct contact with the electrodes | No significant reduction in shear force | Bhat et al. (2019a) |
Longissimus et lumborum (beef) | 20 μs, 200 Hz, 0.23 kV/cm 20 μs, 200 Hz, 0.68 kV/cm Total treatment time: 30 s |
Post-rigor, sample size was trimmed fitted in the batch chamber (13×8×5 cm), direct contact with the electrodes | No significant reduction in shear force | Khan et al. (2017) |
Semitendinosus (beef) | 20 μs, 50 Hz, 1.40 kV/cm, 1,032 pulses | Post-rigor, sample size was trimmed fitted in a triangular batch chamber (6×4×6 cm), direct contact the electrodes | No significant reduction in shear force | Faridnia et al. (2015) |
Breast meat (turkey) | 20 μs, 10 to 110 Hz, 1.25 to 2.0 kV/cm, 100 to 300 pulses | Post-rigor, sample size was trimmed to 6×2×2 cm, Direct contact with the electrodes | No significant reduction in shear force | Arroyo et al. (2015a) |
Longissimus thoracis et lumborum (beef) | 20 μs, 10 Hz, 1.40 kV/cm, 300 and 600 pulses | Post-rigor, sample size was trimmed to 6×2×2 cm, direct contact with the electrodes | No significant reduction in shear force | Arroyo et al. (2015b) |
Longissimus thoracis (beef) | 20 μs, 1 to 50 Hz, 0.20 to 0.56 kV/cm, 30 to 1,528 pulses | Post-rigor, sample size was trimmed to 10×8×4 cm, direct contact with the electrodes | No significant reduction in shear force | Faridnia et al. (2014) |
Longissimus thoracis et lumborum (pork) | 20 μs, 100 and 200 Hz, 1.20 and 2.30 kV/cm, 150 and 300 pulses | Post-rigor, sample size was trimmed to 6×2×2 cm, direct contact with the electrodes | No significant reduction in hardness and chewiness | McDonnell et al. (2014) |
Semitendinosus (beef) | 20 μs, 152 Hz, 1.10 kV/cm, 152 pulses 20 μs, 200 Hz, 1.50 kV/cm, 200 pulses 20 μs, 65 Hz, 1.90 kV/cm, 250 pulses 20 μs, 5 Hz, 2.80 kV/cm, 300 pulses |
Post-rigor, sample size was trimmed to 6×2×2 cm, direct contact with the electrodes | No significant reduction in shear force | O’Dowd et al. (2013) |
Meat materials | PEF condition | Sample status | Findings | References |
---|---|---|---|---|
Semitendinosus (beef) | 30 μs, 50 Hz, 0.5 to 2.0 kV/cm, 100 pulses | Post-rigor, sample size was trimmed to 2×1×1 cm, direct contact with the electrodes | Significant reduction of shear force, hardness, and chewiness of raw meat | Jeong et al. (2023) |
Transversus thoracis muscle (beef) | 20 μs, 50 Hz, 0.7 kV/cm, 1,600 and 5,200 pulses | Post-rigor, sample was cut into 5 cm width pieces treated in a batch chamber filled with agar pads as medium | Significant reduction of hardness in high-intensity PEF-treated meat after sous-vide cooking | Karki et al. (2023) |
Semitendinosus (beef) | 20 μs, 50 Hz, 1.0 to 2.0 kV/cm, 200 pulses | Post-rigor, sample size was trimmed to 6×3×3 cm, treated in a batch chamber filled with tap water | Significant reduction of shear force and hardness both in raw and sous-vide cooked meat | Jeong et al. (2020) |
Longissimus lumborum and semimembranosus (beef) | Repeated treatment (1×, 2×, 3×) 20 μs, 90 Hz, 1.25 kV/cm Total treatment time: 30s | Pre-rigor, sample size was trimmed fitted in the batch chamber (13×8×5 cm), direct contact with the electrodes | Significant reduction of shear force on the semimembranosus | Bekhit et al. (2016) |
Biceps femoris (beef) | 20 μs, 50 Hz, 1.7 kV/cm | Post-rigor, sample size was trimmed fitted in a triangular batch chamber (6×4×6 cm), direct contact with the electrodes | Significant reduction of shear force at all aging times | Faridnia et al. (2016) |
Longissimus lumborum and semimembranosus (beef) | Repeated treatment (1×, 2×, 3×) 20 μs, 90 Hz, 1.25 kV/cm Treatment time or number of pulses are not described | Post-rigor, sample size was trimmed fitted in the batch chamber (13×8×5 cm), direct contact with the electrodes | Significant reduction of shear force on the Longissimus lumborum | Suwandy et al. (2015c) |
Longissimus lumborum and semimembranosus (beef) | Repeated treatment (1×, 2×, 3×) 20 μs, 90 Hz, 1.25 kV/cm Total treatment time: 30s | Pre-rigor, sample size was trimmed fitted in the batch chamber (13×8×5 cm), direct contact with the electrodes | Significant reduction of shear force at all aging times | Suwandy et al. (2015b) |
Longissimus lumborum and semimembranosus (beef) | Repeated treatment (1×, 2×, 3×) 20 μs, 90 Hz, 0.625 and 1.25 kV/cm | Post-rigor sample size was trimmed fitted in the batch chamber (13×8×5 cm), direct contact with the electrodes | Significant reduction of shear force at all aging times | Suwandy et al. (2015a) |
Longissimus lumborum and semimembranosus (beef) | Repeated treatment (1×, 2×, 3×) 20 μs, 20 to 90 Hz, 0.27 to 0.56 kV/cm 606 to 2,724 pulses | Post-rigor, sample size was trimmed fitted in the batch chamber (13×8×5 cm), direct contact with the electrodes | Significant reduction of shear force at all aging times | Bekhit et al. (2014) |
Large sample sizes require a considerable amount of energy to reach the necessary PEF intensity for tenderization. Additionally, the outcome is influenced by sample properties such as fat composition or connective tissue. Bhat et al. (2019a) and Khan et al. (2017) did not observe a significant reduction in shear force due to insufficient PEF intensity. However, Suwandy et al. (2015c) observed a tenderization effect with a sufficient PEF intensity, even when using samples of the same size as the former studies. A sufficient PEF intensity can ensure that the cell permeability induced by PEF is homogeneous. Generally, the presence or absence of a PEF effect is indirectly validated through changes in electric signals. Sufficient PEF intensity increases the electric conductivity of muscle tissue because damaged cells release intracellular fluid, which is an electrolyte. However, it can be challenging to determine whether a homogeneous PEF effect has been achieved for each sample immediately after PEF treatment, as some previous studies did not provide this information (Arroyo et al., 2015a; Arroyo et al., 2015b; McDonnell et al., 2014). Conversely, in a recent study, Jeong et al. (2023) used smaller sample sizes and clearly observed a positive linear relationship between PEF intensity and electric conductivity. While this relationship can be observed through changes in texture, an increase in conductivity can also occur due to changes in microstructure, regardless of the tenderization effect. According to O’Dowd et al. (2013), PEF treatment reduced the diameter of muscle fiber bundles and increased conductivity. Similar results were reported by Khan et al. (2017).
Most of the studies listed in Tables 1 and 2 heated the meat samples for measuring shear force or texture profiles. While Jeong et al. (2023) assessed the texture of raw beef Semitendinosus muscle, it is important to note that eating raw meat is not a common practice worldwide, except in some East Asian countries. Additionally, the texture of raw meat can vary depending on its temperature. Due to these factors, meat texture is generally evaluated using cooked meat, typically prepared in a water bath at 80°C until the internal temperature reaches approximately 70°C. However, this cooking process can potentially negate the effects of PEF treatment. Connective tissues in meat tend to contract at temperatures over 65°C (Tornberg, 2005), resulting in a tougher texture. Tenderization by PEF may not be observed when the PEF effect is not uniformly distributed throughout the meat. This phenomenon can be confirmed by comparing the studies listed in Tables 1 and 2, as they provide evidence of this effect. Furthermore, studies that have explored the combination of PEF and sous-vide cooking have observed a tenderization effect (Jeong et al., 2020; Karki et al., 2023). According to Jeong et al. (2020), the PEF effect observed in raw beef Semitendinosus muscle was maintained even after sous-vide cooking (60°C, 1 h to 24 h). Karki et al. (2023) conducted the PEF treatment and sous-vide cooking (60°C, 24 h and 36 h) on short ribs. The high-energy PEF-treated (0.7 kV/cm, 5,200 pulses) and longer-cooked (36 h) samples exhibited a significant reduction in tenderness compared to the control (p<0.001).
Several studies have demonstrated that proteolysis is facilitated by PEF treatment, regardless of the mechanical texture measurements. Table 3 summarizes the findings of studies on proteolysis induced by PEF, which were approached from three different perspectives: the level of myofibril structure (Chian et al., 2019; Mungure et al., 2020; O’Dowd et al., 2013), structural proteins (Bhat et al., 2019b; Bhat et al., 2019c; Jeong et al., 2023; Suwandy et al., 2015a; Suwandy et al., 2015b), and myofibrillar protein (MP) extracts (Dong et al., 2020; Wang et al., 2022).
Meat materials | PEF condition | Target protein | Findings | References |
---|---|---|---|---|
Semitendinosus (beef) | 30 μs, 50 Hz, 0.5 to 2.0 kV/cm, 100 pulses | μ-Calpain, troponin-T | μ-Calpain was not reduced regardless of PEF intensity, however, troponin-T was proportionally reduced by increases of PEF intensity. | Jeong et al. (2023) |
Myofibrillar protein (MP) extraction of longissimus lumborum (pork) | 6 μs, 500 Hz, 2.5 to 7.5 kV/cm, 15,000 to 45,000 pulses | MP | Microstructure of MP was deformed, and the hydrophobicity of MP surface was enhanced by PEF treatment. | Wang et al. (2022) |
MP extraction from the pectoralis (chicken) | 600 to 1,000 Hz, 8 to 28 kV/cm, pulse width and number of pulses are not described | MP | The solubility and hydrophobicity of MP were increased, and the dynamic rheological properties were enhanced by PEF treatment. | Dong et al. (2020) |
Longissimus lumborum (deer) | 20 μs, 50 Hz, 2.5 to 10 kV, treatment time or number of pulses are not described | Myofibril | The myofibril was fragmented and ruptured along the z-line by PEF treatment. | Mungure et al. (2020) |
Longissimus thoracis (beef) | 20 μs, 50 Hz, 1.0 and 1.25 kV/cm, 500 and 2,000 pulses | Myosin, actin, collagen, myofibril | Thermal profiles of the myosin, actin, and collagen were not affected by PEF treatment. The myofibrillar structure was ruptured by PEF treatment. |
Chian et al. (2019) |
Longissimus dorsi (deer) | 20 μs, 50 and 90 Hz, 0.2 and 0.5 kV/cm, 1,500 and 2,700 pulses | Calpain, desmin, troponin-T | The calpain activity was slightly increased by PEF treatment. The degradation of desmin and troponin-T was observed in PEF treated sample. |
Bhat et al. (2019c) |
Longissimus dorsi (deer) | 20 μs, 50 and 90 Hz, 0.3125 and 1.25 kV/cm, 1,500 and 2,700 pulses | Meat protein Free amino acids | The digestibility and solubility of meat protein were increased by PEF treatment. The concentration of free amino acids was higher in the PEF treated sample. |
Bhat et al. (2019b) |
Longissimus lumborum and semimembranosus (beef) | 20 to 90 Hz, 0.625 and 1.25 kV/cm, pulse width and number of pulses are not provided | Troponin-T Desmin | The degradation of desmin and troponin-T was increased by PEF treatment. | Suwandy et al. (2015a) |
Longissimus lumborum and semimembranosus (beef) | 20 to 90 Hz, 5 and 10 kV/cm, pulse width and number of pulses are not provided | Troponin-T Desmin | The degradation of desmin and troponin-T was increased by PEF treatment. | Suwandy et al. (2015b) |
Semitendinosus (beef) | 20 μs, 5 to 200 Hz, 1.1 to 2.8 kV/cm, 152 to 300 pulses | Myofibril fragment | The particle size of filtered myofibril fragment was decreased by PEF treatment. | O’Dowd et al. (2013) |
In a study by O’Dowd et al. (2013), myofibrils were extracted through a four-step filtration process, and their particle size was measured. The final filtration fraction, from which connective tissues and debris had been removed, exhibited a smaller particle size in the PEF-treated sample compared to the control and heated samples. PEF treatment also resulted in the rupture and fragmentation of myofibril structure (Chian et al., 2019; Mungure et al., 2020). While these phenomena could be observed under a microscope, further measurements are needed to examine finer changes at the level of structural proteins. The degradation of troponin-T and desmin has been associated with tenderization (Marino et al., 2013; Yates et al., 1983). Calpain activity also plays a crucial role in meat protein degradation (Coria et al., 2018). Jeong et al. (2023) investigated the proteolysis effect of PEF treatment (0.5 to 2.0 kV/cm) and found that PEF treatment did not significantly affect μ-calpain but resulted in a significant decrease in troponin-T. Calpain activity was slightly increased by PEF treatment in deer longissimus lumborum muscle (Bhat et al., 2019c). Likewise, troponin-T and desmin were degraded by PEF on different muscles (Bhat et al., 2019b; Bhat et al., 2019c; Suwandy et al., 2015a; Suwandy et al., 2015b). Dong et al. (2020) and Wang et al. (2022) used MP extracts to observe structural changes in the protein, rather than focusing on a specific protein. Unlike other studies, they treated the samples with high-intensity PEF exceeding 10 kV/cm. Their results indicated that the microstructure of MP was deformed, and the hydrophobicity of the MP surface was enhanced by PEF. These structural changes induced modifications in the rheological properties of MP (Wang et al., 2022).
In addition to meat protein degradation, changes in structure and increased permeability induced by PEF treatment can facilitate the aging process. As evidence of the tenderization effect, PEF treatment may not produce immediate results in the absence of an aging process (O’Dowd et al., 2013), but its effect becomes evident with extended aging periods (Suwandy et al., 2015a; Suwandy et al., 2015b). Therefore, in addition to mechanical texture properties, PEF treatment can facilitate tenderization through proteolysis. Further research should explore approaches in which PEF improves end-product properties as a pre-treatment and investigates whether the PEF effect persists after subsequent processes or enhances the efficiency of the process.
Use of Pulsed Electric Field for the Microbial Inactivation of Meat
The majority of studies on the microbial inactivation effect of PEF have been conducted on liquid food due to the higher intensity of PEF required for electrical breakdown of microorganisms compared to solid food. Smaller cell sizes in microorganisms require higher intensities to induce membrane breakdown. Typically, intensities exceeding 10 kV/cm are required for liquid food. However, meat can begin to cook when such high intensities are applied, which is similar to ohmic heating. Although the microbicidal effect on meat is limited for this reason, various studies on meat safety have consistently been conducted and are summarized in Table 4. The studies in Table 4 examined microbial stability over different storage periods (Aşık-Canbaz et al., 2022; Faridnia et al., 2015), contamination that may occur during PEF treatment (Bhat et al., 2020), and the direct effects of pasteurization (Alahakoon et al., 2019; Haughton et al., 2012; McDonnell et al., 2014; Stachelska et al., 2012).
Meat materials | PEF condition | Target microbes | Findings | References |
---|---|---|---|---|
Chicken breast fillets | 0.2 ms, 4.67, and 7 kV/cm Continuous treatment during storage period |
Total mesophilic aero bacteria (TMAB), total coliform bacteria (TCB) Listeria monocytogenes, Pseudomonas aeruginosa |
The limit for TMAB counts was exceed in PEF-treated samples 2 days later than the control samples. The TCB counts were reduced with 2 Log CFU/g in PEF-treated samples. The counts of L. monocytogenes and P. aeruginosa were maintained in 7 kV/cm PEF treated samples during the storage periods. |
Aşık-Canbaz et al. (2022) |
Beef jerky | 20 μs, 20 Hz, 0.52 kV/cm, 606 pulses | Total plate count, coliform yeast and mold | The microbial contamination by PEF treatment was not observed. | Bhat et al. (2020) |
Deep and superficial pectoral (beef) | 20 μs, 50 Hz, 0.7 and 1.5 kV/cm, 1,030 to 6,400 pulses | Lactic acid bacteria Total aerobic bacteria |
The microbial inactivation effect was not observed after PEF pretreatment. | Alahakoon et al. (2019) |
Semitendinosus (beef) | 20 μs, 50 Hz, 1.4 kV/cm, 1,032 pulses | Total aerobic bacteria | The growth of aerobic bacteria in fresh meat was not different between PEF-treated and untreated meat over the course of 7 days. The growth of aerobic bacteria in frozen-thawed meat increased 2 Log CFU/g in PEF treated meat at 7 days. |
Faridnia et al. (2015) |
Longissimus thoracis et lumborum (pork) | 20 μs, 100 and 200 Hz, 1.2 and 2.3 kV/cm, 150 and 300 pulses | Total viable count (TVC) | The TVC was not affected by PEF treatment. | McDonnell et al. (2014) |
Minced beef meat | 28 to 2,800 MHz, 300 V/m, treatment time: 15 mins, pulse width not described | Yersinia enterocolitica | The Y. enterocolitica inoculated in the meat was reduced under 1 Log CFU/g. | Stachelska et al. (2012) |
Chicken breast | 10 μs, 5 Hz, 3.75 and 15 kV/cm, treatment time: 10 to 30 sec | Campylobacter jejuni Escherichia coli, Salmonella enteridis, Enterobacteriaceae, total viable counts | The microbes were unaffected by PEF treatment. | Haughton et al. (2012) |
Aşık-Canbaz et al. (2022) investigated the impact of moderate-intensity PEF (MIPEF) that is under 10 kV/cm level of electric field strength on the cold storage stability of chicken breast. MIPEF treatment extended the limit for total mesophilic aerobic bacteria counts by up to two more days. Moreover, total coliform bacteria were reduced by 2 Log CFU/g in MIPEF-treated samples. However, the counts of Listeria monocytogenes and Pseudomonas aeruginosa remained equivalent to the control group. Similarly, Faridnia et al. (2015) found that the growth of aerobic bacteria in fresh semitendinosus meat did not differ between PEF-treated and control samples over 7 days. However, in frozen-thawed meat, aerobic bacteria increased by 2 Log CFU/g in PEF-treated samples at 7 days. No microbial contamination occurred due to PEF treatment (Bhat et al., 2020), suggesting that PEF is a suitable technology for meat processing in terms of safety.
Although previous studies have shown stability in terms of storage and contamination following PEF treatment, the lethal effect on microorganisms was either not observed or only minimally apparent. Alahakoon et al. (2019), Haughton et al. (2012), and McDonnell et al. (2014) did not observe significant microbial inactivation effects. Alahakoon et al. (2019) and McDonnell et al. (2014) conducted their studies with insufficient PEF intensity for microbial inactivation. Haughton et al. (2012) inoculated Yersinia enterocolitica in meat (approximately 5–7 Log CFU/g) and applied relatively high PEF voltages (3.75 and 15 kV/cm). Despite applying a sufficient PEF intensity, the reduction in microbial counts was less than 1 Log CFU/g. Since PEF treatment for meat is typically performed at cold temperatures, achieving a direct pasteurization effect is challenging. Even in liquid food, the pasteurization effect diminishes at input temperatures below 25°C. Therefore, the microbial inactivation effect of PEF on meat is relatively lower than that observed in liquid food due to limited available voltage and the requirement for the meat to be in a cold state.
Considerations of Pulsed Electric Field Treatment on the Meat
PEF technology is considered a non-thermal processing method. However, it can result in slight temperature increases during the process. Therefore, most PEF studies on meat have focused on changes in meat quality following PEF treatment. Various factors affecting meat quality, such as color, purge loss, cooking loss, and lipid oxidation, have been the primary areas of investigation. In most studies, meat color was not significantly affected by PEF treatment, and although some studies observed a reduction in CIE a*, these changes were not distinguishable to the naked eye (Jeong et al., 2020; Jeong et al., 2023). Purge loss and cooking loss experienced slight increases due to changes in microstructure induced by PEF treatment (McDonnell et al., 2014; O’Dowd et al., 2013). Additionally, lipid oxidation was found to increase with higher PEF intensities in some studies (Alahakoon et al., 2019; Kantono et al., 2021). These observations highlight the importance of determining an appropriate PEF intensity level that does not compromise meat quality.
Conclusion
PEF technology can have various effects on food depending on the intensity used. In the case of meat, PEF studies have primarily focused on the textural effects of tenderization, although there have been conflicting findings in this regard. These discrepancies can be attributed to the homogeneity of PEF treatment and variations in the conditions of mechanical measurements. However, despite these conflicting results, most studies have seem to agree that PEF can facilitate tenderization through proteolysis. Compared to its effect on liquid foods, the microbial inactivation effect of PEF on meat is relatively limited because the required voltage to achieve a lethal effect is often not reached due to the inherent properties of meat. Additionally, PEF intensity must be carefully modulated to prevent the loss of meat quality, such as lipid oxidation. To overcome these limitations, future research in this area should focus on developing chambers or systems that can more consistently and stably process meat via PEF treatment. Additionally, establishing reference points to ensure the homogeneity of PEF treatment in meat processing will be important to promote the widespread adoption of this technology in the meat industry.