Introduction
Alternative proteins are emerging to address environmental concerns regarding the production of livestock products and resolve global food security issues (Kim et al., 2022c). The major alternative protein materials include plant-based proteins, edible insects, and cell-based cultured meat, all of which are being developed into food technologies (Lee et al., 2020; Lee et al., 2023; Lee et al., 2024). Recently, there has been a rapid growth in the demand for plant-based proteins, with the main consumers being vegetarians; however, additional research is ongoing to mimic the texture, flavor, and nutritional value of meat (Wood and Tavan, 2022). Plant-based proteins can mimic the structure and texture of meat proteins using textured vegetable proteins (TVP), which are produced via extrusion molding by applying heat and pressure (Abilmazhinov et al., 2023). TVP are largely divided into two types, depending on the moisture content at the time of extrusion. Although high-moisture TVP better mimics the fibers of meat, its commercial viability is lower owing to limitations in distribution due to its high moisture content (Baune et al., 2022). In contrast, low-moisture TVP is actively used in industry and research to simulate the texture of meat; however, because it cannot form a cohesive structure on its own, it requires a binder (Kyriakopoulou et al., 2021; Lyu et al., 2023).
Edible insects, another alternative protein source, are attracting considerable attention because of their high protein content, between 40%–70%, in addition to their high nutritional benefits (Pan et al., 2022). Tenebrio molitor larvae (brown mealworms; TM) were the first insects recognized as edible in the European Union and according to previous studies are considered to be the most promising insect food material (Choi et al., 2017a; Gkinali et al., 2022; Lee et al., 2020). The quality characteristics of various foods containing TM, such as bread, biscuits, pasta, and emulsified sausages, have also been studied (Gkinali et al., 2022). Another study was conducted to simulate reconstituted plant-based protein jerky by mixing TVP and TM (Kim et al., 2022b). However, the content, structure, and physicochemical properties of TM proteins can be altered during production and processing (Hong et al., 2020). In addition, there is a lack of in-depth research on the interaction between plant proteins and TM proteins in a combined emulsion system.
Transglutaminase (TG) catalyzes the covalent cross-linking between lysine and glutamine residues in proteins. It is used to form stable structures and improve the physical properties of various protein-based foods (Choi et al., 2017b; Kim et al., 2022a). In particular, TG can improve the gel strength of emulsified meat products by forming a stable protein network (Yong et al., 2020). Insufficient protein-protein interactions can result in a weak protein gel structure that is prone to collapse. In a previous study, TG was used to strengthen the interaction of edible insect proteins in yogurt (Gharibzahedi and Altintas, 2024). The effects of TG on the structural stability and strength of protein-based emulsion-type foods may be influenced by the amino acid composition or structure of the sample (Choi et al., 2016).
Therefore, in this study, the ratio of TM to TVP was varied, and TG was added to the mixture. The physicochemical properties and structural stability of the prepared emulsions were determined to identify the optimal mixing ratio and effect of TG treatment.
Materials and Methods
TVP (Solbar, Ningbo, China) was hydrated at room temperature for 2 h before use. Frozen TM were purchased from a local market and thawed at 4°C for 24 h. Once thawed, the mealworm larvae were then blended (VH7230, Bomann, Jangseong, Korea). Mealworms to TVP were then mixed at ratios of 0:100, 25:75, 50:50, 75:25, and 100:0 to produce a homogenate. To each homogenate, 1%, of the optimal ratio of TG (ACTIVA TG-B, ES Food, Kunpo, Korea) was added. These ratios were determined in a previous experiment. The homogenate with the addition of TG was then reacted at 25°C for 1 h (Table 1). Then TG was inactivated by heating it to 90°C for 30 s, as per the manufacturer guidelines. Consequently, treatments were formulated as follows: T1, Emulsion prepared with proteins of TVP0:TM100; T2, Emulsion prepared with proteins of TVP25:TM75; T3, Emulsion prepared with proteins of TVP50:TM50; T4, Emulsion prepared with proteins of TVP75:TM25; T5, Emulsion prepared with proteins of TVP100:TM0; T6, Emulsion prepared with proteins of TVP0:TM100 reacted by TG; T7, Emulsion prepared with proteins of TVP25:TM75 reacted by TG; T8, Emulsion prepared with proteins of TVP50:TM50 reacted by TG; T9, Emulsion prepared with proteins of TVP75:TM25 reacted by TG; T10, Emulsion prepared with proteins of TVP100:TM0 reacted by TG.
The prepared homogenate was mixed with distilled water at a ratio of 1:3, dissolved at 4°C for 12 h, and then centrifuged at 12,000×g for 30 min. The protein concentration in the supernatant separated after centrifugation was measured using the BCA assay.
Changes in tertiary structure were confirmed by measuring the fluorescence intensity of the proteins. Fluorescence measurements were performed using excitation at 280 nm and fluorescence emission at 310–400 nm. The soluble protein from the homogenate was diluted equally to a concentration of 0.3 mg/mL to be used as a sample.
To utilize this protein mixture as a meat alternative, pork fat was used as the fat source in emulsions stabilized by protein mixtures. An emulsion was prepared by homogenizing TVP and TM mixtures with pork back fat at a ratio of 8:2 (Table 1). Emulsions were filled into conical tubes at 25 g each, centrifuged at 1,000×g for 5 min to remove internal air, and heated at 80°C for 30 min.
Five grams of the emulsion was homogenized with 20 mL of distilled water at 8,000 rpm for 30 s and then measured at 20°C using a pH meter (Accumet Model AB15+, Thermofisher Scientific, Waltham, MA, USA). Colorimetry was performed using a colorimeter (CR-410, Minolta, Osaka, Japan), which was calibrated using a white plate (CIE L* +97.83, CIE a* –0.43, CIE b* +1.98).
The rheological properties of the emulsions were examined for storage and loss modulus in the angular frequency range of 1–100 rad/s using a rheometer (MCR102, Anton Parr, Graz, Austria) and a plate with a diameter of 25 mm. The shear strain was set to 0.1% using an amplitude sweep.
Approximately 30 mg of the emulsion was placed in a differential scanning calorimetry (DSC) sample pan and heated to 25°C–95°C at a rate of 10°C/min using the DSC4000 (PerkinElmer, Waltham, MA, USA). The denaturation point and heat capacity changes during emulsion heating were measured.
The cooking loss of the emulsion was calculated as the rate of sample loss due to heating by comparing the weight of the sample in the conical tube before and after heating. The water holding capacities of the emulsions were measured using the centrifugal force method. One gram of the emulsion was placed in a conical tube containing Whatman paper no. 1 (Whatman, Kent, UK) and centrifuged at 500×g for 10 min. The weights before and after centrifugation were compared to calculate the ratio of separated water. For emulsion stability, 20 g of emulsion was placed in a glass tube divided by wire mesh and heated at 80°C for 30 min. The volumes of water and oil separated from the sample were checked, and the ratio of the separated liquid (v/w) was measured (Shin et al., 2022).
The textural properties of the cooked emulsions were confirmed by a textural property-measuring device (TA-XTplus; Stable Micro System, Godalming, UK) and a probe with a diameter of 40 mm. The sample was prepared to have a diameter and height of 25 mm, with a measurement speed of 5 mm/s, strain of 50%, and trigger force of 5 g (Shin et al., 2022).
Statistical analysis showed a significant difference (p<0.05) through one-way analysis of variance (ANOVA) and Duncan’s multiple range test using SPSS Statistics (version 20.0; IBM, Armonk, NY, USA). All experiments were repeated at least three times, and the results were expressed as the mean and SD.
Results and Discussion
Protein solubility was determined when TVP and TM were treated with TG. As shown in Fig. 1, the mixing ratio under TG treatment significantly affected protein solubility (p<0.05). Protein solubility decreased substantially as TVP was increased. There was no significant difference in protein solubility with the addition of TG, except in the T1 treatment group, in which a large amount of TM was added (p>0.05). It is believed that high-temperature extrusion during the manufacturing process of TVP induces denaturation of the protein, resulting in low solubility, which improves the texture, but may deteriorate the functional properties of the protein (Samard and Ryu, 2019). However, even after the heat-induced denaturation and protein network formation, some proteins still can be solubilized (Li et al., 2013). Meanwhile, treatment with TG may increase the protein particle size by inducing the formation of covalent bonds between amino acids, thereby reducing its solubility (Ahhmed et al., 2007). In that case, samples with low protein solubility due to TG were predicted to have good physical properties.
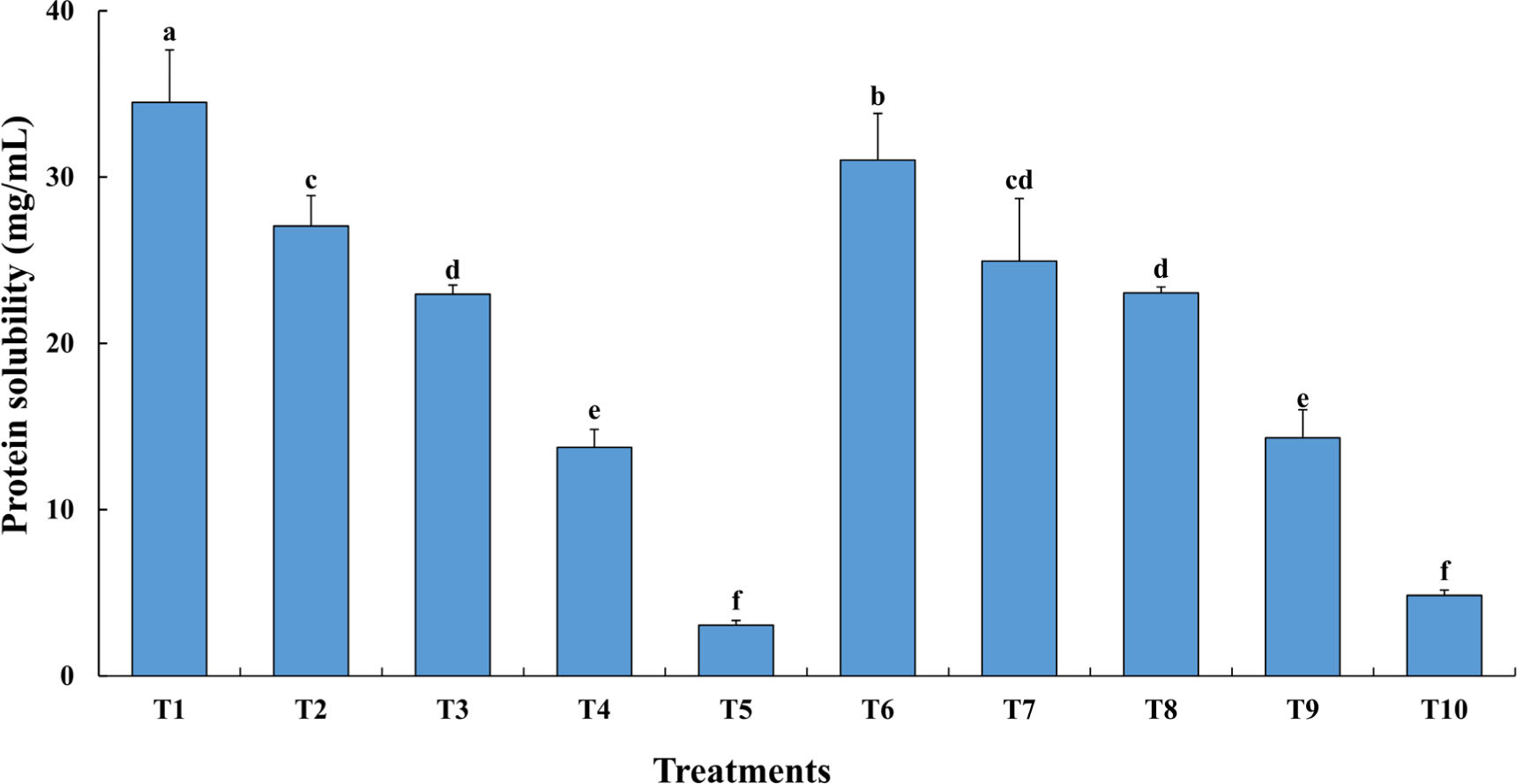
Hydrophobic amino acids such as tryptophan are located inside proteins and change their fluorescence intensity when exposed to protein denaturation (Zhang et al., 2023). Changes in fluorescence intensity due to the addition of TG to the TVP and TM homogenate are shown in Fig. 2. Treatment groups (T1, T2, T3, and T4) without the addition of TG showed little to no change in the fluorescent intensities. However, the increase in fluorescence intensity was observed between T5 (TVP only) and T10 (TVP with TG). In addition, the maximum absorption wavelength of T5 was 350 nm, which shifted to 340 nm due to TG in T10. This indicates that although the amount of protein that can be dissolved in TVP is small, the dissolved proteins are greatly affected by TG. Thus, considerable characteristic changes owing to TG can be expected in the TVP.
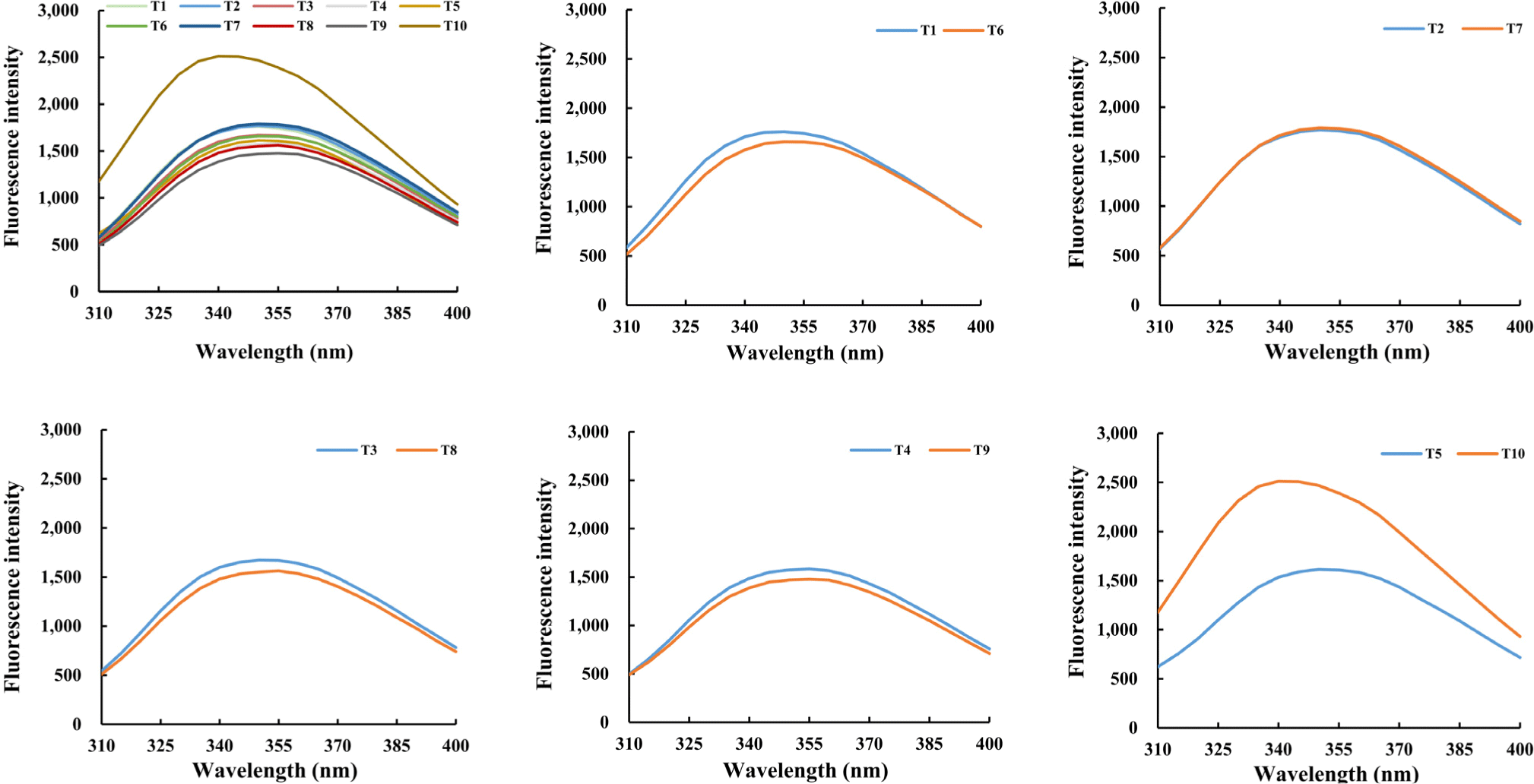
Changes in protein pH influence the type and degree of bonding involved in gel formation by heating, which can affect the physical properties and stability of the gel after heating (Klost et al., 2022). Table 2 presents the results for both pH and color of the emulsion before and after heating. The pH of the emulsion before heating tended to increase as the mixing ratio of TVP increased, thereby, it decreased significantly in TM only treatment (T1), compared to the emulsion prepared with mixed proteins or only TVP (p<0.05). In addition, T10 (TVP with TG) after heat-treatment, exhibited the highest pH value. This finding could be due to the higher pH of TVP (6.98±0.03) compared to the pH of TM (6.35±0.04). Kim et al. (2022b) reported that the pH of restructured jerky analogs with different ratios of TVP and edible insects decreased significantly with an increase in edible insects. Kim et al. (2020) reported that edible insect proteins treated with TG have a significantly higher pH than those treated without TG. In addition, Park et al. (2017) reported that the pH of emulsions containing TG was higher than that of the control without TG. Thereby indicating that TG influences the pH.
CIE L* and CIE b* intensities were highest (p<0.05) in the TVP only treatments (T5 and T10) for both raw and heated emulsions (T5 and T10), indicating no significant difference on the addition of TG. Whereas, CIE a* was the lowest (p<0.05) in the emulsions (T5 and T10) manufactured only with TVP. The color also appeared to be influenced by the ratio of the protein source used rather than the addition of TG. Kim et al. (2022b) showed similar results in the amount of TM added to the restructured jerky analog increased, CIE L* decreased, and CIE a* increased. This is because the unique dark color of TM can negatively affect appearance preference when used as a substitute for meat in processed meat products (Choi et al., 2017a). Therefore, the influence of color can be reduced by using the TVP, and edible insect proteins should be appropriately mixed when used as alternative protein sources.
The mixing ratio of TVP to TM and rheological properties of the emulsion after TG treatment are shown in Fig. 3. Both the frequency-dependent storage modulus (G’) and loss modulus (G’’) increased as the proportion of TVP in the emulsion increased, this was also apparent with the TG treatment. It is known that protein-protein interactions caused by TG treatment can affect the increase in G’ and G’’ (Ruzengwe et al., 2020). Although TVP has a relatively low concentration of dissolved proteins, it is believed that an increase in G’ and G’’ could be caused by significant changes in the protein structure due to TG treatment, as confirmed by the tertiary structure results. The internal structure organized during the high-temperature extrusion process of TVP was also considered to have influenced the improvement in viscoelasticity before heating (Kim et al., 2022b).
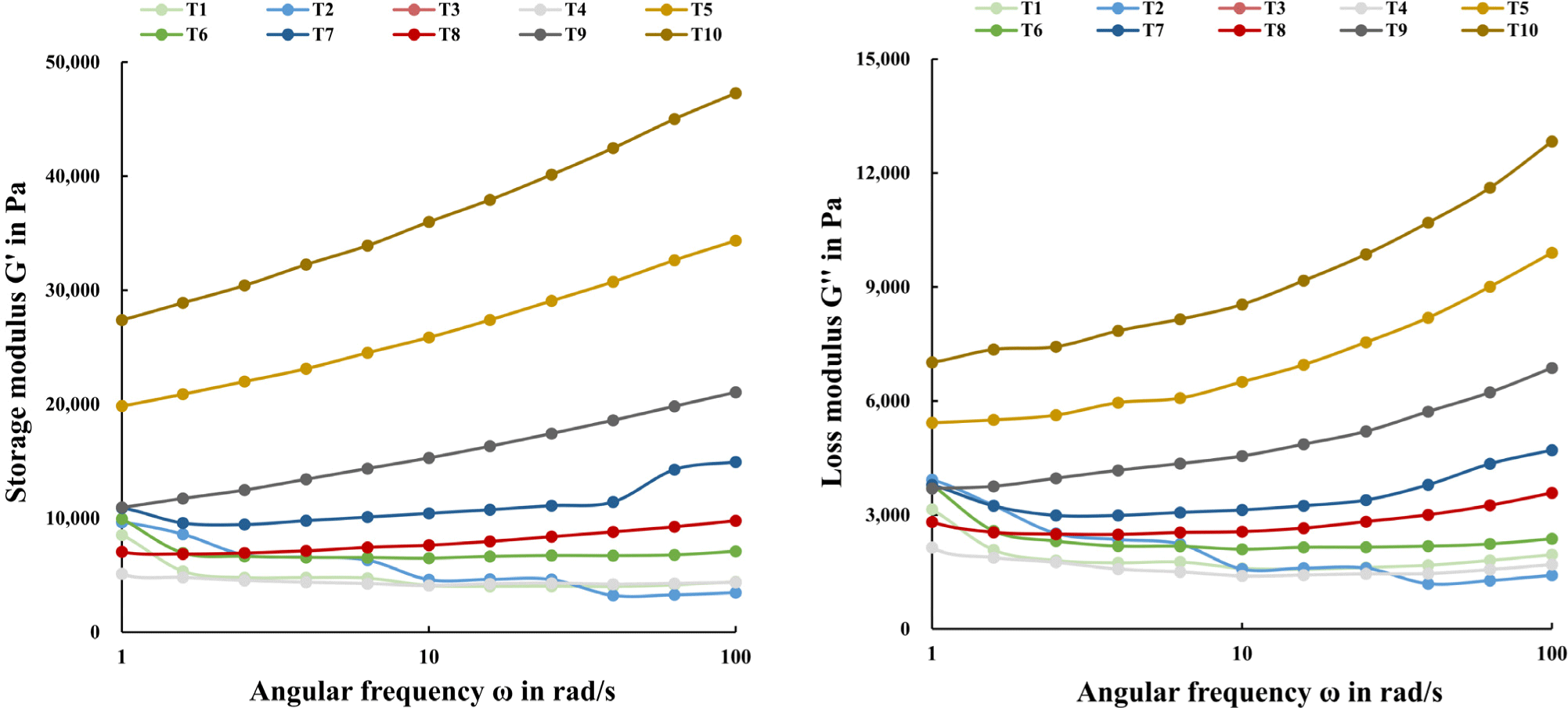
The thermal properties of the emulsion were significantly altered by the mixing ratio of TVP to TM, with or without TG (Table 3). As confirmed by DSC, the protein denaturation peak appeared twice for all treatments. The peak temperatures for both denaturation reactions were high in treatments with a high proportion of TM. T6 showed the highest peak temperature in peak 1 and peak 2 (p<0.05). The thermal capacity for denaturation was highest in T5 and T10, in the first peak, but T6 was the highest in the second peak. A pH close to the isoelectric point of a protein may delay protein unfolding upon heating because the structural stability of the protein is high (Klost et al., 2022). In addition, TVP is a protein that is already denatured during the production process; denaturation is not induced during the heating process of the emulsion, which can lower the peak temperature (Kim et al., 2022b). Because a high denaturation temperature is correlated with the thermal stability of proteins, T6 is considered to have high thermal stability.
Cooking loss, water holding capacity (WHC), and emulsion stability are factors that indicate structural stability through the degree to which the moisture and oil present in the protein structure are separated by heating and external stimulation. The mixing ratio of TVP to TM, with or without TG significantly affected cooking loss, WHC, and emulsion stability characteristics (Table 4). Cooking loss showed a low separation amount of approximately 1% overall but tended to decrease with TG treatment. The WHC in response to centrifugal force showed no significant effect on TG treatments and tended to decrease as the TVP mixing ratio increased. There was no significant difference in the emulsion stability of the total exudate, but the fat exudate increased from 0.67% to 2.00% as the amount of added TVP increased in non TG-added group. This is because the formation of protein structures during heating was mainly caused by the denaturation of the larvae protein rather than by TVP. In addition, similar to the results confirming thermal properties through DSC, T6, which produced an emulsion by treating TM homogenate with TG, showed significantly higher WHC, emulsion stability, and lower cooking loss. Yong et al. (2020) reported that cooking loss and emulsion stability of reduced fat emulsions with konjac gel and TG were lower than those observed for reduced fat samples with konjac gel. Therefore, a stable gel was formed with minimal separation of moisture and fat during the thermal process owing to the high thermal stability of T6.
As shown in Table 5, the textural properties of the gel formed upon heating of the emulsion were significantly affected by the mixing ratio and TG treatment. Hardness was relatively high in the TG-treated group, with significantly higher values at T6, T8, and T10 (p<0.05). There was no significant difference in cohesiveness among the treatment groups (p>0.05); however, gumminess and chewiness were significantly higher at T6 and T8. In the case of T6, as previously confirmed in Tables 3 and 4, the denaturation of TM during the heating process improved the structural stability, and hardness, gumminess, and chewiness are thought to increase. Kim et al. (2022b) reported that the shear force decreased as the amount of TM increased in a restructured jerky analog containing TVP and edible insect protein. This is probably due to the low strength of protein-protein interactions in insect proteins (Bessa et al., 2019). Park et al. (2017) reported that the hardness, gumminess, and chewiness of meat emulsions increased with increasing silkworm pupae levels, and that the incorporation of silkworm pupae and TG into the emulsion significantly improved its hardness, gumminess, and chewiness. Choi et al. (2016) showed that a combination of TG improved and maintained the textural properties of foods by cross-linking with proteins. Thus, it was confirmed that T6 and T8 showed the most improved properties in terms of textural properties, which can be attributed to the thermal stability of TM, high textural properties of the TVP raw material, and promotion of bond formation between proteins by TG treatment.
Conclusion
The mixing ratio of TVP and TM and the quality characteristics of the proteins and emulsions after TG treatment were analyzed. As the TVP content increased, the solubility of the protein decreased; however, a strong TG bond was formed in the dissolved protein. Emulsions with a high proportion of TM treated with TG showed low pH and improved thermal stability, WHC, and emulsion stability. The physical properties of the emulsion after heating were significantly higher in T6, which was an emulsion prepared by the TG treatment of a homogeneous substance composed only of TM. Owing to the excellent physical properties of the TVP raw material and the influence of new bond formation between the two protein sources, T8 also exhibited significantly higher physical property values. Therefore, to improve stability, it is considered most appropriate to treat TM protein with TG, but considering color and physical properties, additional research on the use of a mixture of the two protein sources in equal proportions is necessary.