Introduction
Consumers nowadays are becoming more conscious about their dietary choices, seeking out meat products that provide additional nutritional value and promote overall well-being. Consequently, various functional ingredients primarily derived from plants and animals are being incorporated into processed meat products. Poultry meat is an excellent source of essential proteins, minerals, and vitamins with minimal fat content (de Santana Neto et al., 2021). However, the presence of unsaturated fatty acids in poultry meat may lead to oxidation, resulting in a decline in quality and reduced consumer acceptance (Cartoni Mancinelli et al., 2021). Lipid oxidation leads to the generation of various breakdown components, which may contribute to the unpleasant odors and flavors observed in meat and its products (Domínguez et al., 2019). These compounds possess the potential to induce protein oxidation during processing and storage, resulting in nutrient loss including essential amino acids degradation. Consequently, this leads to reduced protein digestibility, deterioration of color and texture, as well as the formation of potentially harmful substances (Nawaz et al., 2022; Soladoye et al., 2015).
The food industry relies heavily on synthetic antioxidants to prevent lipid and protein oxidation, however, recent research has led to increased efforts in minimizing or substituting the use of synthetic substances in processed goods due to their detrimental impact on human health. In this particular sector, the meat industry has made significant investments in developing natural components that effectively reduce oxidative reactions in meat products, thereby improving their shelf life (Jiang and Xiong, 2016). Chitosan (CH) is extensively used in the food industry for its cationic nature and various beneficial attributes, including metal ion chelation, texture enhancement, and antioxidant and antimicrobial activities. Additionally, it is known for being non-toxic, biodegradable, and non-immunogenic (Harugade et al., 2023). It is extensively employed in the agricultural, poultry, and seafood industries to improve the quality and prolong the shelf life of a wide range of food products. As a result of these advantageous characteristics, CH (in powder or hydrogel form) has been adopted by the comminuted meat industry for the manufacturing of value-added meat products (Han and Bertram, 2017; Qu and Luo, 2020). Due to the absence of a functional group resembling phenolic groups or conjugated structure in its molecule, CH exhibits restricted antioxidant activity. To enhance its antioxidant potential, researchers have explored the grafting technique by incorporating phenolic acids into its composition (Lee et al., 2014; Liu et al., 2014).
Gallic acid (GA) is a naturally occurring phenolic acid that can be found abundantly in various plant sources. Several studies have demonstrated that GA-grafted-CH (GA-g-CH) can serve as an innovative preservative and antioxidant, enhancing the physicochemical characteristics of CH while maintaining food quality (Lan et al., 2023; Yang et al., 2023a). Although numerous studies have been conducted on the synthesis of CH conjugates with phenolic acids, their practical applications remain limited. CH-based conjugates are primarily used as food coating or packaging materials (Lan et al., 2022b; Yang et al., 2022; Zhang et al., 2022), there has been limited research conducted on exploring the potential use of CH grafting with phenolic acids as food ingredients.
The objective of this study was to investigate the impact of GA-g-CH addition on microorganism growth, oxidation stability, water holding capacity (WHC), water migration, and color deterioration in refrigerated chicken patties. The sensory attributes were also analyzed to explore the potential influence of GA-g-CH on the overall quality of chicken patties. The findings may potentially contribute to the development of a novel ingredient for meat preservation and expand the uses of modified CH in the food industry.
Materials and Methods
CH, obtained from Sinopharm Chemical Reagent (Shanghai, China), has a molecular weight of 200 kDa and a degree of deacetylation between 85% and 90%. Gallic acid, 2,4-dinitrophenylhydrazine (DNPH) and bovine serum albumin (BSA) were procured from Sigma-Aldrich Chemical (St. Louis, MO, USA). The media used for the microbiological analysis of samples were obtained from Shanghai Yuanye Bio-Technology (Shanghai, China). The remaining reagents utilized in this study were commercially sourced and of analytical grade.
The GA-g-CH was synthesized using the H2O2/ascorbic acid redox system, following the method described in our previous publication (Zhang et al., 2022). The reaction was conducted for 24 hours at a temperature of 25°C with a GA to CH ratio of 1:1. The Folin-Ciocalteu method was used to determine the graft ratio of GA, which was found to be 141.37±0.84 g of GA equivalents per kg of TA-g-CH (g GAE/kg copolymer).
The fresh chicken breast muscle and pork back fat were obtained from a local meat-processing commercial center within a day after the animals were slaughtered. The samples were promptly chilled and transported to the laboratory for further analysis. The chicken breast muscle was prepared by removing any visible fat and connective tissues. Afterwards, the breast and fat were ground separately for 30 s each using a meat mincer (Ganyun Food Machinery, Ganjiang, China). Four different patty treatments were prepared, which consisted of a control patty (CON) and patties containing 1% (w/w) CH, CH+GA (CH:GA=6:1), and GA-g-CH respectively. For each treatment, a mixture of chicken breast and pork back fat in an 85:15 ratio was combined with additional components, including 15% ice water, 2% sodium chloride, and 1% spices mix (all measured based on the weight of the meat). The meat mixtures were ground for 3 min utilizing the meat mincer. Subsequently, the minced chicken (70 g) was shaped into patties using a round mold with a diameter of 76 mm and a height of 12 mm. The patties were individually packaged in pre-sterilized polystyrene trays and sealed using polyvinyl chloride film with oxygen and moisture permeability properties. The refrigerated storage of packaged patties was conducted at 4°C, utilizing a fridge equipped with fluorescent lamps to replicate the typical retail presentation observed in supermarkets. Fifteen patties were prepared for each treatment group and sampled at intervals of 0, 3, 6, 9, 12, and 15 days.
The assessment of microbial spoilage was conducted using the total viable count (TVC), Pseudomonas spp., and lactic acid bacteria (LAB) as indicators. A 10-g portion of patty was thoroughly mixed with 90 mL of sterile normal saline solution containing 0.9% (w/v) sodium chloride. The suspensions obtained were diluted in sterile normal saline at a ratio of 1:10 for subsequent bacteriological analysis. The TVC was assessed by enumerating the colony-forming units on Plate Count Agar following a 48-hour incubation period at 37°C. Pseudomonas spp. counts were quantified by employing Glutamat Starch Phenol Red agar (30°C for 48 h). The quantification of LAB was performed by employing deMan, Rogosa, and Sharpe (30°C for 72 h). All measurements were reported as Log10 CFU/g and conducted in duplicate.
The thiobarbituric acid-reactive substances (TBARS) values were measured according to the method proposed by Badhani et al. (2015). Briefly, a 5.0 g meat sample was diced and mixed with 50 mL of trichloroacetic acid solution (10% w/v) using a homogenizer. After undergoing filtration, the slurries were then mixed with an equal volume of a solution containing 0.02 M 2-thiobarbituric acid. After being incubated at 100°C for 40 min, the mixture was cooled using tap water. The UV-2600 UV–Vis spectrophotometer was employed to measure the absorbance at 532 nm in order to determine the color intensity of the resulting solution. The concentrations of TBARS were determined by utilizing a calibration curve with 1,1,3,3-tetraethoxypropane as the reference standard. The results were presented in terms of mg malondialdehyde (MDA)/kg meat.
The assessment of protein oxidation in chicken patties involved quantifying the overall level of carbonyl compounds present. This measurement was conducted following the derivatization process using DNPH (Ordaz-Rodríguez et al., 2023). The protein concentration was determined by evaluating the absorbance at 280 nm, employing a standard curve established with BSA. The quantification of carbonyl compounds was represented as nmol/mg protein, utilizing the molar extinction coefficient value for hydrazones (21.0 nM−1 cm−1) at an absorbance wavelength of 370 nm.
The pH variations were evaluated by thoroughly mixing a 10 g patty sample with 100 mL of purified water using a homogenizer operating at 13,000 rpm for 10 seconds. The resulting mixture was then filtered through Whatman No. 1 filter paper to obtain a transparent filtrate. Subsequently, the pH of this filtrate was measured using a digital pH meter following the method outlined by Elhadi et al. (2017), after calibration with pH 7.0 and 4.0 buffer solutions.
The WHC determination was performed using a modified version of the method suggested by Szmańko et al. (2021). The hydration process involved using a homogenizer to mix 10 g of patty with 20 mL of distilled water. Subsequently, centrifugation was conducted at a speed of 1,200×g for 15 min, followed by the removal of the liquid portion above. The determination of the WHC of the sample was conducted utilizing the subsequent Eq. (1):
The mobility and distribution of water within chicken patties were measured using the approach described by Zhang et al. (2023). T2 relaxation times and the corresponding proportions of peak areas were recorded using an LF-NMR analyzer (Shanghai Niumag Analytical Instrument, Shanghai, China) which operated at a magnetic intensity of 0.47 T. Briefly, the samples (approximately 1.5 g) were enclosed using polyethylene films and positioned within a test tube with a diameter of 15 mm for examination. The Carr-Purcell-Meiboom-Gill sequence was employed to assess the transverse relaxation time (T2) of specimens. The program was configured with a sampling frequency of 100 kHz, a scanning period of 2,000 ms, a half echo duration of 150 μs. Data was collected by conducting 8 repetitions of scanning, resulting in a total of 10,000 echoes. The relaxation times were analyzed using the CONTIN algorithm following the normalization of the initial data.
To assess the color variations on the surface of the sample, a portable colorimeter (X-Rite Color I5, X-Rite, Grand Rapids, MI, USA) with an illuminant D65, 10°C standard observer, and 8 mm aperture size was used. Prior to measurement, the device underwent calibration using a reference plate with values set at CIE L*=94.0, CIE a*=0.315, and CIE b*=0.323. The average values of CIE L*, CIE a*, and CIE b* were calculated by taking triplicate measurements from various regions on each patty sample.
The color, odor, and overall acceptance of chicken patties were assessed on each sampling day. The descriptive scale ranged from 1 to 5 points, with 1 indicating the lowest level of acceptability (highly unacceptable) and 5 representing the highest level of acceptability (extremely acceptable). The sensory evaluation panel consisted of ten individuals who had received training to familiarize themselves with the attributes being evaluated and were affiliated with the Department of Food Science at Henan University of Science and Technology (HAUSTIRB 2023 0615). The sensory assessment was conducted in separate chambers with carefully regulated lighting, temperature, and humidity conditions. Based on the shelf-life standards, a rejection would occur if the average sensory ratings were less than 3 (Giménez et al., 2012).
To assess the effects of gallic acid-grafted CH on the quality of refrigerated chicken patties, three sets (replicates) of samples were prepared individually. All measurements were conducted at least three times. The results were reported as the mean values±SE. The General Linear Models procedure provided in Statistix 8.1 software was utilized for the statistical analysis. A two-way factorial analysis of variance (ANOVA) was conducted to analyze the data on quality measurements. To assess differences among mean values, Tukey’s test was employed with a significance level set at p<0.05.
Results and Discussion
The changes in the TVC throughout the refrigerated storage period are depicted in Fig. 1A. The initial TVC (Log CFU/g) of the chicken patties ranged from 2.70 to 2.73 Log CFU/g, suggesting that the samples prepared in this study exhibited excellent quality. Throughout the refrigerated storage period, the different treatment groups exhibited varying degrees of increase in TVC levels. After 3 days, the TVCs of the CON, CH, and CH+GA groups exhibited a significantly greater increase compared to the GA-g-CH groups (p<0.05), as depicted in Fig. 1A. On days 9, 9, and 12 respectively, the TVCs for CON, CH, and CH+GA exceeded the permissible threshold of 7.0 Log CFU/g. By the end of storage, the GA-g-CH treatment demonstrated significantly higher efficacy in inhibiting microbial growth compared to both the CH and CH+GA treatments (p<0.05). Furthermore, the TVC of GA-g-CH treatment consistently remained below the acceptable limit of 7.0 Log CFU/g throughout the entire 15-day period. Hence, the application of GA-g-CH led to a noticeable increase in the microbial shelf life by 3 to 6 days when compared to the control group or other treatments. The findings clearly indicate that the application of GA-g-CH treatment exhibited significantly enhanced efficacy in suppressing microbial proliferation in chicken patties during storage compared to either treatment alone, resulting in an increased preservation period for the chicken patties.
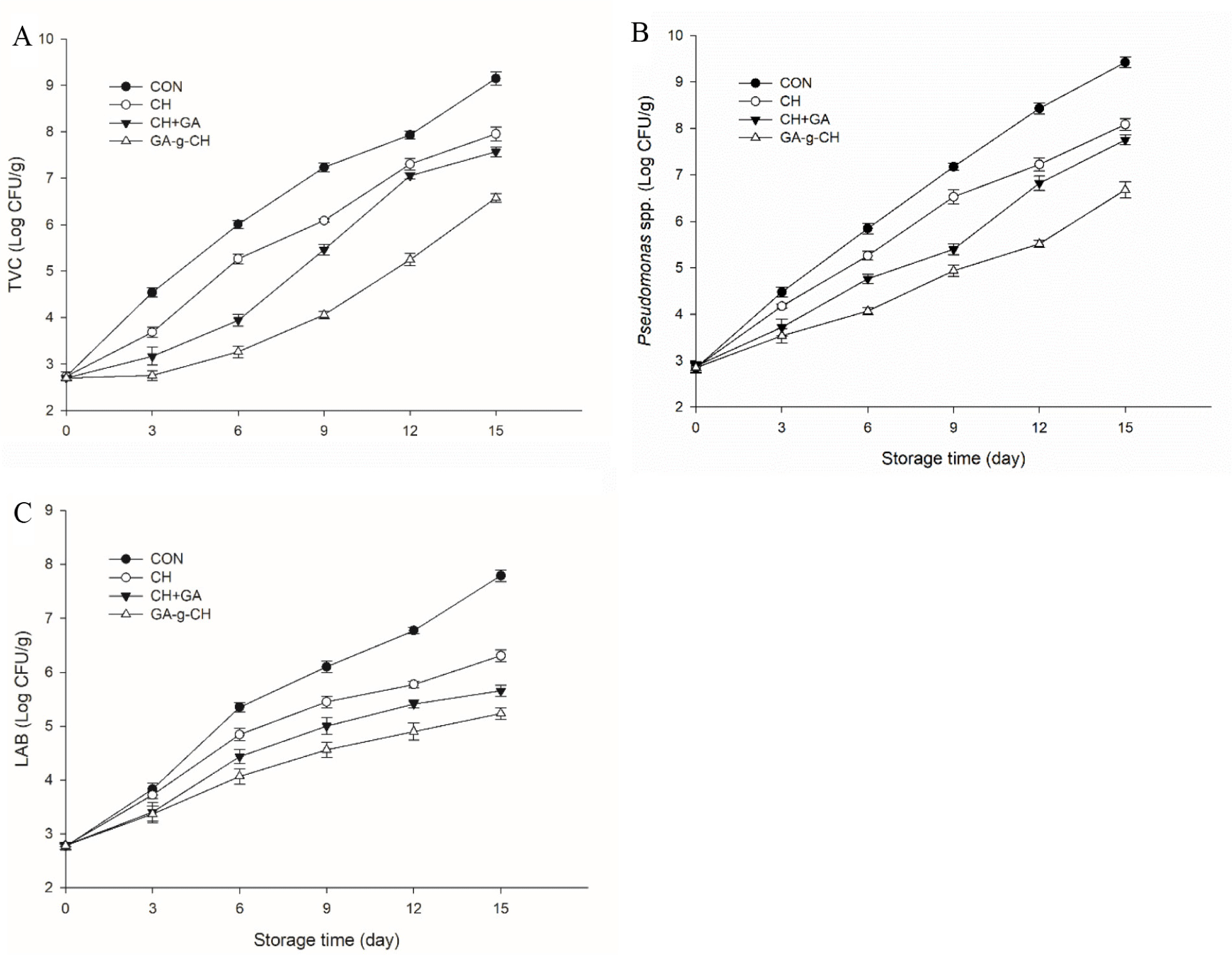
The variation in the Pseudomonas spp. of the refrigerated chicken patties is depicted in Fig. 1B and exhibits a similar trend to that of the variations in the TVC across all samples. The initial TPC for the chicken patties ranged from 2.84 to 2.88 Log CFU/g. At the end of the storage period, the Pseudomonas spp. values for the CON, CH, CH+GA, and GA-g-CH samples were measured as 9.42, 8.08, 7.75, and 6.68 Log CFU/g, respectively. In comparison to other treatments, the incorporation of GA-g-CH exhibited a remarkable inhibitory effect on the growth of Pseudomonas spp. (p<0.05).
The levels of LAB exhibited a noticeable increase over the course of storage, as illustrated in Fig. 1C. The LAB counts initially observed in the chicken patties ranged from 2.77 to 2.79 Log CFU/g. At the end of the storage period, the LAB counts were recorded as 7.78 Log CFU/g for the CON sample, 6.30 Log CFU/g for CH sample, 5.65 Log CFU/g for CH+GA sample, and 5.22 Log CFU/g for GA-g-CH sample. Compared to other treatments, the incorporation of GA-g-CH significantly suppressed the proliferation of LAB in chicken patties (p<0.05).
Gallic acid exhibited antimicrobial properties against various spoilage bacteria commonly found in meat (Del Valle et al., 2018). The antimicrobial properties of CH have been extensively demonstrated, primarily attributed to the interaction between positively charged CH and the negatively charged cell envelope of microorganisms, leading to the disruption of intracellular constituents (Yan et al., 2021). In this research, the addition of GA-g-CH exhibited superior efficacy in inhibiting microbial growth compared to the application of CH+GA during refrigerated storage of chicken patties. The macromolecular graft copolymers exhibit enhanced hydrophobicity due to the interplay between the unoccupied hydroxyl groups in gallic acid and the available amino groups present in CH (Zheng et al., 2018). Hence, there is a higher probability for GA-g-CH to interact with hydrophobic cellular constituents, leading to an increase in membrane permeability. Furthermore, the grafting of natural polyphenols onto CH can enhance the antibacterial activity of the grafted products. Similarly, Liu et al. (2020) demonstrated that the grafting of gallic acid onto CH resulted in improved antimicrobial efficacy in sea bass. According to Zheng et al. (2018), the growth of spoilage bacteria in chilled meat was found to be more effectively inhibited by a coating containing CH grafted with gallic acid compared to a coating containing a mixture of gallic acid and CH.
Lipid oxidation results in the development of unpleasant rancid odors and potentially harmful substances, which can lead to a decline in product quality in general (Domínguez et al., 2019). The susceptibility of meat to oxidation is commonly assessed by measuring TBARS levels. Fig. 2 illustrates the variations in TBARS values of chicken patties under different treatments. The TBARS measurement for the control sample initially recorded as 0.35 mg MDA/kg meat showed a significant increase (p<0.05) during storage and eventually reached to 2.16 mg MDA/kg meat by the end of storage. Compared to the control group, the different treatments resulted in increased protection against oxidation (p<0.05) of the chicken patties throughout storage. No significant differences were observed among the treatment groups (p>0.05) during the initial three-day storage period. At the end of the storage period, it was observed that the control group exhibited significantly higher levels of TBARS (p<0.05). Moreover, the GA-g-CH treatment showed lower TBARS value as the storage time extended (p<0.05). The results indicated that the GA-g-CH treatment effectively reduced TBARS formation in refrigerated chicken patties.
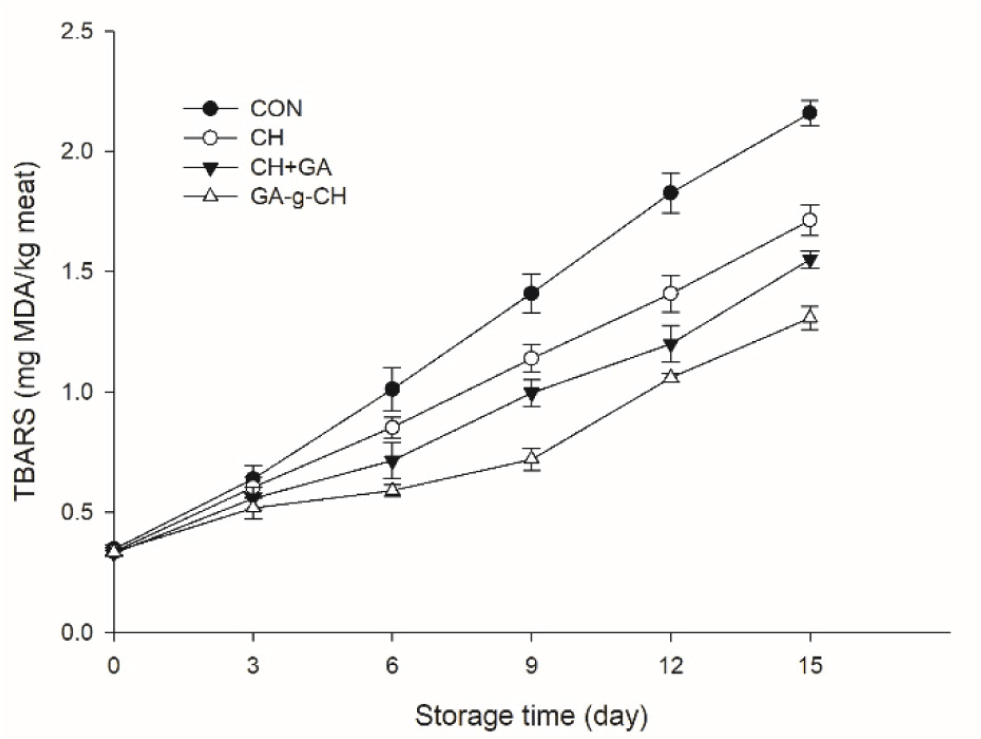
CH has the potential to inhibit lipid oxidation through its ability to scavenge hydroxyl radicals and chelate ferrous ions (Verma and Quraishi, 2021; Yang et al., 2010). Incorporating CH+GA resulted in significantly lower TBARS values compared to the samples incorporated with CH (p<0.05). This can be attributed to the potent antioxidant characteristics of GA, which not only effectively stabilize or eliminate free radicals but also interrupt the progression of oxidation chain reactions (Badhani et al., 2015). The reduction in lipid oxidation levels observed in patty samples incorporated with GA-g-CH can be attributed to either the synergistic impact of GA-modified CH or the controlled liberation characteristic exhibited by GA-g-CH. The results obtained from this investigation align with the research conducted by Yang et al. (2022) and Yang et al. (2023b), as well as Zhang et al. (2022). These studies have highlighted the collaborative impact of CH and polyphenols in retarding oxidation of lipids in meat-based products.
Carbonyl groups are the primary chemical products of protein oxidation, resulting from the conversion of specific amino acid residues into carbonyl compounds (Yang et al., 2022). Measuring the levels of carbonyls provides valuable information about the extent of protein damage caused by oxidative stress during storage of meat products (de Santana Neto et al., 2021). High levels of carbonyls indicate increased protein oxidation, which can lead to changes in texture, flavor, and nutritional quality. Throughout the storage period, there was a noticeable increase in the levels of carbonyl compounds, indicating a deterioration in chicken patty quality (Fig. 3). No statistically significant differences were observed among the groups during the initial 3-day period (p>0.05). The carbonyl content in control chicken patties increased by 10.15 nmol/mg protein at the end of storage, whereas for chicken patties incorporated with CH, CH+GA, and GA-g-CH, the increase was observed to be 7.72, 6.51, and 5.07 nmol/mg protein respectively. Furthermore, it is noteworthy that the carbonyl content in the CH+GA and GA-g-CH treatment groups exhibited significantly lower values compared to the CH treatment group during the same storage period (p<0.05). However, the treatment with GA-g-CH showed a significant decrease in carbonyl group value compared to the treatment with CH+GA at the end of storage time (p<0.05). A comparable trend was noted in terms of lipid oxidation (as depicted in Fig. 3). This is attributed to the strong correlation between lipid oxidation and protein oxidation, both of which are initiated by reactive oxygen species (Domínguez et al., 2022). Therefore, it has been suggested that the oxidation reaction between lipids and proteins can influence each other mutually, resulting in further oxidative processes from their interaction (Geng et al., 2023).
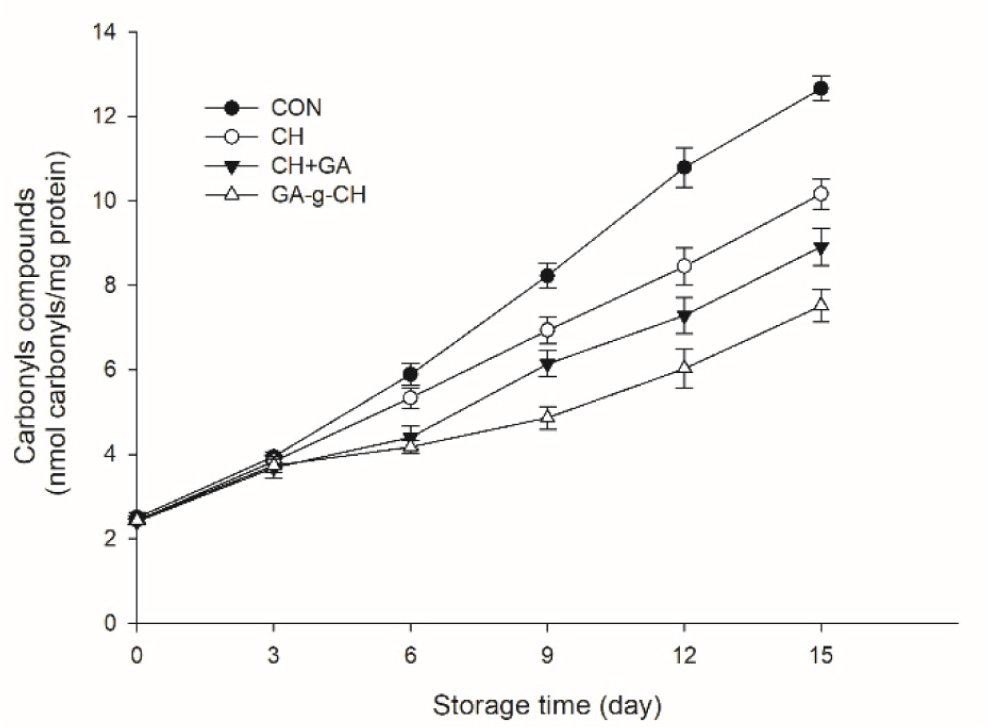
The findings further demonstrated that the incorporation of GA into CH through grafting (GA-g-CH) exhibited superior effectiveness (p<0.05) in inhibiting lipid and protein oxidation compared to the mere combination of GA and CH (GA+CH). The grafting of polyphenols onto CH has been demonstrated to significantly increase the quantity of hydroxyl groups, thereby enhancing the antioxidant activity of CH (Zhang et al., 2022). Moreover, the conjugation system demonstrated the ability to regulate the release of antioxidants, effectively enhancing its efficacy in meat preservation (Wu et al., 2016).
The pH value is commonly used to assess the freshness duration of meat and its products. The pH variations of control and treated chicken patties throughout the storage period are depicted in Fig. 4. The initial pH values of CON, CH, CH+GA, and GA-g-CH were 6.18, 6.20, 6.16, and 6.19 respectively, which exhibited a remarkable increase (p<0.05) to 7.61, 7.29, 7.24, and 6.42 at the end of storage. The rise in pH typically associated with the presence of alkaline autolyzed compounds formed during cellular breakdown and the buildup of bacterial byproducts from protein degradation and microbial proliferation (Fan et al., 2009). No significant differences in pH levels were found between the CH and CH+GA groups (p>0.05), both of which demonstrated antimicrobial properties by effectively preventing an increase in pH levels in refrigerated chicken patties. The pH stability of chicken patties with GA-g-CH was due to its remarkable antimicrobial properties, which inhibited microbial growth and prevented substrate decomposition.
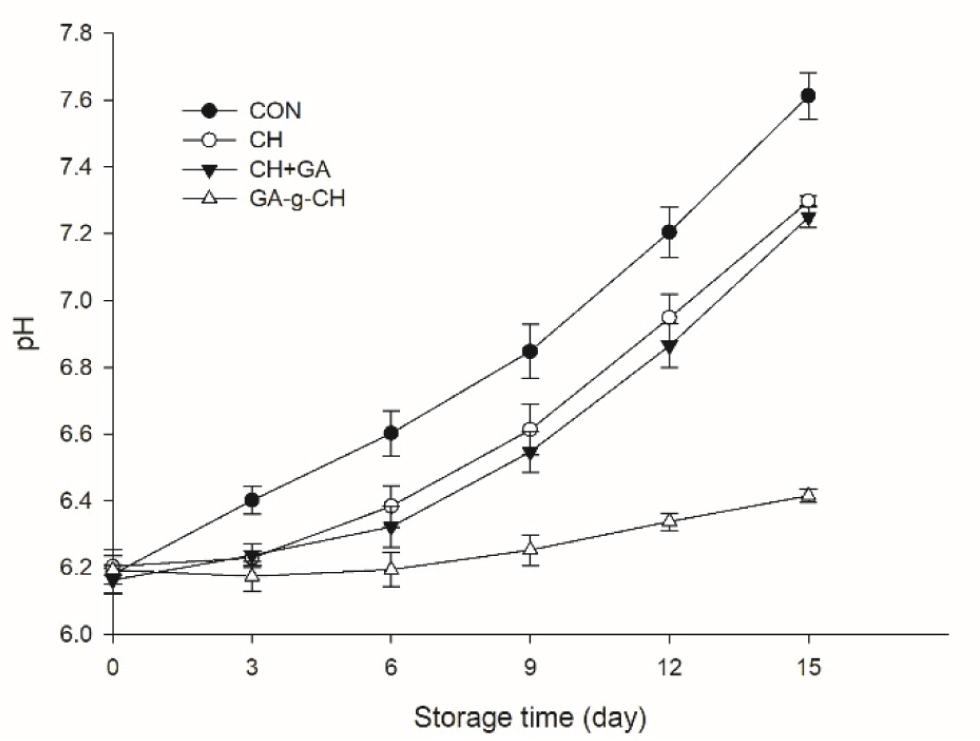
Examining WHC in meat products is essential because moisture plays a vital role in determining their tenderness, yields, and overall flavor (Xu et al., 2023). As depicted in Fig. 5, a decline in the WHC was observed across all samples during the storage duration. After 3 days, the control group exhibited a significantly greater reduction in WHC value (p<0.05) compared to the treated samples. This was primarily attributed to the CH’s ability to absorb and retain moisture from its surrounding environment, thereby slowing down the decrease in WHC (Aranaz et al., 2018). In addition, the incorporation of CH, CH+GA, and GA-g-CH in chicken patties resulted in reduced microbiological degradation, thereby enhancing their WHC. It was observed that the decrease in WHC was slower in the GA-g-CH group compared to both the CH and CH+GA groups. However, there was no significant difference between the CH and CH+GA groups (p>0.05). These findings indicate that incorporating graft copolymer of CH effectively enhances its solubility and dispersion in chicken patties, thus effectively providing protection against moisture loss.
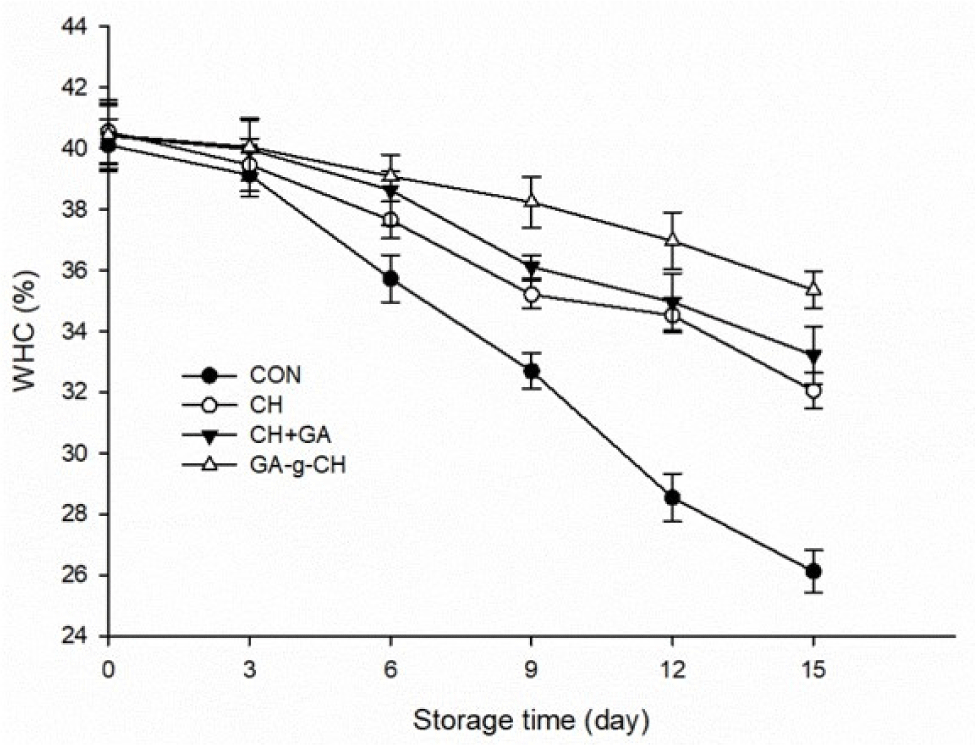
LF-NMR is commonly utilized for assessing the moisture changes in meat during storage or processing. The distribution and percentage of relaxation time (T2) of chicken patties during cold storage are illustrated in Fig. 6 and Table 1. By employing multiple exponential models of relaxation decays, three distinct types of water were identified: bound water (1 ms<T2b<10 ms), immobile water (10 ms<T21<100 ms), and free water (100 ms<T22<1,000 ms; Zhang et al., 2023). The proportions of bound water, immobilized water, and free water are denoted as P2b, P21, and P22, respectively.
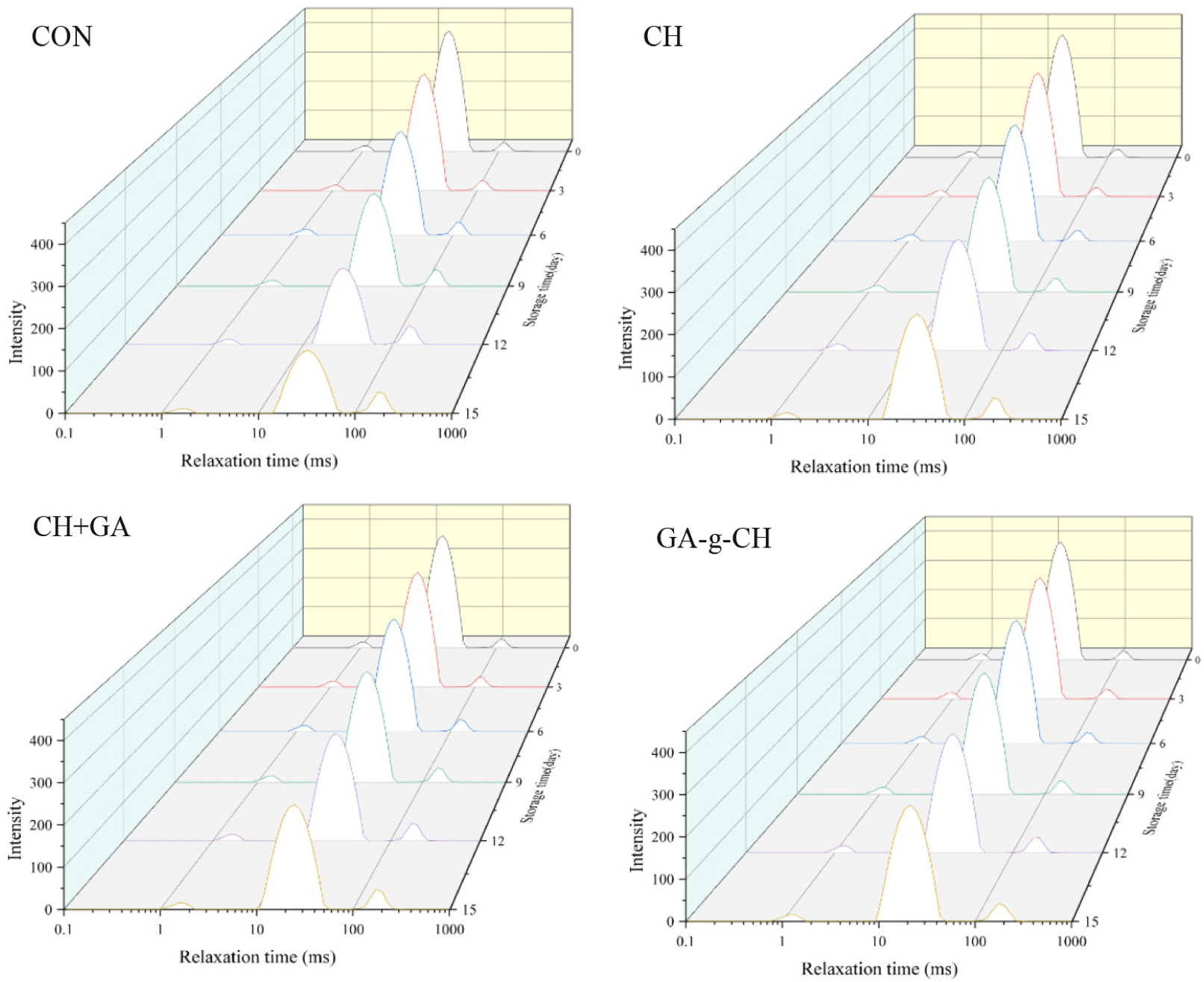
As depicted in Fig. 6, the relaxation times of the T21 and T22 peaks exhibited a noticeable increase as the duration of storage increased (p<0.05). However, the incorporation of CH, CH+GA, and GA-g-CH in chicken patties resulted in a significant reduction (p<0.05) in T21 relaxation times in comparison to the control sample. The T2 relaxation time in the transverse direction is influenced by bonding strength and proton mobility. A prolonged transverse relaxation time indicates a greater degree of molecular mobility and enhanced moisture diffusion (Shao et al., 2016).
The immobilized water (T21) is confined to the extramyofibrillar matrix, which constitutes the primary form of moisture present in chicken patties. On the other hand, the presence of free water (T22) can be observed in the interstitial spaces between myofibrillar structures, but it tends to be lost due to external environmental factors (Zhang et al., 2017). Hence, the rise in relaxation times of T21 and T22 in the chicken patties indicated the occurrence of structural damage to muscle tissue caused by either bacteria or enzymes, leading to a significant expansion of space within and between myofibrils. The findings suggest that the addition of CH and its grafting polymer has the potential to mitigate protein denaturation and hinder the movement of water molecules by inhibiting microbial growth and exhibiting antioxidant properties (Lan et al., 2022a).
P2 reflects the influence of refrigerated storage and additives on the distribution and movement of moisture within chicken patties. As the storage time progresses, the changes in water migration become increasingly apparent. There was no significant change in P2b during the early storage time (3 days; p>0.05). After 6 days of storage in the CON group, there was a noticeable transfer of water. The P2b and P21 exhibited a significant decrease (p<0.05), while the P22 showed a significant increase (p<0.05; Table 1). This implies that there was an increase in the migration of water from tightly bound and immobile states to a more free state during the storage period. The structural integrity of meat muscle fibers was compromised during the storage period, causing the migration of immobilized water from within filaments, muscle fibers, and muscle cell membranes to free water, ultimately leading to a loss of moisture (Zhang et al., 2023).
The P21 of the sample incorporated with CH+GA and GA-g-CH was significantly higher than that of the CON and CH group at 9, 12, and 15 days of storage (p<0.05), while P22 showed a significant decrease (p<0.05). Significant differences were observed between CH+GA and GA-g-CH in P21 and P22 on the 15th day, while no significant differences were found in P2b (p<0.05). The results align with the research conducted by Lan et al. (2022a), which indicated that CH graft copolymer could effectively prevent quality degradation and minimize water migration in fish flesh during cold storage. The findings aligned with the WHC determination (Fig. 5).
The color variation served as a crucial parameter in evaluating the quality of meat products, exerting a direct impact on consumer acceptance. The CIE L* value gradually increased, while the CIE a* and CIE b* values progressively declined in chicken patties during a 15-day refrigeration period for all tested samples (Table 2). The observed elevations in the CIE L* values of the control patties throughout the storage duration could potentially be attributed to the dispersed reflections of light caused by lipid oxidation.
The incorporation of CH, CH+GA, and GA-g-CH resulted in a noticeable reduction in the CIE L* value towards the end of storage (p<0.05). However, there was no significant disparity observed among these three groups. These findings suggest that treatments containing CH can effectively maintain CIE L* and delay the onset of unappealing color compared to the control group. Previous studies have demonstrated that the incorporation of CH into pork effectively prevents an increase in CIE L* value during refrigerated storage (Hu et al., 2015; Siripatrawan and Noipha, 2012).
The presence of myoglobin affects the CIE a* of meat (Lindahl et al., 2001). Generally, higher CIE a* values indicate a lower degree of meat oxidation. The CIE a* values of the samples exhibited a gradual decline during storage, with variations in the rate of decrease observed across different treatments. The control sample exhibited lower CIE a* values compared to the treated samples, and this difference was found to be statistically significant (p<0.05). The considerable reductions in the CIE a* values of the control samples could potentially be ascribed to the metmyoglobin formation subsequent to myoglobin oxidation. The color enhancement of meat by CH is due to its ability to bind water and lipids, resulting in an increased CIE a* value (Fernando et al., 2024). The application of CH+GA and GA-g-CH treatments in this study effectively delayed metmyoglobin-induced discoloration in chicken patties, resulting in higher CIE a* values. This delay in metmyoglobin formation can be associated with the strong antioxidant characteristics of gallic acid. According to Cao et al. (2019), the addition of gallic acid to the CH coating was observed to enhance its antioxidant capacity and improve color stability, specifically a more stable red hue, in fresh pork samples.
Similarly, throughout the refrigeration storage period, the CIE b* value decreased for all experimental groups due to oxidative processes in chicken patties. Furthermore, the incorporation of CH, CH+GA, and GA-g-CH significantly increased the CIE b* value of the samples due to their antioxidant properties. The GA-g-CH treatment exhibited a significantly higher CIE b* value (p<0.05) compared to the other treatments during storage, possibly due to the antioxidative properties of CH and phenolic acid copolymers. This finding aligns with the research conducted by Zheng et al. (2018), which demonstrated that the application of gallic acid-grafted CH effectively maintained the color stability of refrigerated pork.
The sensory scores of all samples showed a noticeable decline throughout the period of refrigerated storage, as indicated in Table 3. The color scores of chicken patties showed a noticeable reduction (p<0.05), which was observed to have a correlation with the CIE a* value in this study. The sensory score for color decreases as the CIE a* value decreases and the CIE b* value increases, suggesting that higher instrumental CIE a* values contribute to enhancing the appeal of chicken patties. The oxidation process affecting both pigments and lipids is the primary cause for the gradual decrease in color scores observed in refrigerated meat products (Talukder et al., 2020). The color scores of the control samples were found to be unacceptable on day 9, whereas the treated samples exhibited a noticeably slower decline in color scores throughout the entire 15-day storage period. This suggests that the treatment groups effectively prolonged the preservation of color in the samples. Furthermore, the results indicated that chicken patties incorporated with CH+GA and GA-g-CH exhibited significantly higher color scores compared to those incorporated with CH, suggesting that these two treatments effectively prolonged the retention of red color in chicken patties.
Off-odor in meat is mainly caused by the existence of oxidation products from lipids and spoilage from microorganisms (Zhou et al., 2022). All treatments led to a delay in the development of unpleasant odor. Samples incorporated with CH showed unfavorable odor evaluations after 12 days. There were no significant differences in odor scores of chicken patties incorporated with CH+GA and GA-g-CH during the initial storage period of 6 days. However, as the storage time increased, samples incorporated with GA-g-CH exhibited significantly higher odor scores compared to those incorporated with CH+GA (p<0.05). The samples incorporated with CH+GA exhibited a significantly lower odor score of 3.37 on day 12, which was closely correlated with elevated TBARS levels and increased microbial counts.
The overall acceptability score of the control samples on day 9 fell below satisfactory levels, while the samples incorporated with CH obtained a lower acceptability score of 3.05 on day 12. The overall acceptance score of chicken patties incorporated with CH+GA exhibited a decrease in score to 3.15 after 15 days of storage. However, the decline in acceptability was significantly delayed by the GA-g-CH treatment. By the end of the storage, a higher score of 3.53 was observed for the GA-g-CH treated patties. This could be associated with the superior antimicrobial and antioxidant characteristics exhibited by GA-g-CH in comparison to CH+GA. Hence, in comparison to other treatments, the addition of GA-g-CH resulted in an extension of 3–6 days in the shelf life of chicken patties.
Conclusion
The grafting of GA onto CH was found to enhance the antioxidant and antimicrobial capacity of CH. This research evaluates the effectiveness of incorporating GA-g-CH in preserving the freshness of refrigerated chicken patties. Compared to CH and CH+GA, the incorporation of GA-g-CH demonstrated superior effectiveness in preventing microbial spoilage, lipid and protein oxidation, as well as water migration. As a result, GA-g-CH addition effectively preserved the sensory characteristics of chicken patties during storage and extended their shelf life by 3–6 days. The findings suggest that GA-g-CH exhibits promising potential as an effective additive for the preservation of ground chicken products.