Introduction
The use of biomaterials in food packaging has recently attracted attention because of health and environmental concerns. The rising cost of petroleum products and the increasing environmental damage caused by their use as packaging materials has led to a growing interest in alternative materials that can be used instead of petroleum-derived materials (Salimiraad et al., 2022). Biopolymers are one of the popular alternative materials (Rahman et al., 2021). These biodegradable packaging materials can include proteins, lipids, polysaccharides and their combinations (Zabihollahi et al., 2020). Chitosan (CH) is a commonly used carbohydrate-derived biodegradable polymer (Wang et al., 2021). CH, obtained from deacetylated chitin, is a polysaccharide composed of glucosamine and N-acetylglucosamine copolymer (Jiang et al., 2022). The fact that CH is non-toxic, biodegradable, biocompatible (Ambaye et al., 2022; Bento et al., 2020), low-cost, sustainable, and renewable makes it increasingly researched (Silva et al., 2021). Also, CH shows broad-spectrum antimicrobial activity (bacteria, yeast, and molds; Yu et al., 2021). Moreover, CH is commonly used in food preservation and packaging owing to its good film-forming properties. An important way to develop many features (mechanical, barrier, antimicrobial, and antioxidant, etc.) of CH-based films and coatings is to combine CH with different organic or inorganic materials (Qu and Luo, 2021).
Despite the development of new food processing techniques that improve food quality, microbial contamination remains a major safety concern for all foods (Nwabor et al., 2020). With modern food processing techniques, a targeted reduction in germs can be achieved during production. However, post-production contamination is still the main factor in microbial food deterioration. Therefore, edible antimicrobial packaging (films or coatings) is of great importance for preventing microbial spoilage (Kumar et al., 2020).
Edible packaging is a biopolymer that can be produced and developed from renewable materials (such as polysaccharides and proteins; Hoque et al., 2021). However, biopolymeric films show poor mechanical and barrier properties. Nanocomposites are a new material class and possess at least one nanoscale size. They have become important in the development of the physico-mechanical and thermal features of these films (Hosseini et al., 2022; Nath et al., 2022). Titanium dioxide (TiO2) nanoparticles are interesting substances utilized in the production of nanocomposite films and coatings (He et al., 2016). TiO2 is a semiconductor metal oxide that is considered a promising material because of its chemical stability, low toxicity, and low cost (Jovanović et al., 2015; Lin et al., 2015). The addition of phenolic substances to these environmentally friendly materials can provide new properties for packaging materials (Heydari-Majd et al., 2019; Nath et al., 2022).
One of the most efficient, naturally water-soluble phenolic co-pigments, rosmarinic acid (Zhao et al., 2021), is an ester of caffeic acid and 3,4-dihydroxy phenyl lactic acid (Petersen and Simmonds, 2003) and lipids. It is of interest to the food industry as a natural antioxidant and antibacterial agent (Marchev et al., 2021). It has been reported that rosmarinic acid can be used mainly in the manufacture of nanocomposite packaging (Sani et al., 2017).
Rainbow trout (Oncorhynchus mykiss) plays an important role in human nutrition because of its high protein and omega-3 fatty acid contents. Therefore, it is highly valued in the market and often sold as fresh fillets (Hosseini et al., 2022). However, rainbow trout and other seafood products are also susceptible to microbiological and chemical deterioration due to their high water activity and pH values, free amino acids, and polyunsaturated fatty acids (Volpe et al., 2015).
The aim of this study was to determine the effects of adding nanoparticles and phenolic substances to edible coatings on the quality of cold-stored rainbow trout under a modified atmosphere. For this purpose, rainbow trout fillets were applied five treatments [control, CH, chitosan+TiO2 (CHT), and chitosan+TiO2+rosmarinic acid (CHTRA)]. After these treatments, rainbow trout fillets were packaged under a modified (40% CO2+30% O2+30% N2) atmosphere. During cold storage (4°C) for 18 days, the samples were subjected to physico-chemical [(pH, thiobarbituric acid reactive substances (TBARS), total volatile basic nitrogen (TVB-N), instrumental color parameters (CIE L*, CIE a*, and CIE b*)], and microbiological analyses [total aerobic mesophilic bacteria (TMAB) and psychrotrophic bacteria].
Materials and Methods
CH with a deacetylation degree of 75%–85% and medium molecular weight (Sigma-Aldrich, St. Louis, MO, USA), glycerol (85%, Merck, Darmstadt, Germany), acetic acid (100%, Merck), and rosmarinic acid (HPLC-grade, purity≥98%; Sigma-Aldrich) were utilized for the preparation of edible coatings. Titanium dioxide nanoparticles (TiO2) with a 30–50 nm particle size and high purity (99%) were acquired from a nanotechnological products company (Nanografi, Ankara, Turkey). The packaging material employed was Polyamide/Polyethylene (PA/PE) bags (15×25 cm, 3- seal bags GB 70) obtained from Südpack Verpackungen (Ochsenhausen, Germany) company, with an oxygen permeability of 40 cm3/m2/day.atm. at 23°C, nitrogen permeability of 24 cm3/m2/day.atm. at 23°C, carbon dioxide permeability of 145 cm3/m2/day.atm. at 23°C, and water vapor permeability of <3 g/m2/day.atm. at 23°C.
Preparation of CH coating and nanocomposite coatings to apply the composite and nanocomposite coating materials, 168 skinned fillets were obtained from 84 rainbow trout (O. mykiss), weighing an average of 350–400 g, sourced from the Atatürk University Faculty of Fisheries Application Center (Erzurum, Türkiye).
In this study, three different coating materials were prepared: CH (2% CH suspension; CH), CH (2% CH suspension) with TiO2 nanoparticles (1.5%; CHT), and CH (2% CH suspension) with TiO2 nanoparticles (1.5%) and 5 ppm rosmarinic acid (CHTRA).
The CH solution was prepared using a modified solvent-casting method described by Kanmani and Rhim (2014) and Nowzari et al. (2013). A 2% CH solution was prepared by dissolving CH in 1% (v/v) acetic acid. To ensure complete dissolution, the solution was stirred for 24 h at 50°C using a magnetic stirrer (MSH-20, Daihan Scientific, Wonju, Korea). After 24 h of mixing, 1% (v/v) glycerol was added as a plasticizer and the solution was stirred for an additional 6 h. TiO2 nanoparticles (10 mg/L) and/or rosmarinic acid (0.005 mg/mL) previously prepared using ultrasound (BANDELIN electronic, Berlin, Germany) were slowly added to the coating solutions. The mixture was stirred at 24,000 rpm with a homogenizer (Ultra-Turrax T25, IKA T25, IKA-Werke, Staufen, Germany) during the addition process, followed by an additional 20 min of stirring. The nanocomposite coating solutions, containing titanium dioxide and rosmarinic acid underwent 20 min of ultrasound treatment and 10 min of UV irradiation (Lin et al., 2015).
The coating process for trout fillets was conducted using the immersion method. Trout fillets were immersed in the prepared coating solutions for 1 min to facilitate the coating process. Subsequently, the coated trout fillets were dried at 4°C for 12 h. The dried samples were then packaged using a packaging machine (Multivac A 300/16, Multivac, Wolfertschwenden, Germany) under modified atmospheric conditions (40% CO2+30% O2+30% N2). The packaged samples were stored at 4±1°C for 18 days. Trout fillets without any coating process but with direct modified atmosphere packaging (MAP) were designated as the control group.
For microbiological analysis, 25 g of sample was homogenized with 225 mL of sterile physiological saline solution (0.85% NaCl) in a stomacher (Lab Stomacher Blander 400-BA 7021, Seward Medical, Newport Gwent, UK) for 1 min. Serial dilutions were prepared from this homogenate and microbiological analyzes were carried out on days 0, 3, 6, 9, 12, 15, and 18 of the storage period. For enumeration of aerobic for TMAB, Plate Count Agar (PCA, Merck) was used. The plates were incubated at 30°C for 48 h (Baumgart et al., 1993); For psychrotrophic bacteria, PCA (Plate Count Agar, Merck) was used and the plates were incubated at 10°C for 7 days (Vanderzant and Splittstoesser, 1992); Enterobacteriaceae were determined on Violet Red Bile Dextrose (VRBD, Merck), the plates were incubated at 30°C for 2 days under anaerobic condition using (Anaerocult, Merck); colonies larger than 1 mm were counted (Baumgart et al., 1993). All microbiological analyses were carried out using the surface spread plate method. The results were expressed as Log CFU/g.
To determine the pH value, 10 g sample was homogenized in 100 mL of distilled water. The mixture was homogenized using an Ultra-Turrax (IKA T25, IKA-Werke, Staufen, Germany) for 1 min. The pH values were calibrated using appropriate buffer solutions (pH 4.00 and pH 7.00) and measured using a pH meter (Schott, Lab Star pH, Thermo Fisher Scientific, Mainz, Germany).
The color intensities of the cross-sectional surface of the samples were determined using a colorimeter device (CR-400, Konika Minolta, Osaka, Japan). The CIE L*, CIE a*, and CIE b* were determined based on criteria established by the International Commission on Illumination for three-dimensional color measurement (Commision Internationale de I’E Clairage).
The TBARS analysis was conducted according to the method described by Lemon (1975), and the TBARS values were expressed as μmol malondialdehyde (MDA)/kg. A steam distillation method was used to determine the TVB-N level of the samples. The results obtained were given in mg TVB-N/100 g (European Commission, 2005).
In the study, coating application [control: uncoated (C), CH, CHT, and CHTRA)] and storage time (at 4±0.5°C, 0, 3, 6, 9, 12, 15, and 18 days) were considered as the factors. The experiments were set up in two replications using a random complete blocks trial plan in a 4×7 factorial order. The data obtained were subjected to analysis of variance, and significant mean values of the main sources of variation were compared using the Duncan multiple comparison test. The SPSS analyses were performed using the SPSS 22 software package (SPSS 22.0, 2013).
Results and Discussion
The overall effects of coating application and storage period on the microbiological and physico-chemical properties of rainbow trout fillets were given in Table 1 (mean±SD). The coating application had a very significant effect TMAB and total psychrotrophic bacteria (p<0.01). The control group showed the highest mean TMAB count. Similar results were observed for psychrotrophic bacteria. The mean lowest TMAB count was determined in CHTRA treatment. However, no significant difference was observed between CHTRA and CHT treatments with regard to psychrotrophic bacteria (p>0.05). On the other hand, it was determined that the coating application×storage period interaction had a very significant effect on both bacterial groups (p<0.01; Table 1). As shown in Fig. 1, the TMAB in the control group increased more rapidly after the 6th day compared to coating treatments. While the mean TMAB count of the control group was 108 CFU/g at the end of storage, the number did not exceed 106 CFU/g in the coated treatments. Moreover, the differences between coated treatments were not significant (Fig. 1). Psychrotrophic bacteria are the main group of microorganisms responsible for the spoilage of fresh fish stored at low temperatures (4°C). Therefore, the count of these bacteria is a reliable indicator of the quality of cold-stored fish meat (Shokri et al., 2020). The interaction of coating application and storage period also had a very significant effect on the number of psychrotrophic bacteria (p<0.01; Table 1). The number of psychrotrophic bacteria showed a similar trend to the number of TMAB (Fig. 2). All results indicated that coating treatments led to a significant reduction in bacterial counts. TiO2 or rosmarinic acid had no additional effect on the reduction of the psychrotrophic bacteria. As shown in Fig. 2, the coating treatment resulted in lower psychrotrophic bacterial counts than the control on all days of analysis. The differences between the coating treatments were not significant (Fig. 1). Ojagh et al. (2010) reported comparable increases in TMAB count in rainbow trout coated with CH enriched with cinnamon oil during cold storage, suggesting a prolonged storage period. Likewise, Echeverría et al. (2018) found a decrease of 2 logarithmic units in the TMAB counts on 15 day of the storage in nanocomposite–coated samples of tuna fish compared to the control group. On the other hand, in a study examining the effect of quince seed gum containing thyme or thyme essential oil on the shelf-life of rainbow trout fillets, it was reported that the number of psychrotrophic bacteria count in control group reached 108 CFU/g on day 18. In comparison, it remained at levels of 105–106 CFU/g in fillet samples with quince seed mucilage films containing thyme essential oil (Jouki et al., 2014). In a study investigating the effect of an edible active coating based on CH-sage essential oil nanoemulsion on the shelf life of rainbow trout fillets, psychrotrophic bacterial counts in the control group samples exceeded 108 CFU/g on day 16 of storage, while the coating treatment resulted in a count of 104–105 CFU/g (Shokri et al., 2020).
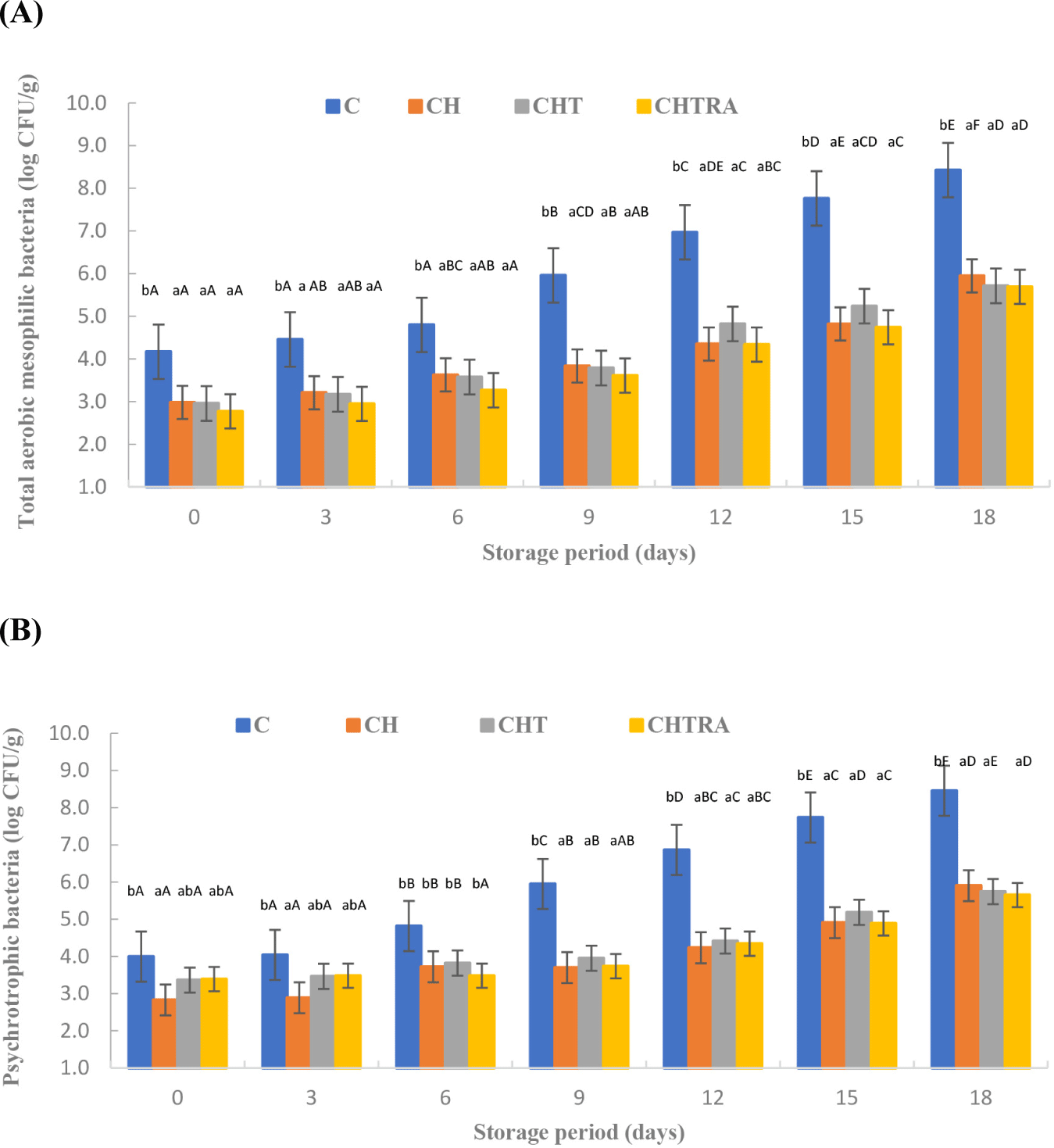
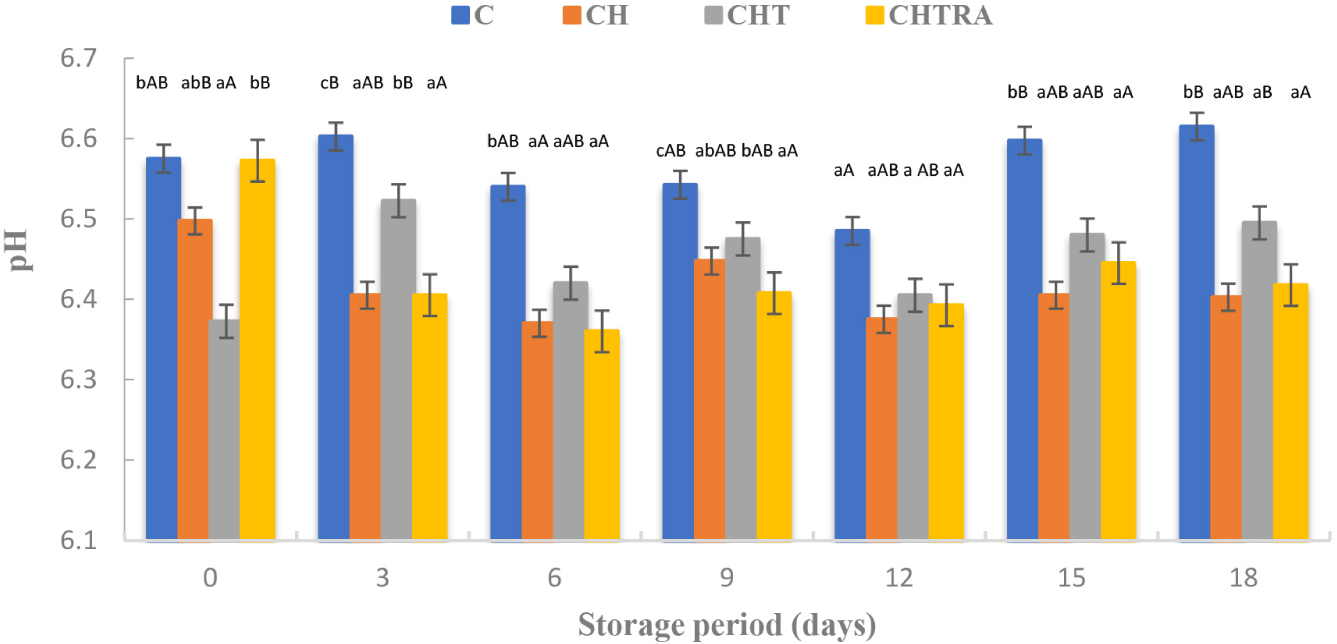
The antimicrobial properties of the CH coatings have already been reported in previous studies (Priyadarshi and Rhim, 2020). López-Caballero et al. (2005) found that a coating consisting of CH dissolved in acetic acid and gelatin exhibited an inhibitory effect on the Gram-negative flora of fish cakes. In our study, at the end of storage, the counts of Enterobacteriaceae were below the detectable level (<102 CFU/g; data not shown). Comparable results were also observed in another study on fish fillets (Volpe et al., 2015). Furthermore, Hisar et al. (2004) reported that MAP significantly decreased the count of Enterobacteriaceae in fillets.
The overall effects of coating application and storage period on pH value of rainbow trout fillets were given in Table 1 (mean±SD). The lowest mean pH value was observed in the control group. Changes in the average pH values were also observed during storage. As shown in Fig. 2, on the 15th day and 18th day of storage, higher pH values were observed in the control group than in the treatment groups. According to these results, the pH value in the coating groups was 6.50 or below at the end of storage, whereas the pH value of the control group was above 6.50. In other words, the pH change in the coating applications was limited. Berizi et al. (2018) also reported that trout fillets coated with CH and permanganate extract showed a lower pH value at the end of frozen storage than the control. An increase in pH during storage can adversely affect the quality of the product, especially with regard to sensory properties such as color, odor and texture (Alak et al., 2010).
The lipids of fresh fish are very susceptible to oxidation, which leads to changes in the quality characteristics of fish. The coating application had a significant effect on the TBARS value of rainbow trout fillets (p<0.05). The lowest mean TBARS value was observed in the CHTRA treatment. The differences among the other groups were not significant. In contrast, TBARS values increased with increasing storage time (Table 1). As shown in Fig. 3, the control group had a lower value than the other groups on the 9th day of storage, and the TBARS value for control was 13.52 μmol MDA/kg (<1 mg MDA/kg). On the other hand, the TBARS value for coating treatments were under 2 mg MDA/kg. Karki et al. (2023) reported that the tolerable TBARS value of fish products is 1 mg MDA/kg (100 μmol MDA/kg is equivalent to 7.2 mg/kg MDA). On the other hand, Xiong et al. (2021) reported that the threshold of TBARS value for oxidatitive ransidite and sensory acceptability ranged from 1–2 mg MDA/kg. On the following days of storage, significant increases in TBARS values were observed in all groups. The TBARS value on the 12th day of storage was below 2 only in CHT treatment. On the 15th day of storage, the TBARS value increased significantly in all groups (>3.0 mg MDA/kg). The highest TBARS value during cold storage was found in the CHTRA group at the end of storage. However, with regard to TBARS, no significant differences were observed among all treatments, including the control (Fig. 3). On the other hand, another study reported that CH coating on salmon fillets provided better results against lipid oxidation than gelatin coating (Xiong et al., 2021).
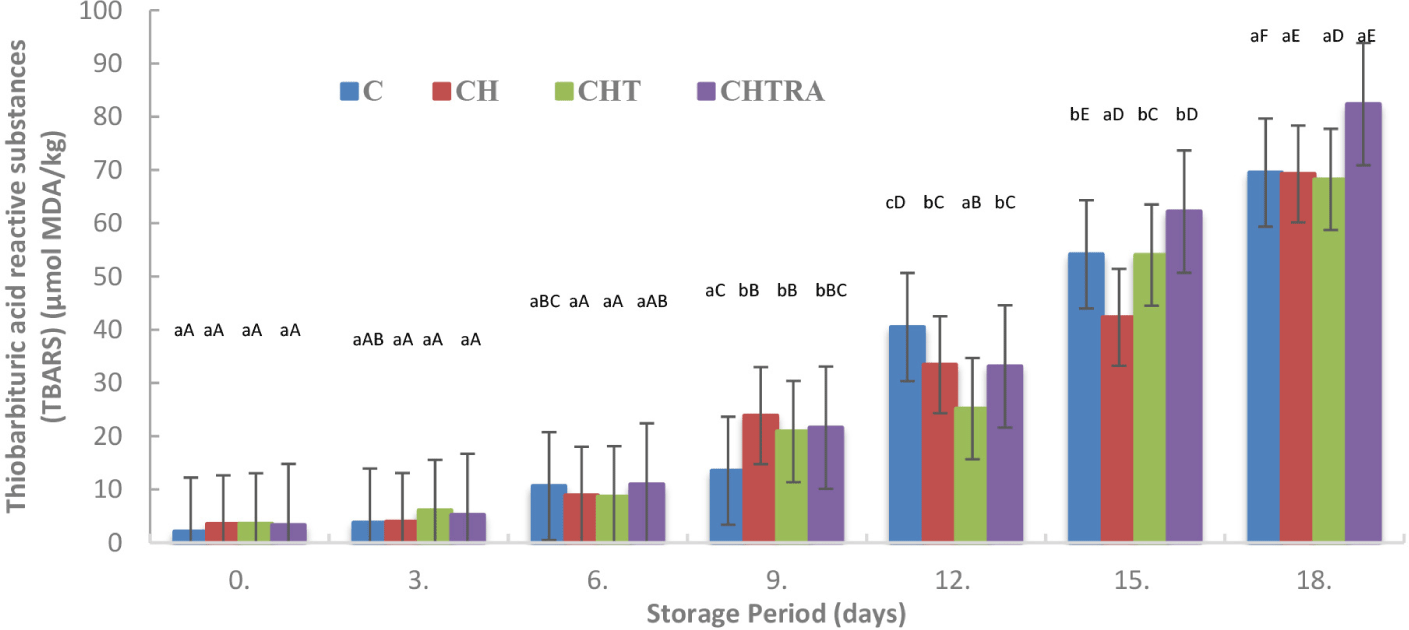
The determination of volatile nitrogenous compounds such as trimethylamine, dimethylamine, and ammonia, collectively referred to as the TVB-N (Shokri et al., 2020). The control group exhibited the highest mean TVB-N levels during storage. There was no significant difference between the coating groups. As the storage time increased, the mean TVB-N level increased (Table 1). The interaction of the coating application and storage period had a very significant effect (p<0.01) on the TVB-N level. These results were consistent with the microbiological results. It was observed that CH and TiO2 nanoparticles contributed to a significant decrease in the TVB-N values and significantly impacted the shelf-life of trout fillets. However, rosmarinic acid had no effect on TVB-N level (Fig. 4). As shown in Fig. 4, the highest TVB-N value was observed in the control group on the 12th, 15th and 18th days of storage. However, the differences between the coating treatments were not significant. It was reported that maximum acceptable TVB-N level for rainbow trout is 25 mg/100 g (Giménez et al., 2002). In our study, the TVB-N levels in the coated samples remained below this acceptable limit during storage period. In contrast, the TVB-N level in the control group exceeded the acceptable limit of 25 mg/100 g after 15 days (Fig. 4). Similarly, it has been reported that after 16 days of refrigerated storage, rainbow trout fillet samples coated with CH and CH combined with other substances remained below the acceptable limit, while the TVB-N level of the control group samples increased to 40 mg/100 g (Ojagh et al., 2010). In addition, López-Caballero et al. (2005) demonstrated that a protective CH-gelatin coating applied to fish balls significantly reduced TVB-N level. On the other hand, Korkmaz (2016) studied the effect of quinoa edible film on rainbow trout fillets and reported a TVB-N value of 20.35±0.49 mg/100 g in the control group and 18.65±0.21 mg/100 g in the experimental group after 12 days of storage.
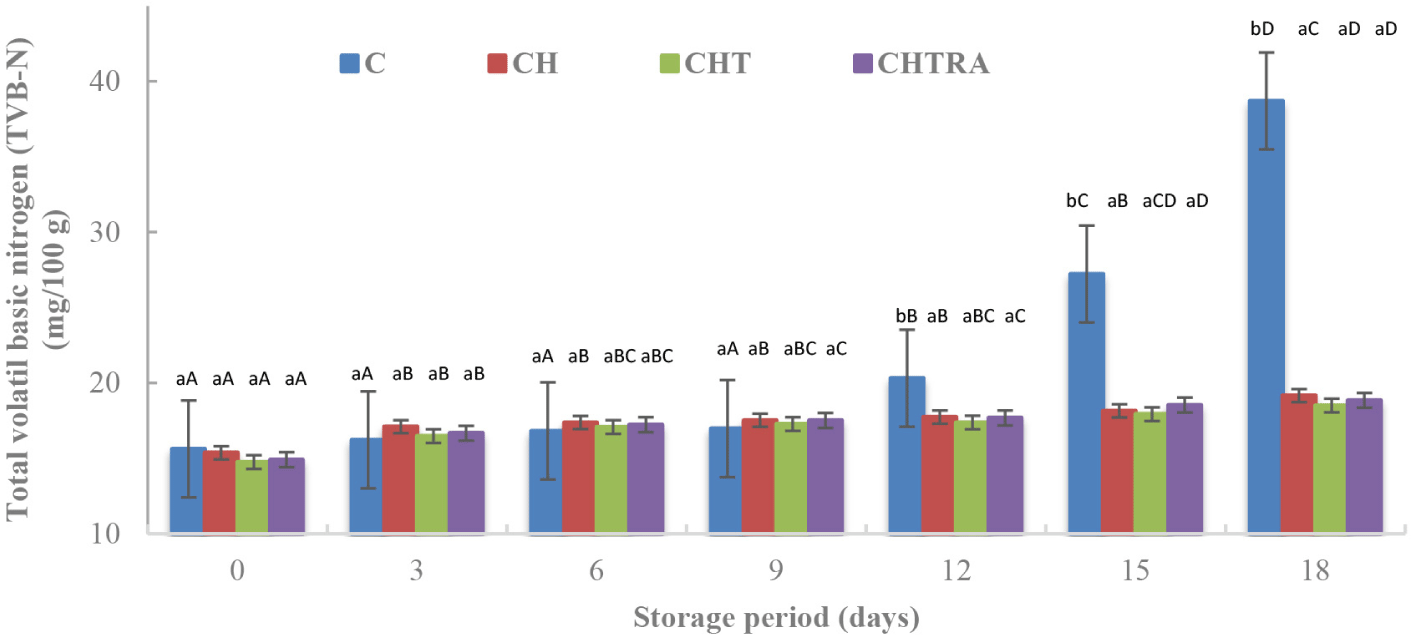
Physico-chemical changes that occur during storage can affect the appearance and texture of the fish (Zarandona et al., 2021). The coating application had a very significant effect the CIE L*, CIE a*, and CIE b* of rainbow trout fillets (p<0.01). The storage period was found to be very effective for the CIE L* and CIE b* (p<0.01). This factor also affected CIE a* (p<0.05; Table 2). Control and CH treatment had lower CIE L* than nanocomposite groups. In another study on sea bream, the highest CIE L* was found in the coating groups. As shown in Fig. 5, the lowest initial CIE L* was observed in the CH treatment. At the end of storage, the highest CIE L* was obtained with CHTRA treatment. However, the value of this treatment did not differ from that of the CH treatment. Considering these results, it can be concluded that the coating process (composite or nanocomposite coating) has a positive effect on the CIE L*. However, no remarkable changes were observed in the CIE a* and CIE b* in this study. Duan et al. (2010) also reported no changes in the CIE L*, CIE a* and CIE b* of fish samples stored at 2°C for three weeks.
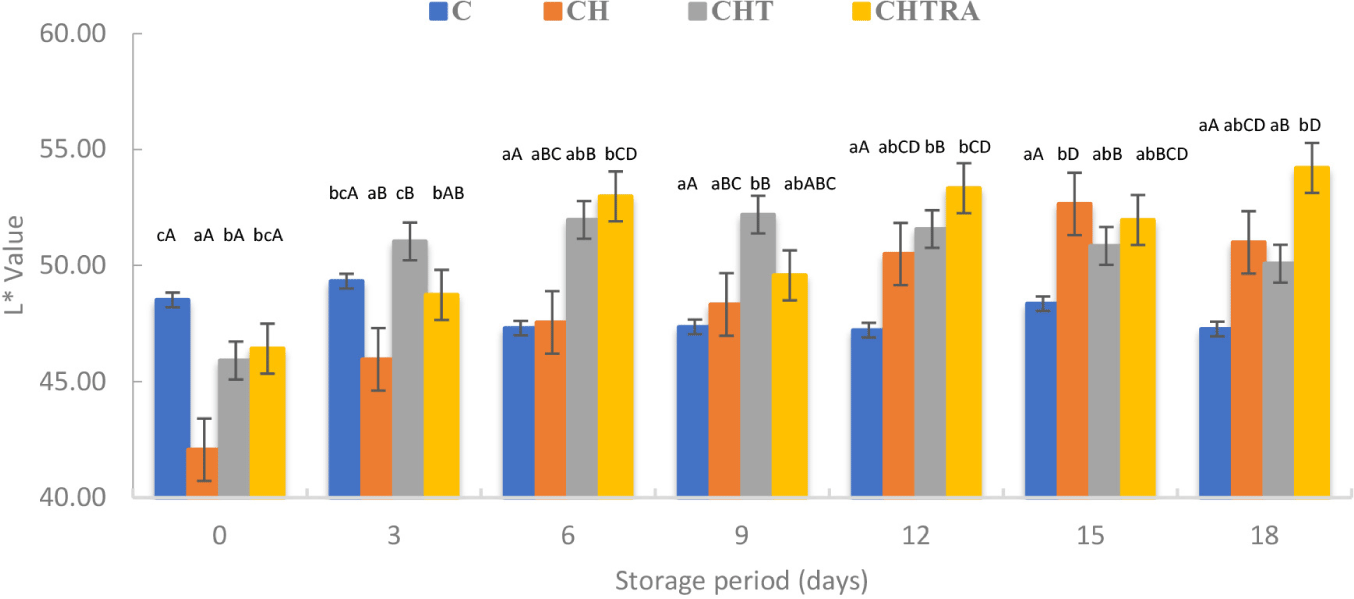
Conclusion
The results demonstrated that the coating application (CH, CHT, or CHTRA) effectively inhibited microbial growth in rainbow trout fillets during cold storage. In addition coating application showed the lower TVB-N level than control group. The TBARS value increased as the storage time increased. At the end of storage, no significant differences were observed between treatments in terms of TBARS (Fig. 3). It was also determined that the pH change in the coating applications was limited. On the other hand, the coating process has a positive effect on the CIE L*.