Introduction
Irradiation, as a method of meat preservation, is the most effective technology in the elimination of pathogenic microorganisms without compromising the nutritional properties and sensory quality of food (WHO, 1999). The wholesomeness of irradiated food has been permitted by international expert organizations such as the World Health Organization (WHO), the Food and Agriculture Organization (FAO), the International Atomic Energy Agency (IAEA), and the US Food and Drug Administration (FDA) (Sohn et al., 2009). Since then, significant progress has been made in this respect by using irradiation doses lower than 10 kGy to control the growth of pathogenic and spoilage bacteria on meat and meat products, such as Listeria monocytogenes and Salmonella Typhimurium (Fu et al., 1995). A number of researchers have investigated the effect of irradiation on microbial, sensory, and nutritional qualities of beef (Fu et al., 1995). Their results indicated that irradiation reduced microbial loads on beef without adversely affecting the sensory and nutritional values of meat. Among the irradiation, electron-beam (EB) irradiation is used to inactivate foodborne pathogens during storage and to provide the hygienic quality by reducing microbial spoilage of foods (Sarrias et al., 2003). EB irradiation has merit in industry due to the short processing time, low temperature rise, and consumer-friendliness, as it does not generate radioactive waste (Hong et al., 2008).
Beef aging is widely used by the meat industry to improve tenderness and flavor, resulting in a more acceptable product for consumers (Troy and Kerry, 2010). Most of the research on the aging of beef has been concerned with the meat quality and sensory traits (DeGeer et al., 2009). Temperature and time are the variables that can be controlled to affect aging in meat (Lee et al., 1996). One of the important concerns of beef industry is that meat quality does not deteriorate during extended aging and distribution.
The radiation technology can be applied for beef to secure the microbial problems during the extended aging time or high temperature aging. Even though differences between the different aging methods have been evaluated, no comparison study on the aging time and temperature on beef quality were carried out. The present study was conducted to determine the effects of EB irradiation and aging temperature on quality and safety in beef loins during storage.
Materials and Methods
Beef loins (Longissimus dorsi) were purchased from a local market in Daejeon, Korea. They were trimmed of all visible fat, and subsequently sliced to 1 cm thick pieces using a meat slicer (HFS 350G, Hankook Fugee Industries Co. Ltd., Korea). The samples was individually packaged using a vacuum-packaging machine (Multivac, model 1960/10, type AG800, Germany), stored at 5℃ overnight, and irradiated next day morning.
For the inoculation test, samples were randomly selected and sterilized using EB irradiation (35 kGy at 2.5 MeV) with a linear electron beam RF accelerator (EB tech, Korea). Escherichia coli O157:H7 (ATCC 43894), and L. monocytogenes (KCTC 3569) were obtained from the Korean Collection for Type Culture (KCTC, Korea). E. coli O157:H7 and L. monocytogenes were cultivated in tryptic soy broth and agar (Difco Laboratories, USA) at 37℃ for 48 h. The cultures were then centrifuged (3,000 g for 15 min at 4℃). The resulting pellet was washed twice with sterile saline solution (0.85%) and suspended in the same saline solution. The viable cell density was approximately 108 CFU/mL. The samples (5 g, approximately 3.5 cm × 3.5 cm) were inoculated with 100 μL of this solution. Each sample was then resealed and shaken for homogenization.
Each prepared sample was irradiated on both sides in a linear EB RF accelerator (Energy 10 MeV, beam power 40 kW, EB Tech., Korea). The beam current was 0-7 mA. Irradiation was performed with a conveyor velocity of 5.16 m/min and a dose rate of 2.95 kGy/s. Because the incident EB had a low penetration power, all the samples were sliced to a 1 cm thickness to enhance the effectiveness of irradiation. To confirm the target dose, alanine dosimeters, attached to the top and bottom surfaces of the sample packs, were read using a 104 Electron Paramagnetic Resonance unit (EMS-104; Bruker Instruments Inc., USA). The calculated maximum/minimum dose ratio was less than 1.004 for all samples. The doses employed in this study were 0, 0.5, 1, 2, 3, 4, and 5 kGy. The experiment was replicated 4 times and 3 observations were made during each experiment. After irradiation, the samples were immediately stored under commercial storage conditions at 25℃, until further analysis.
After irradiation, each sample (5 g) was cut into small pieces (approximately 0.5 cm × 0.5 cm) and homogenized for 2 min in a sterile Stomacher bag containing 45 mL of sterile saline solution using the Stomacher BagMixer® 400 (Interscience Co., France). Then samples were serially diluted in sterile saline (0.85%) solution, and each diluent (0.1 mL) was spread on each bacterial media. Tryptic soy agar (Difco Laboratories) was used for E. coli O157:H7 and L. monocytogenes. Plates were incubated at 37℃ for 48 h, and microbial counts were expressed as colony forming units per gram (CFU/g). Radiation sensitivity of the pathogens was calculated as D10, a value that represents the dose required to inactivate 90% of the microbial population.
Each prepared sample (non-sterilized) was irradiated using the same conditions previously described above. The applied dose at meat samples was 2 kGy. Until irradiation was over, non-irradiated control (0 kGy) samples were kept in ice. After irradiation, the irradiated and non-irradiated samples were immediately returned to a 4℃ cold room and aged for 8 d. Microbiological, shear force, lipid oxidation, color and nucleotides analyses of the samples were performed on non-irradiated and irradiated samples during ageing. Beef loins with 3 different aging temperatures (2, 10, and 25℃) were determined on 0, 4, and 8 d of storage to establish optimal conditions of ageing at different temperatures and times of beef loins.
The sample (5 g) was aseptically homogenized for 2 min in a sterile Stomacher bag containing 45 mL of sterile saline solution using the Stomacher BagMixer® 400. Subsequently, the homogenized samples were serially diluted in sterile saline and plated by spreading 0.1 mL on a total plate count agar (Difco Laboratories) and eosin methylene blue agar (EMB; Difco) for TAB counts and total coliform counts, respectively. Plates were then incubated at 37℃ for 48 h. For enumerating mold count, molds were plated onto YM agar (containing 10% citric acid, pH 3.6, Difco Laboratories), and the plates were incubated for 5 d at 25℃. The number of colonies was counted and expressed as colony forming units per gram (CFU/g).
Shear force (kgf) was determined using a Texture Analyzer (Model TA-XT 2i, Stable Micro system Ltd., UK) with a Warner-Bratzler blade attachment. Cross-sections (across to the fibers) as close as possible to 25 × 230 × 350 mm were cut from each treatment and samples were measured for shear force. The cross sections of samples were placed at middle to the blade. Crosshead speed was 200 mm/min and full-scale load was 50 kg.
Lipid oxidation in samples was measured using 2-thiobarbituric acid reactive substance (TBARS) values, according to the modified method described by Ahn et al. (1998). The sample (3 g) was added to 9 mL of 1 N NaOH with 100 μL of 7.2% butylated hydroxyl toluene and was hydrolyzed in a shaking water bath at 60℃ for 1 h. The hydrolysate was filtered (filter paper, Whatman no. 1) after stirring with 6 mL of 40% trichloroacetic acid (TCA) by vortexing for 30 s. The filtrate (1 mL) was mixed with 20 mM 2-thiobarbituric acid (1 mL), and the mixture was kept in a boiling water bath (90℃) for 40 min, cooled, and then centrifuged at 2,090 g for 15 min. The absorbance of the supernatant was measured at 532 nm with a spectrophotometer (DU 530; Beckman Instruments Inc., USA). The amount of malondialdehyde was calculated using a standard curve prepared from tetraethoxypropane, and the TBARS value was reported as mg malondialdehyde/kg meat.
The color of the beef sample surface was evaluated using a Color Difference Meter (Spectrophotometer CM-3500d, Konica Minolta Sensing, Inc., Japan), and Hunter color values, L* (lightness), a* (redness), and b* (yellowness) were determined. The instrument was calibrated to standard black and white plates before analysis. The Hunter values were monitored by a computerized system using spectra magic software (Konica Minolta Sensing, Inc., Japan). Measurements were performed in triplicate.
The meat samples (5 g) were mixed with 25 mL of 0.7 M perchloric acid and homogenized (T25b, Ika Works, Malaysia) for 1 min at 1,130 g to extract nucleic acids. The extracted nucleic acids were centrifuged (Union 32R, Hanil Co., Ltd., Korea) for 15 min at 2,090 g (4℃) and filtered through Whatman No. 4 filter paper (Whatman Inc., England). The supernatant was then adjusted to pH 7 with 5 N KOH (SevenEasy, Mettler-Toledo Int. Inc., Switzerland). The pH-adjusted supernatant was placed in a volumetric flask and adjusted to a volume of 100 mL with 0.7 M perchloric acid (pH 7). After 30 min of cooling, the mixture was centrifuged (Union 32R) at 2,090 g (4℃) and the supernatant was filtered through a 0.2-μm PVDF syringe filter (Whatman). The filtrate (5 mL) was analyzed using HPLC (ACME 9000, Younglin Instruments Inc., Korea). The analytical conditions for HPLC included a Waters-Atlantis C18 RP column (4.6 × 250 mm, 5 μm particles, Waters Co., USA), with a mobile phase of 0.1 M triethylamine in 0.15 M acetonitrile (pH 7.0). The flow rate of the mobile phase was 1.0 mL/min, and the injection volume was 10 μL. The column temperature was maintained at 35℃ and detection was monitored at a wavelength of 260 nm. The peaks of individual nucleotides were identified using retention times for the following standards: hypoxanthine, inosine, inosine-5-phosphate (IMP) (Sigma, USA); the concentrations were calculated using the area for each peak.
The experiment was conducted as 3 independent trials with 3 observations for each treatment combination in each trial. Data were analyzed using SAS software (Release 8.01, SAS Institute, Inc., USA). Statistical analysis was performed by two-way analysis of variance (ANOVA). When significant differences were detected, the differences among the mean values were determined by the Duncan’s multiple comparison test at a confidence level of p<0.05. Mean values and standard errors of the mean are reported.
Results and Discussion
L. monocytogenes and E. coli were initially loaded at 8.83 and 8.05 Log CFU/g in beef loin samples, respectively (Fig. 1). Irradiation of 4 kGy resulted in a 6-7 decimal reduction in the number of L. monocytogenes and E. coli. In addition, the irradiated samples had a significantly lower L. monocytogenes and E. coli population at each irradiation dose compared to non-irradiated sample. The initial population of L. monocytogenes and E. coli significantly decreased in beef loin samples, with increasing irradiation dose. Bacterial populations in samples were below the detection limit (101 CFU/g) following irradiation at 5 kGy. A similar result was reported that EB irradiation of 2 kGy reduced the population of L. monocytogenes and E. coli to an undetected level in pork jerky (Kim et al., 2014). Foong et al. (2004) confirmed that the doses of EB irradiation needed for a 3-log reduction of L. monocytogenes were 1.5 kGy for bologna, roast beef, and turkey, and 2.0 kGy for frankfurters and ham.
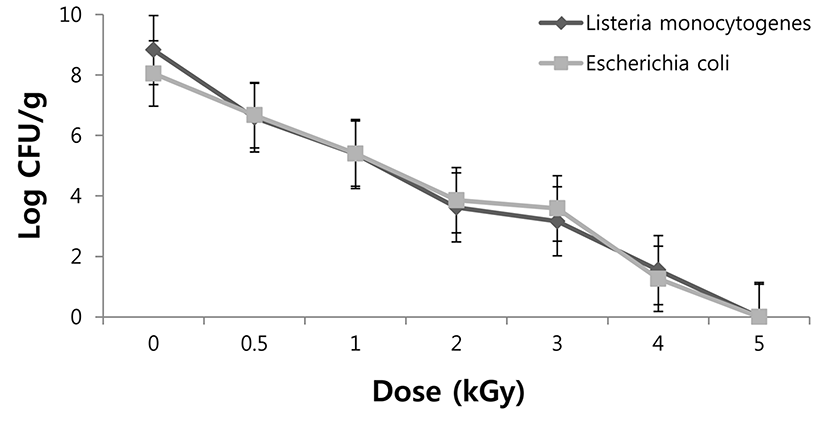
The Irradiation decimal reduction (D10) value for L. monocytogenes and E. coli inoculated in beef loin samples was 0.67 kGy and 0.64 kGy, respectively (data not shown). D10 values of bacteria in food depends on several factors, including water activity, food composition, irradiation or storage temperature, and presence of oxygen (Mendoca, 2002). In addition, some of the constituents of complex food system, such as proteins, are thought to compete with cells for interactions with radiolytic free radicals, thereby reducing the net effect of radiation damage and making the organisms more radiation-resistant (Jo et al., 2004). D10 values for L. monocytogenes and E. coli in pork jerky ranged from 0.18 to 0.25 kGy (Kim et al., 2014). Clavero et al. (1994) reported that D10 values for E. coli O157:H7 in ground beef at 5℃ was reported to be 0.27 kGy. Sommers et al. (2003) found that bologna inoculated with L. monocytogenes had a D10 value of 0.56 kGy after gamma irradiation. The present study showed that EB irradiation was found to be effective in sterilization and inhibition of survival of L. monocytogenes and E. coli in beef loin samples.
The effect of different aging temperature on total TAB in non- and irradiated beef loin during the storage period is shown in Table 1. Aging periods, temperature, and irradiation influenced TAB of beef loin. EB irradiation improved the safety of beef loin by reducing the TAB. TAB counts of non-irradiated samples were higher than those of irradiated ones at a storage time. Niemand et al. (1981) demonstrated that the number of TAB was reduced by 99.9% with a dose of 2 kGy. TAB was in the range 3-4 Log CFU/g at 4 d, regardless of irradiation and reached a level of 5-6 Log CFU/g after 8 d. With higher aging temperature, TAB increased. TAB at 25℃-aging samples were higher than 2 or 10℃ in irradiated samples, showing not much difference between 2 and 10℃ samples. Lee et al. (1996) showed that irradiation resulted in a greater reduction in bacteria counts at the beginning of aging, but later the numbers was influenced by aging temperature. Our results showed that after 4 d, TAB proliferated rapidly in non-irradiated aged at high temperatures. Coliform bacteria were not detected in pork jerky in this study (data not shown). Our investigation suggested that EB irradiation was effective reducing microbial levels in beef loin samples.
1)Standard error of the means (n=9).
a-cValues with different letters within the same column differ significantly (p<0.05).
x,yValues with different letters within the same row differ significantly (p<0.05).
The effect of different aging temperature on shear force values in non- and irradiated beef loin during the storage period is shown in Table 2. As aging periods and temperature increased, shear force values decreased despite of irradiation. The decrease in shear force can be attributed to the structural disruption of myofibrillar components occurring during the ageing (Ba et al., 2014). Many studies have found that as the beef loin was aged longer at high temperature shear force values decreased resulting in improvement in meat tenderness (Monson et al., 2004). In the current study, aging time and temperature could affect shear force value and improve tenderness for beef loin. Especially, aging of the samples at 10 or 25℃ after 5 d brought about reduced shear force values compared with those at 2℃.
1)Standard error of the means (n=9).
a-cValues with different letters within the same column differ significantly (p<0.05).
x,yValues with different letters within the same row differ significantly (p<0.05).
Lipid oxidation was influenced by aging time, temperature, and irradiation (Table 3). As aging periods and temperature increased, TBA values increased in both non-irradiated and irradiated samples. This result agrees with several authors reporting aging time and temperature influenced lipid oxidation (Lee et al., 1996; Nam et al., 2003). In our study, TBA values at 25℃ ageing samples were higher than that at 2 or 10℃ in irradiated samples, and there was not much different between 2 and 10℃ samples. Lipid oxidation could be reduced at low temperature storage and these results are consistent with a previous study (Lee et al., 1996). A possible explanation can be made that lipid oxidation in the irradiated beef was delayed due to growth of microorganisms at high temperature (Lucke, 1994). Previous studies have found that irradiation increased lipid oxidation of vacuum-packaged beef (Dempster et al., 1985). However, the results of our study suggest that irradiation dose at 2 kGy did not increase the values in most of samples. It was reported that the TBARS value above 0.5 mg MDA/kg indicates a level of lipid oxidation products which impart a rancid flavor and odor that can be detected by consumers (Wood et al. 2008). Therefore, it can be concluded that aging up to 4 d at 10℃ did not result in excessive oxidation of lipids.
1)Standard error of the means (n=9).
a-cValues with different letters within the same column differ significantly (p<0.05).
x,yValues with different letters within the same row differ significantly (p<0.05).
Meat color is the crucial factor for meat acceptability when consumer purchases it. The effect of different aging temperature on color values in non- and irradiated beef loin during the aging is shown in Table 4. Ahn and Nam (2004) noted that the L* value increased as the storage time increased. Regardless of irradiation, the degree of aging was a critical factor on the redness of beef during storage and aging increases L* value of beef (Nam and Ahn, 2003). Irradiation was the most critical factor on decreased a* values of beef, and irradiated samples had lower a* values than non-irradiated. The gaps of a* values between non-irradiated and irradiated samples were reduced with increase of aging storage. Nam and Ahn (2003) reported that a* values of short term-aged beef were not changed much during storage and the redness was higher in nonirradiated than irradiated beef. This result agrees with many authors who reported that vacuum-packaged irradiated beef reduced a* and b* values during storage (Ahn and Nam, 2004; Nam and Ahn, 2003; Nanke, 1998). Reflectance data indicated that both O2-Mb and Fe3+-Mb developed but a significant decrease in red and increase in brown pigments could develop at dose levels as low as 1.5 kGy in vacuum-packaged beef (Brewer, 2004). Luschinger et al. (1997) reported that irradiation at 2.0 and 3.5 kGy initially darkened and reduced the redness of raw beef patties in aerobic or vacuum packaging, but the color difference disappeared with display time. Nanke (1998) suggested that color changes of irradiated raw meat were species, irradiation dose, and packaging type dependent.
1)Standard error of the means (n=9).
a-cValues with different letters within the same column differ significantly (p<0.05).
x,yValues with different letters within the same row differ significantly (p<0.05).
Nucleotide-related compounds result from the decomposition of adenosine-5-triphosphate (ATP) (Flores et al., 1999). ATP is the major compound in muscle before slaughter, whereas muscle after slaughter contains large amounts of inosine monophosphate (IMP) and small amounts of ATP, adenosine-5-monophosphate (AMP), and adenosine-5-diphosphate ADP (Lee and Lee, 2001). ATP converts into AMP by dephosphorylation and then becomes to IMP providing good taste and flavor to the meat. IMP changes to inosine, which has a sour taste and then to hypoxanthine, which has a bitter taste (Tikk et al., 2006). The nucleotide-related compounds of beef loin were influenced by aging time and temperature (Table 5). In our study, the amount of IMP decreased with the increase of storage whereas the inosine and hypoxanthine increased, indicating IMP was transformed into inosine and hypoxanthine. These results are consistent with a previous study (Dashdorj et al., 2013), where they indicated the IMP conversion took place strongly during aging by endogenous enzymes and/or by microbial activity, which may explain the opposite changes between IMP, inosine, and hypoxanthine during the aging. As aging temperature increased, the IMP decreased and hypoxanthine increased. Especially, 25℃-aged samples had lower IMP and higher hypoxanthine than 2 or 10℃, but there was small different between 2 and 10℃ samples. Our results showed that IMP decreased and hypoxanthine increased rapidly after 5 d at 25℃. Thus, in terms of nucleotide-related flavor compounds, aging at 25℃ of irradiated beef loins were not beneficial.
1)Standard error of the means (n=9).
a-cValues with different letters within the same column differ significantly (p<0.05).
x,yValues with different letters within the same row differ significantly (p<0.05).
Conclusion
EB irradiation was shown to be an effective method for reducing the risk of foodborne pathogens of beef loins. Thus EB irradiation can be applied to beef products which is supposed to be aged at relatively higher temperature than conventional refrigerated temperature, to accelerate aging process and to shorten the aging time and cost. Based on the results of microbiological and physicochemical properties of beef irradiated at 2 kGy, aging at 25℃ would not be recommended for a high temperature aging method because the samples had problems microbial growth and undesirable conformation of nucleotide-related flavor compounds. However, the conditions could also be changed by elevating irradiation dose.