Introduction
Milk contains a wide range of biologically active compounds such as immunoglobulins, antimicrobial peptides, oligosaccharides, lipids and other minor components (Korhonen and Pihlanto-Leppälä, 2004a; Park, 2009), conferring important physiological and biochemical functions that have crucial impacts on host metabolism and health (Gobbetti et al., 2007; Park 2009). In addition, milk fermentation by lactic acid bacteria releases a large number of peptides with various biological functions, such as antioxidant (Chang et al., 2013), angiotensin-converting enzyme inhibitory (Ha et al., 2015), immunomodulatory (Gauthier et al., 2006), opioid activities (Park, 2009).
Among them, antioxidant peptides have free radical-scavenging activity and nonenzymatic lipid oxidation (Rival et al., 2001; Suetsuna et al., 2002). The side effect of oxidation in the body and in foodstuffs is well known. Excessive free radicals and other reactive oxygen species can deteriolate food quality, for example unacceptable taste, rancid flavor and shortening of shelf life (Korhonen and Pihlanto-Leppälä et al., 2007b). In addition, oxidative damage plays a significant role in the number of age-specific diseases, such as atherosclerosis, diabetes, rheumatoid arthritis, Alzheimer’s disease and cancer (Halliwell, 2001; Hoelzl et al., 2005; Korhonen and Pihlanto-Leppälä et al., 2007b). Therefore antioxidant peptides can then be utilized as an excellent source of natural ingredients to develop functional foods that may enhance physiological and metabolic functions or condition of the body to benefit the host (Clare et al., 2003; FitzGerald and Meisel, 2003; Kitts and Weiler, 2003; Li et al., 2004).
Different studies have demonstrated antioxidative activity of dairy products (De Gobba, C. et al., 2014; Gupta et al., 2009; Sabeena Farvin et al., 2010). Several peptides from milk hydrolysates or fermented milk have been reported to have antioxidative activity. Rival et al. (2001) identified a peptide with antioxidative property from the tryptic β-casein digest. Suetsuna et al. (2002) and Lopez-Exposito et al. (2006) also reported antioxidative peptides from the peptic digests of αs1- and κ-casein, respectively. Recently, Chang et al. (2013) identified two antioxidative peptides from the bovine β-casein hydrolysate fermented by Bifidobacterium longum. However, to date, only a few antioxidant peptides from fermented milk products have been identified and characterized.
The aim of this study was to identify and characterize novel antioxidant peptide from fermented milk which has not been identified yet. Moreover, the peptide was synthesized according to the obtained amino acid sequences to confirm its antioxidative activity.
Materials and Methods
An on-line screening method for antioxidative activity was developed based on the method of Koleva et al., (2000), as given in Fig. 1. Samples were subjected to reverse phase-HPLC (Ultimate 3000 RSLC HPLC, Dionex, Germany) to allow separation in the column (Zorbax, 3.5 um, 300SB-C8, 2.1*50 mm, Agilent). Each peak was detected by a diode array detector (DAD) at 280 nm. Then 2,2'-azino-bis(3-ethylbenzothiazoline-6-sulphonic acid) (ABTS) radical solution was pumped into the separated fractions on-line at a flow rate of 0.5 mL/min. A peak showing a negative absorbance at 734 nm after reacting with the ABTS reagent was indicative of antioxidant activity. Antioxidative activity selection was done using an on-line ABTS reaction assay by pumping an ABTS radical solution into the separated fractions on-line with a flow rate of 0.5 mL/min. The peak showing negative absorbance at 734 nm after reaction with the ABTS reagent indicates antioxidative activity. Among the strains tested, the strain Lactococcus lactis SL6 was the one showing the antioxidative activity using the ABTS method and was thus used for the next experiments.
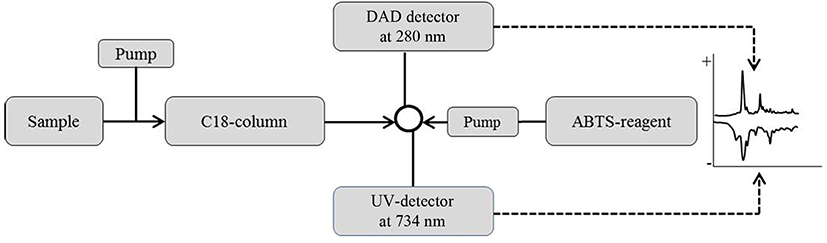
Lactic acid bacteria (LAB) isolated from various traditional fermented foods were used for screening of the strain which has antioxidative activity. The LAB isolates were incubated in skimmed milk (10%, w/v) and incubation at 37°C for 48 h. Then the fermented milk was centrifugated at 8,000 g for 15 min at 4°C, and the supernatant was treated with ammonium sulfate (60%) to remove the precipitate. Finally the resulting supernatant was dialyzed to remove salts using Spectra/Por dialysis membrane (Spectrum labs, USA) with a molecular weight cut-off of 1 kDa and used for on-line screening of antioxidant peptides.
Selection and identification of peptides were performed using an online platform (Fig. 2) following the methods previously described by Chang et al. (2013). The samples were analyzed in a Triple TOF 5600 Q-TOF LC/MS/MS system (ABSciex, CA) using a Ultimate 3000 RSLC HPLC system (Thermo Fisher Scientific Inc., USA), including a degasser, an auto-sampler, diode array detector and a binary pump. The LC separation was performed on a column with a mobile phase A (0.1% formic acid in water) and a mobile phase B (0.1% formic acid in acetonitrile). The flow rate and injection volume were 0.25 mL/min and 1-5 μL, respectively. Mass spectra were acquired under positive electrospray ionization (ESI) with an ion spray voltage of 4500 V. Two full-scan mass spectra were acquired over an m/z range of 50-2000 on the MS mode. The data were collected using Analyst TF 1.7 software and analyzed using PeakView 2.2 and ProteinPilot 4.5 software.

The antioxidant activity of the sample was measured as free radical scavenging activity of ABTS and 1,1-diphenyl-2-picryl hydrazyl (DPPH) radical cation according to the previously described method by Chang et al. (2013) and Volta et al. (2014) with minor modifications.
The ABTS radical was generated by preparing ABTS and potassium persulfate at final concentrations of 7 and 2.45 mM, respectively, and incubated for 12 h in the dark at room temperature. The ABTS radical was adjusted to an absorbance of 0.70±0.02 at 734 nm by diluting with distilled water using a spectrophotometer. The sample (10 μL) was mixed with 990 μL of the ABTS radical solution. Changes in absorbance were monitored at 734 nm after 30 min at room temperature.
For DPPH radical scavenging assay, DPPF solution (0.1 mM) in ethanol was prepared. This solution (990 μL) was added to synthetic peptide resolved solution (10 μL). The mixture was mixed vigorously and incubated at room temperature for 30 min. Then, absorbance was measured at 517 nm by using UV-spectrophotometer (Shimadzu, Japan).
All assays were carried out in triplicates and recorded as means. The percentage ABTS and DPPH antioxidant activity were calculated following the equation below where Acontrol represents the initial ABTS/DPPH absorbance:
ABTS/DPPH Scavenging activity (%) = [1 - (Acontrol - Asample) / Acontrol] × 100
The half maximal inhibitory concentration (IC50) value based from the calculated standard curve and expressed as μM of 6-hydroxy-2,5,7,8-tetramethylchroman-2-carboxylic acid (Trolox) (Sigma, USA). A standard curve was prepared using various concentrations of Trolox, a water-soluble analogue of vitamin E.
The antioxidative peptide identified by RP-HPLC-tandem mass spectrometry and its derivatives were chemically synthesized by the solid-phase procedure by Bead-Tech, Inc. (Korea). The purity was verified as >95% by using analytical HPLC coupled with MALDI-TOF MS. The peptides were dissolved in high-performance liquid chromatography (HPLC)-grade water at a concentration of 1 mg/mL for the ABTS redical scavenging test.
Results and Discussion
On-line screening system of antioxidative activity using RP-HPLC was performed, as described in Fig. 1. First, samples were injected into RP-HPLC and separated by C18 column, and each peak was detected by DAD detector (280 nm). Then, ABTS reagent was pumped into the separated fractions on-line using isocratic pump to mix each other well. Finally, the fraction showing negative absorbance at 734 nm after reaction with ABTS reagent was regarded indicating antioxidative activity and was collected for further experiments.
Among tested strains, Lactococcus lactis SL6 (KCTC 11865BP) showed strongest antioxidative activity. L. lactis SL6, provided by Cell Biotech, Co. (Korea), was originally isolated from kimchi. Fig. 2 shows the UV-visible chromatogram of the supernatants of skim milk fermented by L. lactis SL6. The retention time (Rt) 5 min showed high negative absorbance at 734 nm was its antioxidative activity was confirmed by ABTS·+ radical cation assay (data not shown). These results indicated that the fermentation by SL6 plays an important role in the release of antioxidant peptides from milk proteins.
The HPLC fraction showing high antioxidative activity was subjected to MS/MS spectrometry using a quadrupole ion trap capable of multiple stages of mass analysis from a single precursor ion. Fig. 3A shows the UV chromatogram obtained at Rt near 5 min from Fig. 2, and a dominating peak at 5.596 min was analyzed further. Fig. 3B shows the MS spectrum of the sample from Fig. 3A and one fragment which was the triply charged ion (m/z 788.38, 3+), indicating with an arrow, among intense fragments only matched to bovine milk peptide. Following sequence interpretation and database searching using PeakView 2.2. and ProteinPilot 4.5 softwares, the MS/MS spectrum was matched to C-terminal fragment of αs1-casein (f179-199). The amino acid sequence of the identified peptide with triply charged ion (m/z 788.38, 3+) corresponded to the loss of three protons from the precursor ion. The corresponding amino acid sequence was identified as FSDIPNPIGSENSEKTTMPLW (2,362 Da), as shown in Fig. 4. This peptide characterized in this study has never been identified as antioxidant peptide yet, to the best of our knowledge.
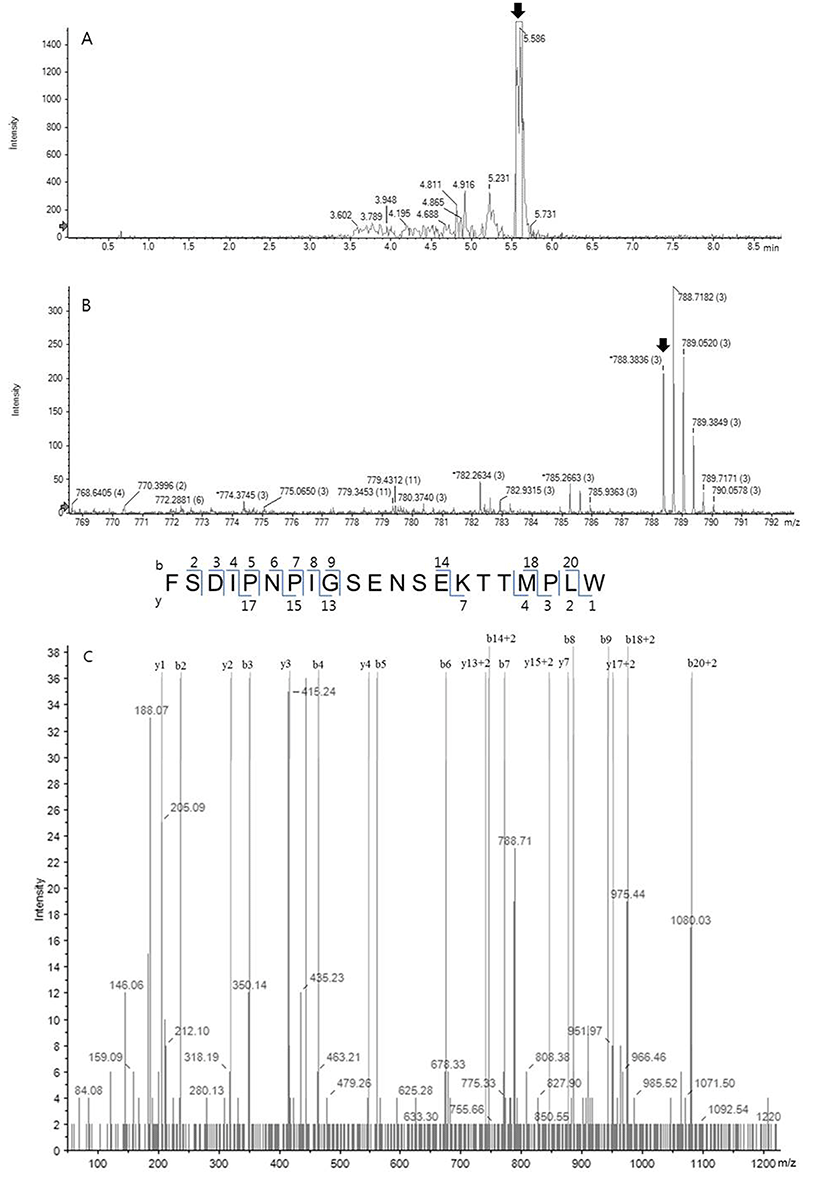
To confirm whether the identified peptide has antioxidative activity, it was chemically synthesized by solidphase procedure, and further evaluated for their antioxidative activity using both ABTS and DPPH scavenging activity assay.
As shown in Table 1, the synthesized peptide showed radical scavenging activities with ABTS scavenging of 70.83±1.15% and DPPH scavenging of 89.73±1.19%. The synthesized peptide quenched ABTS radicals more effectively. The hydroxyl radicals scavenging effect of the peptide (IC50: 28.25±0.96 μM) showed a slightly lower radical scavenging activity than that of the natural antioxidant Trolox (IC50: 15.37±0.52 μM) as positive control (Table 2).
Synthetic peptide sequence | Radical scavenging activity1) | |
---|---|---|
ABTS2) | DPPH3) | |
FSDIPNPIGSENSEKTTMPLW | 70.83±1.15% | 89.73±1.19% |
1)Each observation is mean of triplicates of each batch. Results are expressed as mean of scores ± standard error of mean.
2)ABTS radical scavenging was tested at 5 μM
3)DPPH radical scavenging was tested at 50 μM.
Synthetic peptide sequence | IC502) ± SEM3) | |
---|---|---|
Trolox1) | 15.37±0.52 μM | |
FSDIPNPIGSENSEKTTMPLW | 28.25±0.96 μM |
1)Trolox: a water-soluble analogue of vitamin E.
2)The concentration of needed to inhibit 50% of the radical scavenging.
3)Each observation is mean of triplicates of each batch. Results are expressed as mean of scores ± standard error of mean.
Antioxidative activity of peptides is closely related to their amino acid composition, structure, and sequences (Sarmadi and Ismail, 2010). In addition, many antioxidant peptides contains at least one proline residue and higher antioxidant capacity of the peptide was related to their high proline content (Hernández-Ledesma et al., 2005). Accordingly, the antioxidant peptide identified in this study contained three proline residues (Fig. 4).
In order to evaluate the importance of proline residues in antioxidative activity of the peptide, proline residues in the template peptide were replaced by alanine and checked their antioxidative activity by ABTS radical scavenging assay. As shown in Fig. 5, significant decrease in the antioxidative activity was observed in M-AAA variant in which all proline residues were replaced by alanine. In contrast, the antioxidant activity of the M-AA variant in which only two proline residues in the N-terminal region were replaced by alanine remained the same. These findings suggest that 3rd proline in C-terminal region, in particular, is critical for antioxidative activity of the peptide identified in this study. The distinctive cyclic structure of proline's side chain gives proline an exceptional conformational rigidity of the protein (Williamson, 1994). Therefore 3rd proline might affect the secondary structure of the peptide, then changing the antioxidative activity of the peptide. This should be elucidated in the future.
Additional manipulation of the template peptide was performed, as shown also in Fig. 5. The peptide derivatives consisting of 6 or 8 amino acids from N-terminus (M-N1 and M-N2, respectively) did not show antioxidative activity, whereas peptide derivatives from C-terminus (M-C1 and M-C2) maintained antioxidative activity. Interestingly, M-C2 derivative rather showed higher antioxidative activity than template peptide. This is maybe because the native structure of the peptide was altered and bioactive functional groups particularly within hydrophobic regions become exposed (Power et al., 2013). Further investigation is required in the future.
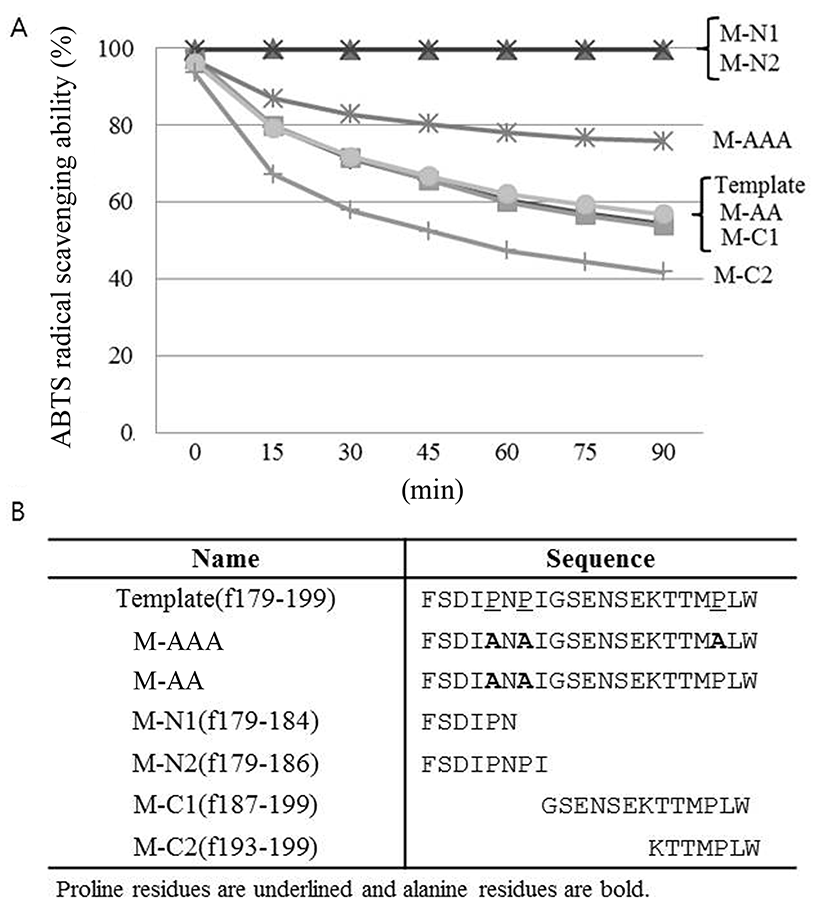
M-C2 peptide (f193-199) previously had been identified as an inhibitory peptide of angiotensin I-converting enzyme (ACE) (Hernandez-Ledesma et al., 2005). ACE-inhibitory and antioxidative activity could be related each other. Future studies will focus on the multi-functional activities of peptide antioxidants and investigate structure-function relationships.
Conclusion
Novel antioxidant peptide from fermented milk by L. lactis SL6 was identified, and it was identified as C-terminal fragment of bovine αs1-casein. Mutation experiments of the antioxidant peptide indicated proline residue at C-terminal region is important for its activity. Our findings suggest that fermentation of skim milk by L. lactis SL6 could have important applications in the food and pharmaceutical industries. Further studies to explore the capability of this peptide as a functional food ingredient will be of great interest in the future.