Introduction
For many centuries, humans have used horses for the purposes of travel, transport, entertainment, and as a source of food. Horse products are commercially available in various forms, including meat, meat powders and extracts. U.S. horse exports to the Mexican and Canadian markets increased by 148% and 660%, respectively, from 2006 to 2010. China produced 170,848 tons of horse meat to become one of the largest horse meat producers in the world (FAO, 2010). Jeju horses are native to the Jeju province in Korea. Although the population was nearly decimated, major animal husbandry and their designation as a Natural Monument have allowed the horse population in Jeju to increase. Although the native horses in Jeju are small, they have been improved for the production of meat and by-products by the Korean government by crossbreeding with thoroughbred horses (Jeju Native Horse × Thoroughbred). Some of these horses are used for racing or a food source (Lee et al., 2007).
Most nutritionally based studies of the horse have focused on horse meat, especially in terms of safety, storage stability, nutritional value, and meat quality (Lorenzo, 2014). Although horse meat is not particularly popular in Korea, horse bones have been consumed and are regarded as traditional foods and medicines for preventing bone diseases such as bone fracture and arthritis (Lee et al., 2007). The chemical composition of food materials, especially animal bone, has an important role in maintaining good health by supplying essential nutrients (Chalamaiah et al., 2012). Collagen is present in animal bone, skin, cartilage, blood vessels, and tendon. As a fibrous protein, it plays a key role in maintaining the structures of various organs and tissues. Collagen is extracted mainly from the bones and skin of livestock, which has been applied in cosmetics, foods, and medicines because of its good biological and functional properties (Li et al., 2009). The amino acid contents of all food proteins have significant roles in the physiological activities of the human body, and either directly or indirectly affects the maintenance of good health. The mineral contents of bone comprise mostly calcium and phosphorus. Calcium prevents osteoporosis by increasing bone formation and inhibiting bone loss (Reid and Ibbertson, 1986). Also, the bone marrow may positively affect the immune system because it helps in the transport of oxygen to the body cells. The marrow contains essential minerals such as P, Ca, and Fe, which are necessary for maintaining healthy bones (Kim el al., 2014). A recent study found that horse bone extracts affect longitudinal bone growth in adolescent male rats (Lee et al., 2009). Kim et al. (2014) suggested that antioxidation and anti-wrinkling effects of horse leg bone hydrolysates were observed in vitro. However, there is a lack of information about the nutritional composition of Jeju crossbred horse leg bone extracts (HLBE). Furthermore, the effects of the slaughter age on the nutrient content of HLBE are unknown. Therefore, the present work was conducted to evaluate the nutritional composition of HLBE from Jeju crossbred horses at 28, 32, and 38 mon of age.
Materials and Methods
Jeju crossbred horses (female, n=14) were raised with their mothers on a pasture and all animal experiments were carried out in accordance with the guidelines for the care and use of laboratory animals in National Institute of Animal Science of the Rural Development Administration (RDA) in Jeju, Korea. They were weaned after 6 mon and then fed ad libitum with a dried grass diet supplemented with 1.6% concentrate until 28, 32, or 38 mon. Horses were fed a rich basal diet (3.59 cal/g) composed of 17.12% crude protein, 6.30% crude fiber, 5.02% crude fat, 11.80% moisture, 0.26% Ca, 0.07% P, 0.06% Mg, 1.33% K, 0.03% Na, Cu (7.81 mg/kg), Fe (59.7 mg/kg), Mn (89.4 mg/kg), and Zn (27.8 mg/kg). The horses were selected and slaughtered according to their age (28 mon, n=4; 32 mon, n=5; 38 mon, n=5). The horses were stunned with a captive bolt, slaughtered, and dressed. The femur and tibia bones were obtained and kept in a freezer until analysis. Horse leg bone extracts (HLBE) and horse leg bone extracts powder (HLBP) were prepared as shown in Fig. 1. To clean the bones, the femur and tibia were soaked in water overnight at 4°C, and the water was discarded. The bones were subsequently boiled in fresh water for 30 min and the liquid and debris were discarded. The cleaned bones were then extracted three times in boiling water for 8 h each. The filtered, combined extracts were used for the proximate composition. The extracts were lyophilized to produce sample for fatty acid composition, amino acid content and mineral contents. Meanwhile the samples extracted 6 times (8 h each) were used as sample for the analysis of the change of the collagen content.
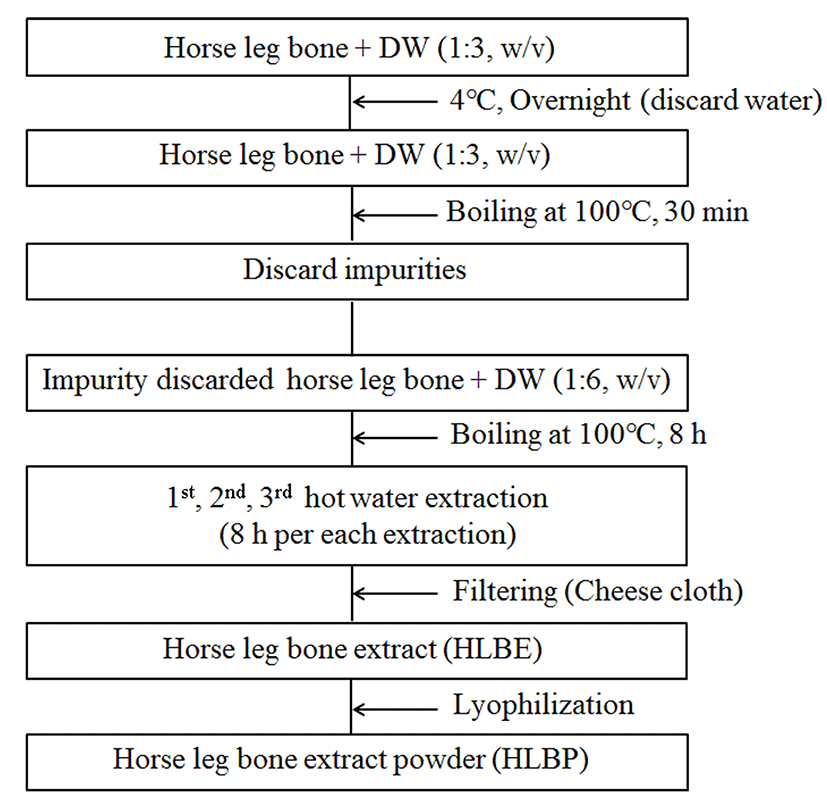
To determine the proximate composition of HLBE, the analyses were performed using AOAC methods (AOAC International, 1997). Crude protein was assessed using the Kjeldahl method (Method 726.08); crude fat was determined by solvent extraction (Method 991.36); and moisture was obtained by oven drying (Method 950.46). Crude ash, as the inorganic matter in the sample, was analyzed from dry ashing using furnace (Method 920.153).
The collagen content was estimated according to Kolar (1990). A collagen buffer solution (pH 6.0) was prepared, containing sodium acetate trihydrate (90 g), sodium hydroxide (15 g), citric acid monohydrate (30 g), and 1-propanol (290 mL) per liter of distilled water. The HLBE (4 g) was hydrolyzed in 30 mL of 7 N sulfuric acid at 105°C for 16 h. Then, the hydrolyzed sample (1 mL) was oxidized by mixing with a solution of chloramine-T (0.5 mL, 1.41% in collagen buffer solution. The mixture was incubated in the dark at room temperature for 20 min. Separately, a reactive color reagent was prepared by combining 4-dimethylaminobenzaldehyde (5 g) with 60% sulfuric acid (17.5 mL) and 2-propanol (32.5 mL). The solution from the oxidation reaction was blended the reactive color reagent (0.5 mL) and incubated in a constant-temperature water bath at 60°C for 15 min. Then, the absorbance of the hydroxyproline in the sample was measured at 558 nm using a SpectraMax M2e UV/Vis spectrophotometer (SpectraMax M2e, Molecular Devices, USA). A hydroxyproline standard curve was prepared for concentrations of 0.3, 0.6, 1.2, 2.4, and 4.8 μg/mL. The collagen content was calculated in samples according to the AOAC method using a correction factor of 8.
Lipids were extracted from the HLBE (1 g) using tert-butyl-4-hydroxyanisole (BHA, 20 μL) and Folch solution (15 mL, 2:1 v/v mixture of chloroform and methyl alcohol). After the sample was homogenized, the mixture was filtered through Whatman No.1 filter paper. The filtered sample was mixed with 3 mL of 0.88% KCl and put overnight in dark place. The bottom layer was evaporated using a stream of nitrogen gas. The sample was spiked with unesterified heptadecaenoic acid (10 g/mL) as an internal standard. The lipids were blended with 1 mL of 14% BF3 in a constant-temperature water bath at 90°C for 1 h, and then dissolved in 99% hexane. Chromatographic analysis was performed using a gas chromatography (Agilent 7890A, Agilent, USA), under the conditions given in Table 1.
Amino acid analysis was conducted according to the Aristoy and Toldra (1991)'s method with slight modification. The HLBE was finely ground to pass through a 0.5 mm mesh sieve. The powder was hydrolyzed in 6 N hydrochloric acid in a 110°C oven (VS-1202D3, Vision Scientific Co., Ltd., Korea) for 24 h. The decomposed samples were vacuum evaporated in a 55°C water bath (JSWB-22T, JS Research Inc., Korea). The samples were mess-up in a 25 mL volumetric flask using 0.2 N sodium citrate buffer (pH 2.2). After filtration through a 0.45 μm membrane filter, the sample was analyzed using a SYKAM S433 amino acid analyzer (SYKAM S433, SYKAM GmbH, Germany) with a lithium high-resolution PEEK column (LCAK06/Na, 4.6 mm × 150 mm).
The HLBE was diluted using an HCl according to the Microwave Pre-treatment protocol by US EPA (1999). After preparing a standard solutions of Mg, K, Ca, Mn, Cu, Zn, Se, Na, Fe, and P, the mineral content was determined by an inductively coupled plasma-optical emission spectrometry (ICP/OES, PTIMA 7300 DV, PerkinElmer, USA). The operating conditions are provided in Table 2. The mineral contents were obtained by multiplying the measured concentration by the dilution number.
Radial mode | |
---|---|
Plasma | 15 L/min |
Auxiliary | 0.2 L/min |
Nebulizer | 0.65 L/min |
RF Power | 1300 W |
Pump (Flow Rate) | 1.5 mL/min |
For the statistical analysis of all the data, one-way analysis of variance (ANOVA) calculations were performed using the General Linear Model (GLM) procedure in the SAS software package (ver. 9, SAS Institute Inc., USA) for all variables considered in the study. When treatment effects were significant (p<0.05), mean values were compared with Duncan’s multiple range test.
Results and Discussion
The proximate compositions of the HLBE from Jeju crossbred horses of different ages are shown in Table 3. Jonsson et al. (1985) reported that the water content of animal bones decreases with age. The moisture contents (92.75-93.05%) of the HLBE obtained from horses of different ages did not show any significant difference. The crude protein content was lowest in the HLBE at 28 mon (5.20%), but increased with age (p<0.05). The crude ash in the HLBE at 28, 32, and 38 mon ranged from 0.10 to 0.22%. Duerr and Earle (1974) reported that the crude ash content of a beef bone extract obtained after extraction for 60 min at 60°C water was 0.14-0.20%, which is similar to our results. Also, the crude ash of HLBE was significantly increased (p<0.05) with an increase in age.
a-cMean ± SE within same column with different superscript letters differ significantly at p<0.05.
The collagen contents of the HLBE from Jeju crossbred horse leg bones by age and extractions are given in Table 4. Collagen is the main component of bone, which is rich in connective tissue. Because of its excellent bioactivity, good biocompatibility, and lack of irritation in the body, collagen is used as an important active component of skin health and antioxidation (Kamara et al., 2009). The collagen content was highest in the first extraction (1.54-1.79 g/100 mL) for all age groups, but decreased with increasing numbers of extractions. Kim et al. (2002) reported the collagen contents of 33.20 and 33.16 mg/100 mL from the bones of Hanwoo (Korean Native Cattle) and Holstein cattle after extraction for 6 h. Another study showed that the total collagen content of the subchondral bone was not affected by training exercise, but, collagen density was increased in the cortical bone during growth (Jonsson et al., 1985). In this study, the collagen contents of the HLBE at 32 and 38 mon showed no significant difference, although these values were higher than that observed at 28 mon.
a,bMean±SE within same row with different superscript letters differ significantly at p<0.05.
A-FMean±SE within same column with different superscript letters differ significantly at p<0.05.
The fatty acid compositions of the HLBP from the Jeju crossbred horses at different ages are presented in Table 5. The saturated fatty acid (SFA) contents were higher than the unsaturated fatty acids (USFA), and the latter increased with age (p<0.05). The three most abundant fatty acids in the HLBP were palmitic acid (28.83-32.73%), oleic acid (30.09-32.23%), and linoleic acid (14.30-18.12%). In meat from this species, the three major fatty acids have been identified as oleic acid (30-35%), palmitic acid (28-31%), and linoleic acid (15-19%) (Seong et al., 2016). Thus, the fatty acid compositions of the leg bone extracts and meat from the Jeju crossbred horse are similar. The palmitic acid values in the HLBP were significantly decreased (p<0.05) as the horses aged. The palmitoleic acid (C16:1n7) contents of the HLBP at 28, 32, and 38 mon were 4.46, 4.62, and 5.82%, respectively, and HLBP at 38 mon showed the highest value (p<0.05). Oleic acid represents approximately 88% of the monounsaturated fatty acid. In its cis form, it decreases blood cholesterol levels, acts to maintain high density lipoprotein cholesterol and reduce low density lipoprotein cholesterol, and is related to the prevention of cardiac disease (Melton et al., 1982). Oleic and palmitoleic acids have been shown to improve the skin’s innate antimicrobial defense system (Ní Raghallaigh et al., 2012). Palmitoleic acid is the most active antimicrobial fatty acid component of human sebum (Wille and Kydonieus, 2003). The concentration of palmitoleic acid (C16:1n7) in horse subcutaneous adipose tissue (7.85% total detected fatty acid) was higher than in beef cattle (Japanese black, 4.85%; Holstein steer, 5.56%; pigs, 1.73%) (He et al., 2005). The HLBP at 38 months has the highest essential fatty acids, C18:2n6, C18:3n6, and C18:3n3. However, the C20:4n6 contents were higher (p<0.05) in the HLBP at 28 (0.51%) and 32 mon (0.50%) than in those at 38 mon (0.33%).
a,bMean±SE within same row with different superscript letters differ significantly at p<0.05.
SFA, Saturated fatty acid; MUFA, Monounsaturated fatty acid; PUFA, Polyunsaturated fatty acid.
The amino acid contents of the HLBP from Jeju crossbred horses of different ages are presented in Table 6. The HLBP were rich in glycine (13.17-17.40%), proline (6.99-8.55%), glutamic acid (6.70-8.53%), alanine (4.96-6.35%), and arginine (4.62-5.86%) at all ages. Golan and Jelen (1979) noted that the amino acid contents of low-temperature alkaline extracts from beef bones were abundant in glycine, glutamic acid, alanine, and arginine. Also, Hanwoo shank bones exhibited higher contents of glycine (23%), glutamic acid (13%), and alanine (13%) (Park et al., 1986).
a-cMean ± SE within same row with different superscript letters differ significantly at p<0.05.
The HLBP showed a similar amino acid composition to that of the collagen polypeptide from yak bone (Li et al., 2009), which is rich in glycine, alanine, proline, hydroxy-proline, and glutamic acid, but low in tyrosine. In this study, we found that the amino acid content of the HLBP from horse leg bone is also similar to that of cattle (Hanwoo, Holstein, and Australian beef) (Park et al., 1994). Glycine and proline are the major components of collagen, and proline elicits stress-stimulated phenolic biosynthesis and stimulates antioxidant enzyme response pathways (Shetty, 2004). The amino acid contents of the HLBP at 38 mon is higher than in that at 28 mon and this may be due to the higher contents of crude protein of it. The HLBP at 38 mon showed the highest contents of essential amino acids such as threonine, valine, isoleucine, leucine, and lysine (1.44, 2.47, 1.05, 2.71, and 2.98%). Also, two aromatic amino acids, tyrosine and phenylalanine, are known to act positively as direct radical scavengers (Chalamaiah et al., 2012). The tyrosine and phenylalanine levels in the HLBP at 32 and 38 mon were increased significantly compared to those at 28 mon (p<0.05). Arginine is included in the essential amino acids because it is considered a conditionally essential amino acid. The arginine values in the HLBP were significantly increased (p<0.05) with the age of the animal. Amino acids are essential for the synthesis of a wide variety of proteins with important functions including carriers of oxygen, CO2, vitamins, and structural proteins (Chalamaiah et al., 2012). Above 32 mon, the HLBP appear to be important sources of high biological value proteins due to their essential amino acids content.
The mineral contents of the HLBP from the Jeju cross-bred horses at different ages are presented in Table 7. The Ca content was unaffected by age. Na and K play indispensable roles in the electrical conductivity of the brain and facilitate the improvement of brain function (Syad et al., 2013). K is also essential for the homeostatic balance of body fluids (FAO, 2009). Na, as the predominant element, is present to the largest extent (p<0.05) in the 38 mon HLBP (736.60 mg/mL) and has the lowest value at 28 mon (558.54 mg/mL). The K content of the HLBP was significantly increased (p<0.05) with age. The HLBP at 38 mon also contains higher amounts of Zn, Se, P, and Fe, and thus, may contribute significantly to the daily intake of these essential minerals (Cabrera et al., 2010). Most minerals of HLBP were increased with an increase in age, and it was similar to the result of crude ash. In the HLBP obtained from 38 mon old horses, the Ca and P contents were 49.92 and 92.35 mg/mL respectively, whereas the Ca/P ratios were approximately 1:1 in the HLBP of 28 and 32 mon. When the P consumption is higher than the Ca content, Ca absorption is inhibited and bone loss is increased (Hsu and Davis, 1981). To optimize the absorption of Ca, an 1:1 consumption ratio of Ca/P is recommended (Kwon et al., 2013). Kim et al. (2002) and Ha et al. (2008) reported the Ca contents of Hanwoo and Holstein cattle, as well as kangaroo tail, as 13.79 mg/L, 14.01 mg/L, and 0.68 mg/100 g, respectively. The mineral contents of bone have been reported to increase during maturation, and this process can be modified by physical exercise (Van Weeren et al., 2000). In this study, the horses were older and exercise significantly more than these other animals. It assumed that the high mineral content of the horse bone is due to both physical exercise and maturity.
a,bMean±SE within the same row with different superscript letters differ significantly at p<0.05.
Conclusions
Horse bones are valuable as an alternative animal food source. Most Asians consume animal bones for nourishment. However, horse bones or horse bone extracts distributed in the market are often underestimated in terms of nutritional quality, and their origin, composition, age, and/or sex have been misrepresented. Despite the prior lack of nutritional information for Jeju crossbred horses, our results show that bone extracts from horses at 38 mon of age are more nutritious than those from 28 and 32 mon-old horses, since they contain significantly more nutrients due to their high levels of palmitoleic acid, essential amino acids, minerals, and collagen. Further in vivo tests are needed to evaluate the bioavailability of these nutrients.