Introduction
Skeletal muscles, accounting for more than 40% of human body, conduct several crucial functions such as controlling movement and storing energy (Lee et al., 2018; Lee et al., 2021; Shin et al., 2020). With ageing, skeletal muscles gradually decline, which results in functional declines such as falls, frailty, and decline in basal metabolic rate (Cruz-Jentoft and Sayer, 2019). These functional declines restrict physical activities, which causes more muscle atrophy and leads to increased hospitalization, morality, exacerbation of metabolic diseases, and reduced quality of life (Shin et al., 2020). Because of increasing disorders and morality caused by muscle atrophy, medical expenses may increase, and national economy may be affected. Statistics Korea (KOSTAT, 2021) has suggested that the proportion of elderly population could rise by 2.1-fold in 2040, reaching over 17 million. As the number of elderly persons continues to increase, muscle atrophy has gained much importance in recent years.
Sarcopenia occurs owing to biological processes such as elevated levels of proinflammatory cytokines and oxidative stress (Lee et al., 2021). Oxidative stress and intracellular damage occur by redox imbalance owing to the accumulation of reactive oxygen species (ROS) throughout the lifespan and can lead to mutations in mitochondrial DNA, thereby impairing mitochondrial function and causing cellular damage (Bak et al., 2019). Furthermore, altered mitochondrial functions are associated with increased secretion of proinflammatory cytokines (López-Armada et al., 2013), such as interleukin (IL)-6 and tumor necrosis factor (TNF)-α, which induce muscle wasting by impairing maturation and regeneration of muscle cells (Späte and Schulze, 2004). Lipopolysaccharide (LPS), a constituent of the cell wall of gram-negative bacteria, is commonly used to induce oxidative stress and inflammatory responses (Kurhaluk et al., 2018; Pasqualetti et al., 2014). Additionally, various cell and animal-based studies have employed glucocorticoid injection to induce oxidative stress. Long-term use of dexamethasone (DEX), a glucocorticoid medication, causes proteolysis and oxidative stress (Kim et al., 2021). DEX upregulates the genes associated with the ubiquitin proteasomal system through the Forkhead box O (FoxO) pathway and decreases protein synthesis by inhibiting the protein kinase B (AKT)/mechanistic target of rapamycin (mTOR) pathway and degrading myogenin (Hasselgren et al., 2010; Kim et al., 2021).
Insufficient daily protein intake accelerates loss of muscle mass (Wall et al., 2013) and it has been observed that more than 50% individuals aged ≥60 consume inadequate amount of protein in Republic of Korea (Kwon et al., 2019). In this context, horse meat has gained attention as a healthy food owing to its high contents of protein and mineral, despite being low in fat (Lee et al., 2018; Manning and Cantley, 2007). Historically, horses have served roles in transport, recreation as well as for meat and milk production. Presently, horsemeat remains a dietary choice in Central Asia and in some European countries such as Iceland, Belgium and Italy (Balji et al., 2020). In recent decades, consumer perceptions of horse meat have become increasingly positive not only in Finland but also in Korea (Jeon et al., 2015; Leipämaa-Leskinen et al., 2018). In general horses are gaining recognition as an alternative animal protein source due to their nutritional value (Bò et al., 2013). Notably, previous studies by Kim et al. (2013) have observed the antioxidative potential of hydrolysate of horse leg bone, while Kim et al. (2018) have reported hide gelatin hydrolysate of donkey as an antiphotoaging agent. However, the efficacy of horse meat in alleviating muscle atrophy has not yet been investigated.
Bioresources hydrolyzed with food-grade enzymes, which can alleviate muscle atrophy have been reported. Pacific oyster (Crassostrea gigas) hydrolyzed with protamex and neutrase (Jeon and Choung, 2021), soluble whey protein hydrolyzed with Alcalase and protamex (Shin et al., 2020) and potato peptide hydrolyzed with Alcalase (Chen et al., 2021) significantly inhibit muscle atrophy by facilitating the PI3K/AKT/mTOR pathway and suppressing FoxO activation. Through enzymatic hydrolysis, proteins are converted into peptides, which can change the functional characteristics of proteins and improve their quality (Liceaga‐Gesualdo and Li‐Chan, 1999). In addition, protein hydrolysates are absorbed more rapidly and easily than intact proteins. In particular, protein hydrolysates of 1.0–5.0 kDa have broad dietary applications for their high nutrient-related and medicinal properties (Bhaskar et al., 2007; Steffl et al., 2017).
Based on these observations, this study aims to evaluate the potential of horse meat hydrolysates to inhibit the production of ROS, proinflammatory cytokines, and atrophy related biomarkers in DEX-treated C2C12 cells.
Materials and Methods
The hydrolysate was prepared using m. biceps femoris of horse meat. To improve functional characteristics of proteins, hydrolysis was performed using three food-grade enzymes under specific conditions as follows: Alcalase (pH 8.0, 50°C), papain (pH 8.0, 37°C), and Protamex (pH 6.0, 50°C). Horse meat was combined with distilled water at ratio of 1:5; and 1% enzyme diluted with distilled water was added; and hydrolysis was carried out for 4 or 8 h. After hydrolysis, the protease was inactivated by raising the temperature to approximately 100°C for 10 min. Hydrolysates were filtered through Amicon Ultra centrifugation filters (Merck Millipore, Billerica, MA, USA) to separate fragments <3 kDa. The supernatants were freeze-dried and stored at –20°C. The overall procedure is shown in Fig. 1.
C2C12, mouse skeletal myoblast line (CRL-1772, ATCC, Manassas, VA, USA), was cultured in Dulbecco’s Modified Eagle Medium (DMEM) supplemented with 10% fetal bovine serum (WELGENE, Daegu, Korea) and 1% penicillin/ streptomycin (Biowest, Nuaillé, France) at 37°C under 5% CO2 within an incubator. To induce myogenic differentiation, the culture medium was substituted with DMEM with 2% horse serum (Thermo Fisher Scientific, Waltham, MA, USA) for 5 d. Media was changed at 48-h intervals.
Cell viability was assessed using an 3-(4,5-dimethylthiazol-2-yl)-2,5-diphenyltetrazolium bromide (MTT) assay. C2C12 myoblast cells were seeded in 24-well plate (2×105 cells/well) and incubated overnight at 37°C under 5% CO2 within an incubator. To evaluate cytotoxicity of low molecular-weight horse-meat hydrolysates, C2C12 myoblast cells were exposed to different concentrations (10, 50, 100, 200, 300, 500, 1,000 μg/mL) of six hydrolysates (A4<3kDa, A8<3kDa, M4<3kDa, M8<3kDa, N4<3kDa, and N8<3kDa) for 24 h. To assess the effect of H2O2-induced oxidative stress, C2C12 myoblast cells were seeded in a 24-well plate (4×105 cells/well) and incubated overnight under same conditions. Cells were exposed to different concentration of hydrolysates (10, 50, 100, and 200 μg/mL) for 1 h, and 1 mM H2O2 was added to each well and incubated for 6 h. Cells were then incubated with 5 mg/mL MTT (Sigma-Aldrich, St. Louis, MO, USA) for 4 h. The culture medium was discarded, and formazan crystals were dissolved in dimethyl sulfoxide. Finally, the absorbance at 540 nm was measured using a UV spectrophotometer (SpectraMax M2e, Molecular Devices, San Jose, CA, USA).
Cellular ROS was measured using the fluorescent dye 2’, 7’-dichlorofluorescin diacetate (DCFDH-DA, Sigma-Aldrich). Briefly, C2C12 myoblasts were seeded in a 96-well black plate (2.5×105 cells/well) and cultured overnight in DMEM as described above. Cells were rinsed twice with phosphate-buffered saline (PBS) and pretreated with the hydrolysates for 1 h, followed by treatment with 25 ng/mL LPS for 4 h. After the media was removed, cells were stained with 10 μM DCFDH-DA for 30 min at 37°C in dark. Cells were washed with PBS, and immediately analyzed using a spectrofluorometer (Spectramax M2e, Molecular Devices) at excitation and emission wavelengths of 485 and 535 nm, respectively.
C2C12 myoblasts were incubated in a 6-well plate (3×106 cells/well), and differentiation was induced. C2C12 myotubes formed after 5 d were pretreated with the 1% v/v of hydrolysates for 1 h, followed by treatment with 100 μM of DEX (Sigma-Aldrich) for 24 h to mimic the conditions of muscle atrophy in vitro. Cells were washed twice with cold PBS and lysed using RIPA buffer (ATTO, Tokyo, Japan) containing freshly added protease and phosphatase inhibitors (ATTO). Whole cell lysates were centrifuged (4°C, 10,000×g for 20 min), and supernatants were collected. Protein concentration was determined by using a BCA protein assay kit (Thermo Fisher Scientific, Rockford, IL, USA). Proteins (30 μg) were mixed with 4× Laemmli sample buffer (GenDEPOT, Barker, TX, USA) and separated by 6%–10% sodium dodecyl sulfate–polyacrylamide gel electrophoresis. Separated proteins were transferred to polyvinylidene difluoride membranes using a semi-dry electrophoretic transfer system (ATTO). The membranes were blocked with 5% bovine serum albumin (Bovogen, Keilor East, Australia) at room temperature at least 1 h, followed by incubation with primary antibodies against AKT (1:2000; Cell Signaling Technology, Danvers, MA, USA), FoxO3a (1:1,000; Cell Signaling Technology), mTOR (1:1,000; Cell Signaling Technology), p-AKT (1:1,000; Cell Signaling Technology), p-FoxO3a (1:1,000; Cell Signaling Technology), p-mTOR (1:1,000; Cell Signaling Technology), myogenin (1:200; Santa Cruz Biotechnology, Dallas, TX, USA), and β-actin (1:200; Santa Cruz Biotechnology) overnight at 4°C. Each membrane was washed thrice with 0.05% TBST (25 mM Tris, 0.05% Tween 20, and 140 mM NaCl) and incubated with anti-mouse or anti-rabbit IgG antibodies at room temperature. Protein bands were visualized through enhanced chemiluminescence (Cyanagen, Bologna, Italy) detection, and densities of the bands were analyzed using Fusion Solo 6S Edge (Vilber-Lourmat, Marne la Vallée, France).
C2C12 myoblasts were incubated in a 6-well plate (3×106 cells/well), and differentiation was induced. To analyze the expression of proinflammatory cytokines (TNF-α and IL-6), C2C12 myoblasts were treated with 0.25 μg/mL of LPS. C2C12 myotubes wer analyzed for expression of two ubiquitin-proteasomal markers [Atrogin-1 and muscle RING finger protein-1 (MuRF-1)]. Following differentiation for 5 d, C2C12 myotubes were pretreated with horse meat hydrolysates for 1 h, and then cells were treated with 100 μM DEX for 24 h to mimic the conditions of muscle atrophy. Total RNA was extracted using a RNeasy Mini kit (Qiagen, Hilden, Germany) according to the manufacturer’s instructions. Pre-Mix (dT18 Plus; BioFact, Daejeon, Korea) was used to synthesize cDNA. Each cDNA was amplified on a LightCycler 96 System (Roche Diagnostics, Basel, Switzerland) using 2X Real-Time PCR Master Mix (BioFact). Glyceraldehyde-3-phosphate dehydrogenase (GAPDH) was used as a reference gene to normalize mRNA levels. The primers used for amplification and corresponding annealing temperature values are provided in Table 1.
All data were assessed by analysis of variance (ANOVA) using Statistical Analysis System (SAS) program v.9.4. Each experiment was conducted in triplicate, obtaining three independent data points for each condition. The results are presented as means±SD of these replicates. Statistical significance was determined at p<0.05 using Tukey’s test.
Results and Discussion
To evaluate the effects of six horse meat hydrolysates (A4<3kDa, M4<3kDa, N4<3kDa, A8<3kDa, M8<3kDa, N8<3kDa) on C2C12 myoblasts, we examined cell viability of the cells. A8<3kDa (Fig. 2B), M4<3kDa (Fig. 2C), M8<3kDa (Fig. 2D), and N8<3kDa (Fig. 2F) had no significant impact on cell viability. A4<3kDa at 50 μg/mL concentration significantly increased viability of C2C12 myoblasts by up to 33.7% compared to that of the control cells (p<0.05, Fig. 2A). Although cell survival decreased by N4<3kDa treatment (Fig. 2E) at all concentrations, it was above the threshold for safety as defined by the guidelines of the Korea Ministry of Food and Drug Safety (MFDS) that states that cell viability >70% is considered safe. These findings demonstrate that the six horse meat hydrolysates do not have a cytotoxic effect on C2C12 myoblasts.
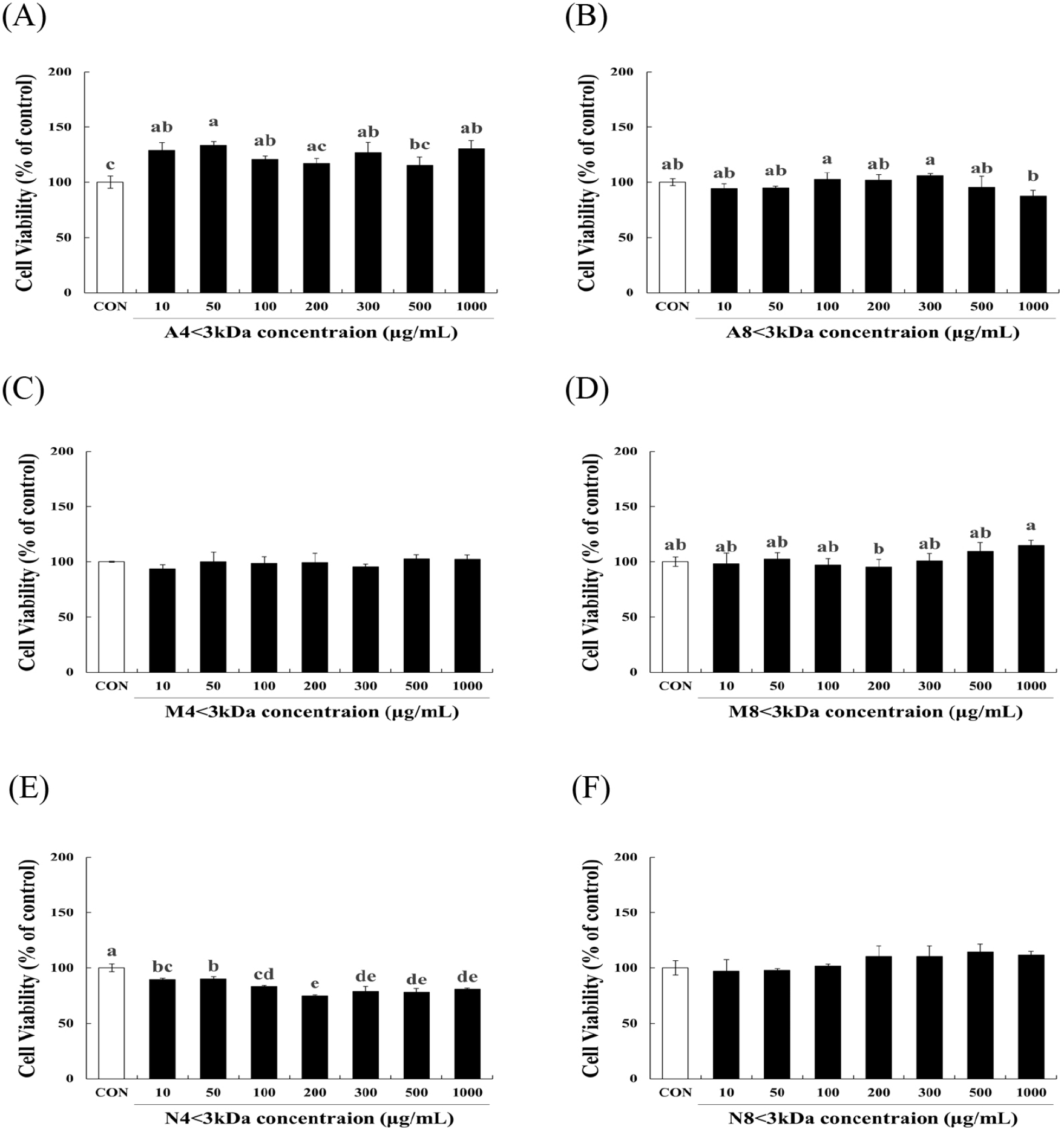
H2O2 treatment significantly decreased the viability of C2C12 myoblasts (p<0.05, Fig. 3). The viability of H2O2-treated cells increased by 34.86%–55.55% when treated with 10–200 μg/mL A4<3kDa (Fig. 3A), while 100 and 200 μg/mL M8<3kDa increased the viability of H2O2-treated cells by 10.12% and 10.33%, respectively (Fig. 3D).
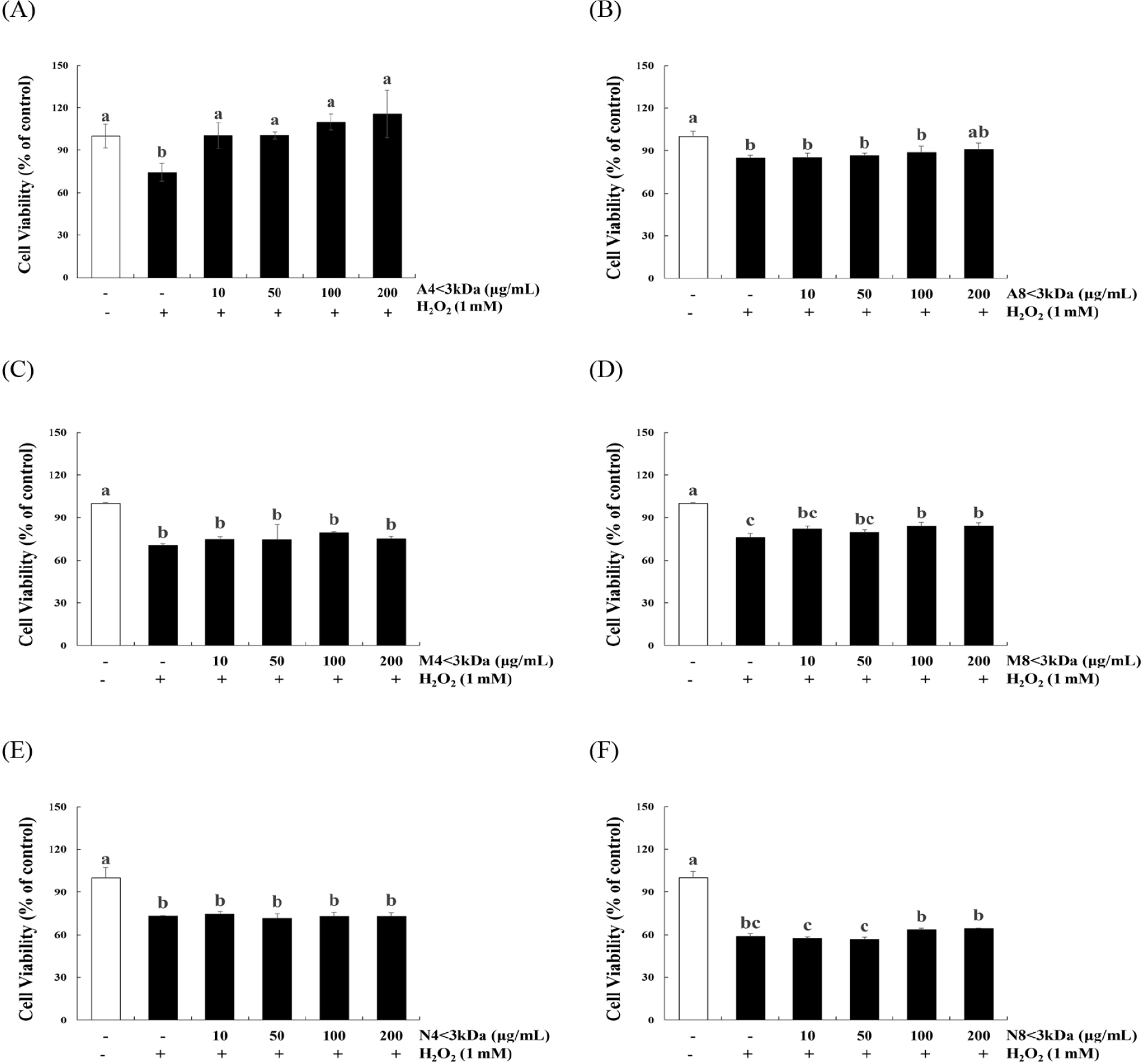
Lee et al. (2023) have previously reported that two types of hydrolysates of the edible insect Protaetia brevitarsis (100 μg/mL of myofibril protein and 200 μg/mL of sarcoplasmic protein hydrolysates) restore the viability of H2O2-treated C2C12 cells by approximately 73.28% and 76.38%, respectively; these hydrolysates are mainly composed of 150-, 75-, 37-, and 10-kDa peptides. Sivakumar et al. (2014) reported that 50.4 μM of a 1202.56-Da peptide from bovine muscle increases the viability of H2O2-treated C2C12 cells by approximately two times. However, A8<3kDa (Fig. 3B), M4<3kDa (Fig. 3C), N4<3kDa (Fig. 3E), and N8<3kD (Fig. 3F) did not affect the viability of H2O2-treated cells. Consequently, A4<3kDa that increased the viability of H2O2-treated cells more efficiently than did the other hydrolysates was selected for further analysis.
LPS induces an acute inflammatory state by releasing proinflammatory mediators and ROS, a known factor implicated in oxidative stress (Lee et al., 2021; Qiao et al., 2011). As oxidative stress is a crucial cause of age-relate muscle atrophy, potential of A4<3kDa in various doses (10–200 μg/mL) was evaluated in alleviating LPS-induced oxidative stress.
Intracellular ROS content of C2C12 cells exposed to LPS increased by 2.08-fold compared to that of the control group, which was significantly decreased by 10–100 μg/mL A4<3kDa treatment and reached the level of control group (Fig. 4). Notably, cells treated with 200 μg/mL A4<3kDa indicated significantly low intracellular ROS production compared to that of the control group, showing a 58.92% decrease in comparison to that of cells treated with LPS alone (p<0.05, Fig. 4). This antioxidant effect exhibited by the sample can be attributed to the increased cell viability of C2C12 cells in our study. Oxidative stress often characterized by an imbalance between ROS production and antioxidant defenses, plays a role in cellular damage and compromised viability (Schieber and Chandel, 2014). The A4<3kDa effectively scavenged ROS, mitigating oxidative stress.
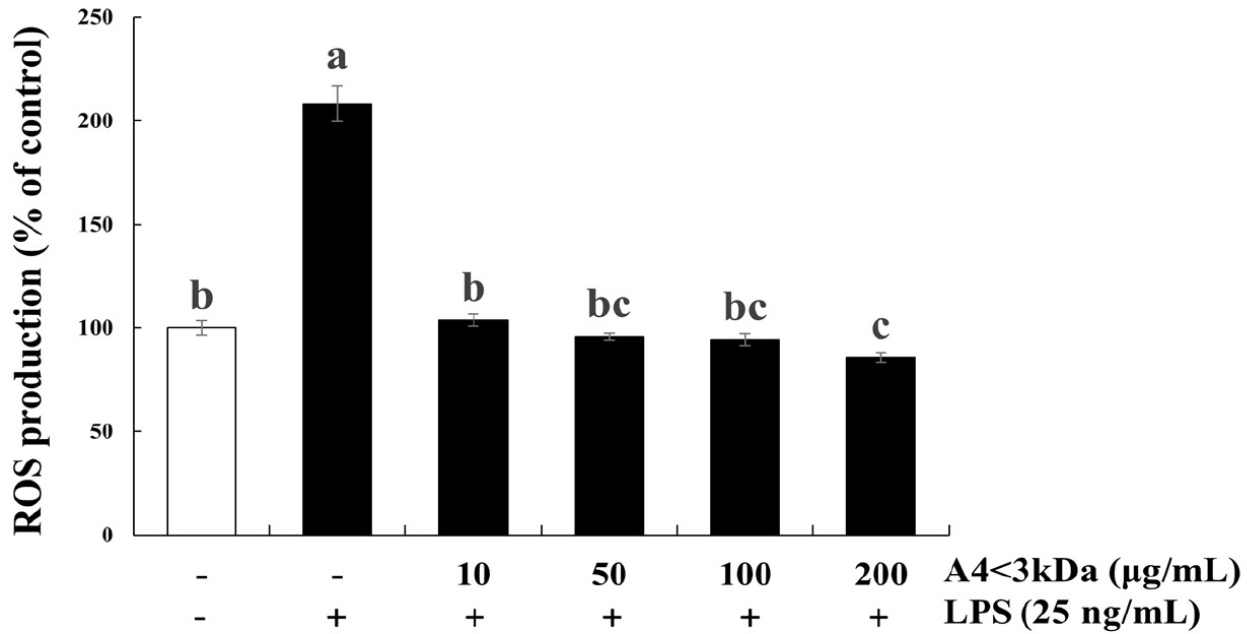
Impairment of mitochondria by oxidative stress can contribute to inflammatory processes by producing the proinflammatory cytokines IL-6 and TNF-α (Fan et al., 2016). IL-6 and TNF-α inhibit muscle synthesis. In aged individuals, small muscle area and less appendicular muscle mass indicate high concentration of TNF-α and IL-6, which inhibit the expression of insulin-like growth factor-1 (IGF-1) in plasma (Thoma and Lightfoot, 2018).
Treatment of C2C12 myoblasts with LPS significantly increased the expression of IL-6 (Fig. 5A) and TNF-α (Fig. 5B) compared to that of the control group (p<0.05); however, IL-6 expression decreased up to 24.07% by A4<3kDa treatment at 10–200 μg/mL, and TNF-α expression decreased by 50.85% with 200 μg/mL A4<3kDa. This anti-inflammatory property of A4<3kDa also likely contributed to the observed increased cell viability. Inflammation-induced cellular damage can lead to reduced viability and impaired cellular functions (Schieber and Chandel, 2014). The sample’s ability to suppress the expression of proinflammatory cytokines, such as IL-6 and TNF-α, underscores its potential in alleviating inflammation-associated cell damage.
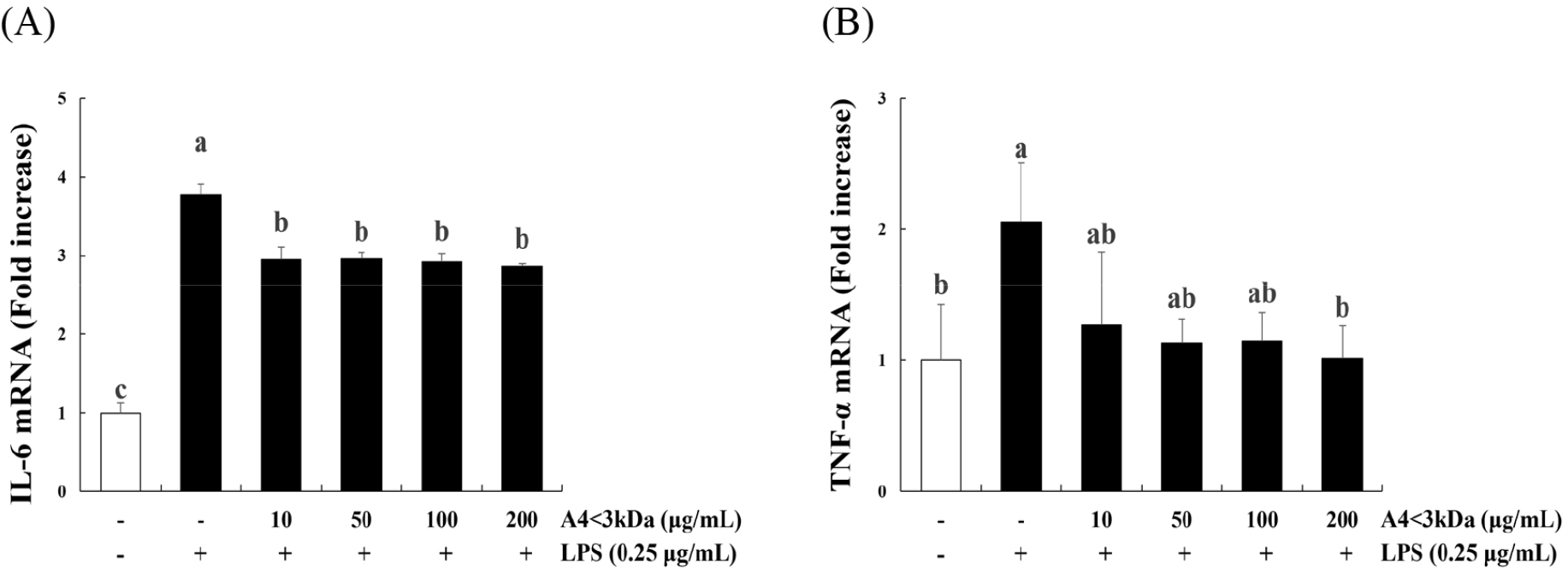
Proinflammatory cytokines at elevated levels contribute to the deterioration of skeletal muscles by upregulating proteins involved in muscle breakdown, including E3 ligase. Also, proinflammatory cytokines hinder protein synthesis by promoting FoxO activation and suppressing AKT activation, a critical aspect of protein metabolism (Sharma and Dabur, 2020).
DEX decreases proliferation of muscle cells and protein synthesis while increases protein breakdown (Desler et al., 1996). The proteolytic action of DEX is mediated through the activation of the ubiquitin-proteasomal and lysosomal degradation pathways (Schakman et al., 2013). Fig. 6 presents an overview of the signaling pathways of glucocorticoid-induced ubiquitin-proteasome system. These catabolic systems are associated with enhanced expression of Atrogenes, the genes associated with atrophy, such as FoxO, Atrogin-1, and MuRF-1 (Schakman et al., 2013). These ubiquitin-proteasomal systems degrade myogenic transcription factors, such as myogenin, which are associated with muscle development (Hasselgren et al., 2010), and inhibit the IGF-1 signaling pathway, an anabolic growth factor including AKT and mTOR (Lee et al., 2019; Xie et al., 2018).
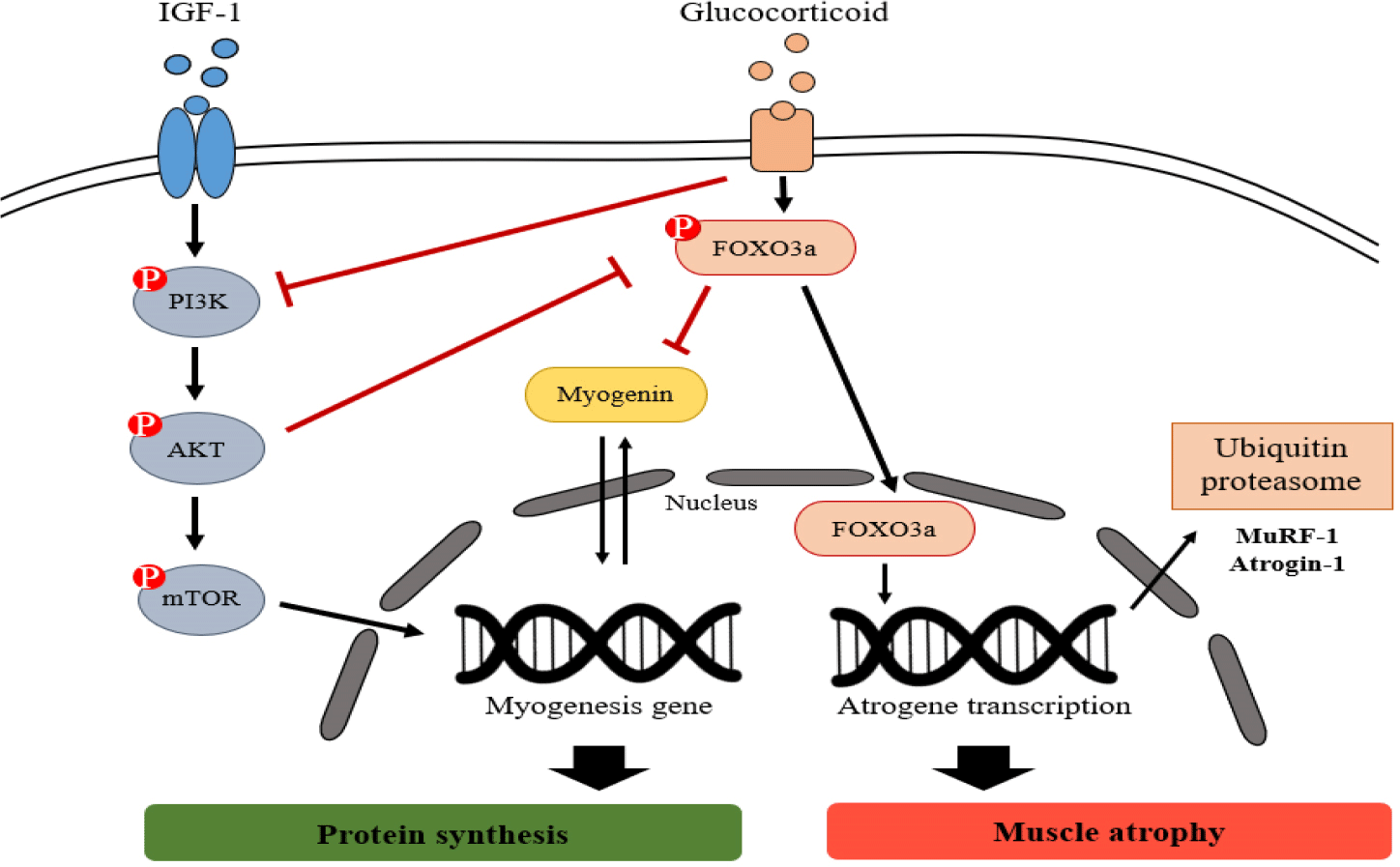
To identify the effect of A4<3kDa on proteins involved in DEX-induced muscle atrophy, the phosphorylation of mTOR, FoxO3a, and AKT and expression of myogenin were assessed. The phosphorylation of AKT (Fig. 7A), FoxO3a (Fig. 7B), and mTOR (Fig. 7C) and expression of myogenin (Fig. 7D) significantly decreased in the DEX-treated group in comparison to those of the control group (p<0.05). The phosphorylation of AKT, FoxO3a, and mTOR was significantly increased by up to 34.49%, 54.09%, and 60.18% by treating with A4<3kDa at doses of 1,000, 100, and 50 μg/mL, respectively (p<0.05). In addition, 100 μg/mL A4<3kDa significantly enhanced myogenin expression by up to 24.51% (p<0.05).
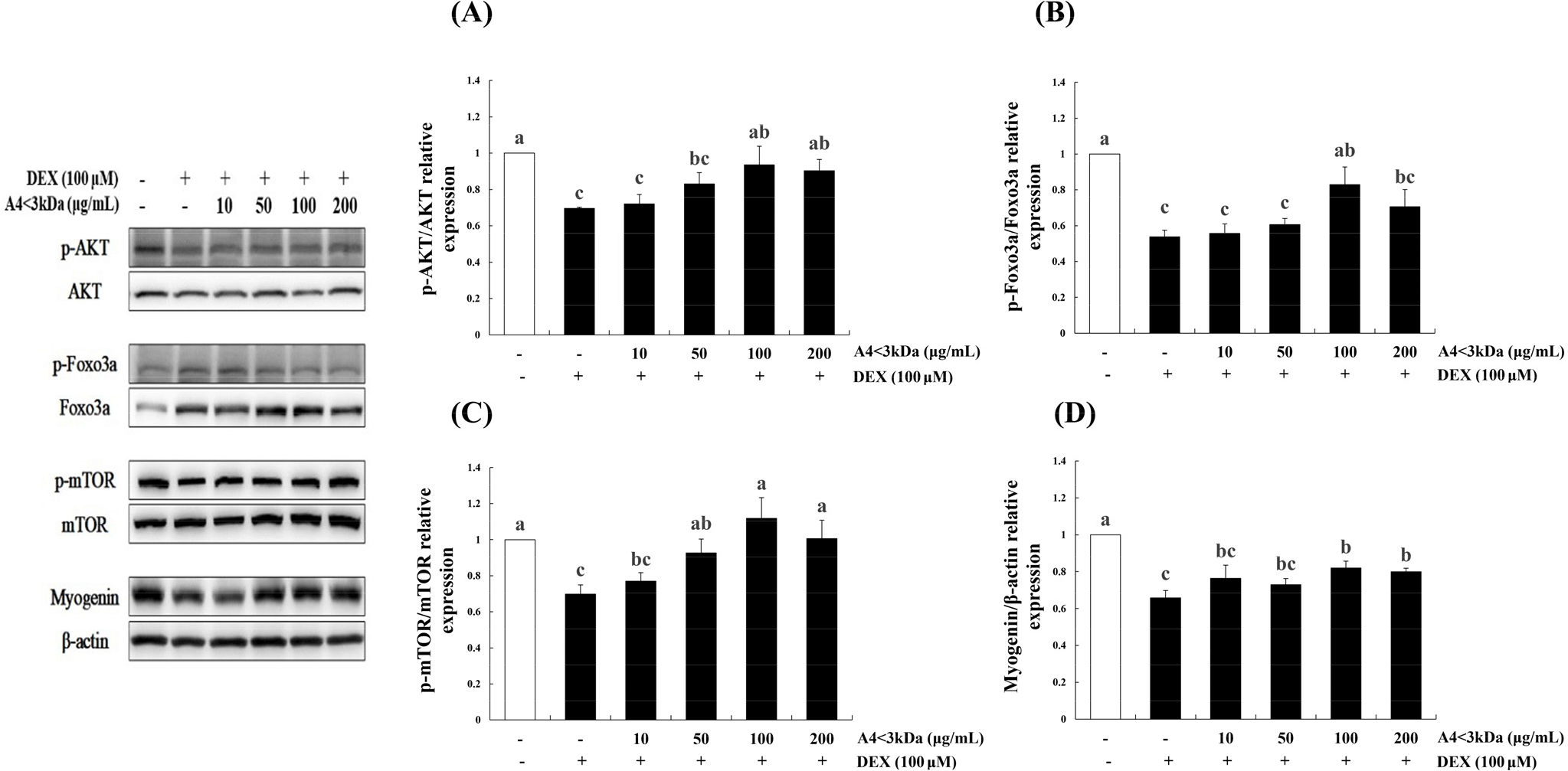
Jo et al. (2021) also observed that hydrolyzed deer antler extract with Alcalase decreased FoxO3a expression by approximately 76% (50 μg/mL extract) and 80% (200 μg/mL extract) and increased myogenin expression by 2-folds (50 μg/mL extract) and 2.5-folds (200 μg/mL extract) in C2C12 cells treated with 5-aminoimidazole-4-carboxamide ribonucleoside. Similar to other food-grade enzymatic hydrolysate, A4<3kDa increased the phosphorylation levels of AKT, FoxO3a, and mTOR and myogenin expression.
Horse meat has been found to contain notably higher levels of essential amino acids compared to pork (Jeon et al., 2015). Specially, branched-chain amino acids (BCAAs) like valine, leucine, and isoleucine, which are essential for regulating body protein synthesis (Holeček, 2002) are present in significant amounts. Previous research has highlighted the specific benefits of leucine, which is known to enhance myofibrillar protein content by upregulating the expression of key components in the AKT/mTOR pathway, including mTOR, 4E-BP1, and S6K1 in C2C12 cells (Haegens et al., 2012). Our findings further suggest that BCAAs, particularly leucin, could potentially contribute to the improved expression of mTOR.
Since FoxO3a activation causes atrophy of skeletal muscles by increasing transcription of ubiquitin ligases (Zhao et al., 2008), we further analyzed the expression of Atrogin-1 and MuRF-1, regulators of ubiquitin-mediated proteins related to muscle atrophy.
To assess the effect of A4<3kDa on DEX-treated C2C12 myotubes, we measured the mRNA levels of Atrogin-1 (Fig. 8A) and MuRF-1 (Fig. 8B), the muscle atrophy-associated ubiquitin ligases. Treatment of C2C12 myotubes with 100 μM DEX for 24 h elevated the mRNA level of Atrogin-1 (by 6.2-folds) and MuRF-1 (by 2.3-folds) compared to those of the control group (p<0.05). Treatment with 50 μg/mL A4<3kDa significantly decreased the mRNA expression of Atrogin-1 and MuRF-1 by up to 22.22% and 28.78%, respectively (p<0.05).
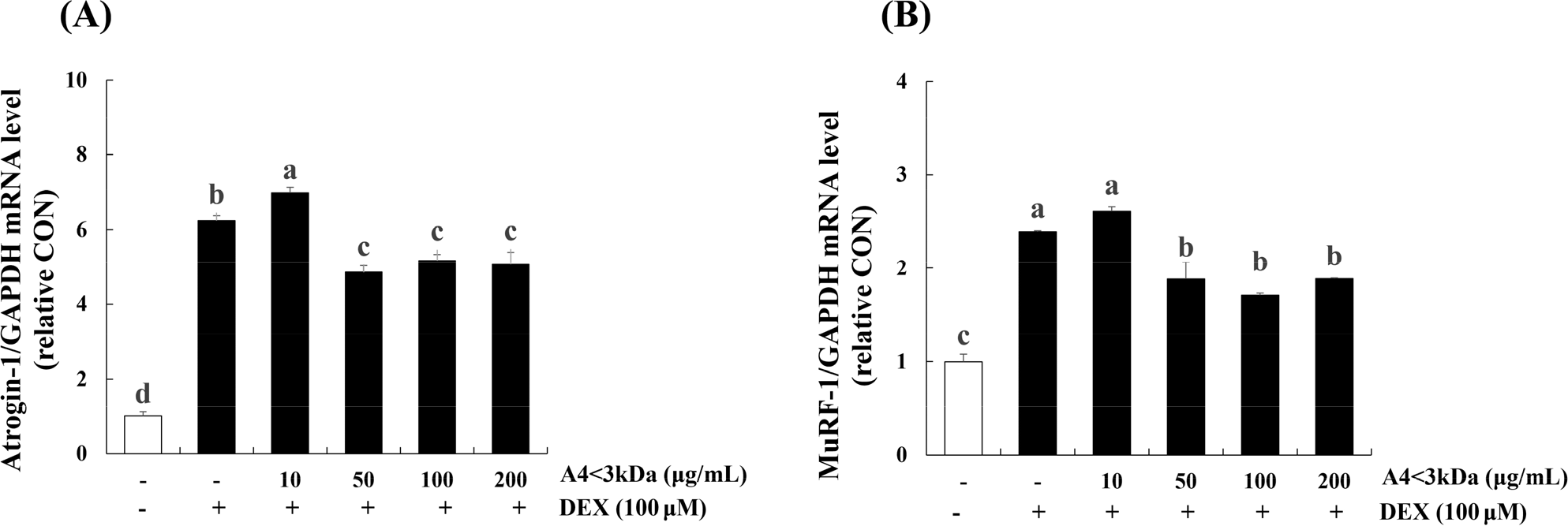
Jo et al. (2021) showed that hydrolyzed deer antler extract with Alcalase decreased MuRF-1 expression by approximately 5% (200 μg/mL of extract) in 5-aminoimidazole-4-carboxamide ribonucleoside-inflicted C2C12 cells. Kang et al. (2023) reported that hot water extract of goat meat (forelegs, hindleg, loin and ribs) caused lower Atrogin-1 expression level (approximately 66.67%, 33.33%, 53.33%, 60% respectively) and MuRF-1 expression level (about 28.57%, 33.33%, 28.57%, 28.57% respectively) in DEX-treated C2C12 cells. Also, they explored the potential cause behind the reduced expression of two ubiquitin-proteasome pathway components, attributing it to the sufficient leucine content in goat meat. Notably, other previous research indicates that leucine supplementation in rats led to a down-regulated mRNA expression of MuRF-1 (Wan et al., 2017) and treating C2C12 with leucine inhibited the mRNA levels of Atrogin-1 (Herningtyas et al., 2008). This suggests that the presence of leucin in horse meat might potentially prevent expression of ubiquitin-proteasomes induced by DEX.
In accordance with these studies involving hydrolysates of natural origin, A4<3kDa inhibited the upregulation of ubiquitin-proteasomal system and degradation of myogenic transcription factors while elevated muscle protein synthesis through regulating the AKT/mTOR/FoxO signaling pathway in a DEX-treated in vitro model. Our data provide molecular evidence for the protective efficacy of A4<3kDa against DEX-induced oxidative stress and muscle atrophy. Our findings indicate that A4<3kDa has the potential to prevent sarcopenia; however, additional in vivo studies are required to validate its effectiveness in promoting muscle health.