Introduction
There is no specific definition in poultry meat quality. Poultry meat quality is difficult to define because it is a complex concept determined by consumer preferences. The most important factor in determining poultry meat quality is not only physical and chemical properties but also a degree of preference of consumers towards meat products. In general, there is lack of information and common standard in identifying poultry meat quality, because meat quality depends on several quality traits and their complex effects. At the view point of consumer, meat quality traits can be classified into three: i) appearance quality traits (AQT) such as meat color, drip or purge loss and texture of meat (Joo et al., 2013; Tilman, 2000); ii) eating quality traits (EQT) such as tenderness, flavor, juiciness, and succulence of cooked meat (Bello Acebrón and Calvo Dopico, 2000; Joo et al., 2013) and iii) reliance quality traits (RQT) such as safety, nutrition, animal welfare, ethics, price, product presentation, origin, and brand of meat products (Joo et al., 2013; Troy and Kerry, 2010).
Poultry meat is composed primarily of muscle and variable quantities of connective tissues. It contains also some epithelial and nervous tissues, as well as adipose tissue, bone, and cartilage. Muscle and connective tissues are the most abundant tissues in meat, and their properties and relative proportion of each tissue are responsible for the leanness and quality of meat (Listrat et al., 2016). Muscle fiber biochemical and structural characteristics can be independently manipulated by intrinsic and extrinsic factors to achieve production efficiency and improve meat quality (Listrat et al., 2016). These muscle fibers are characterized by their morphological traits, contractile, and metabolic properties (Astruc, 2014; Joo et al., 2013; Lefaucheur, 2010). The morphology traits are classified by a total number of fibers (TNF), the cross-sectional area of fibers (CSAF), and fiber type composition (FTC) (Joo et al., 2017). In general, muscle fiber types in adult skeletal muscle can be categorized as type I (slow oxidative), type IIA (fast oxido-glycolytic), type IIX and IIB (fast glycolytic) (Schiaffino and Reggiani, 1996). All of these fiber types are observed in most mature mammalian skeletal striated muscles and their relative composition in the different muscles can determine the predominance of muscle’s metabolic properties (Ozawa et al., 2000; Ryu and Kim, 2005).
Another factor affecting poultry meat quality is growth rate. Over the past 50 years, fast-growing demand for poultry meat in the world has resulted alteration of muscle fibers to the maximum functional size and put pressure on chicken breeders and farmers to increase the growth rate of the chicken, feed efficiency, size of breast muscle, and reduction in abdominal fatness (Petracci and Cavani, 2012). Today, poultry meat, especially chickens, are marketed in about half the time and at about twice the body weight compared to the 50 years ago (Barbut et al., 2008). These improvements are mainly due to the high heritabilities of body weight and body composition during breeding (Le Bihan-Duval et al., 2003). However, we should notice that fast muscle growth influence on poultry meat quality, resulting in deteriorating the quality. In order to produce high-quality poultry meat, it is necessary to understand the characteristics of poultry meat quality traits and factors to control them. Therefore, the relationship between muscle fiber characteristics and poultry meat quality must be well understood and detrimental effects of fast growth rate in poultry industry on meat quality also should be widely studied. The present paper reviews the scientific literature on poultry meat quality traits in relation to muscle fiber characteristics and muscle growth factor on poultry meat quality.
Poultry Meat Quality
The term ‘poultry meat quality’ is not standardized and there are many definitions in interpreting poultry meat quality. According to Duclos et al. (2007), to produce a carcass with a good quality, the carcass must have maximal meat yield with a low-fat content. Le Bihan-Duval et al. (2008), stated that changes in pH during postmortem responsible for the variations in meat quality. While Sokołowicz et al. (2016) suggested that poultry meat quality is made up of its safety, nutritive value and sensory characteristics. However, Baracho et al. (2006) defined in different context i.e., poultry meat quality must meet consumers’ demand and this can be done by controlling production chain from farm to processing plant, and the use of technologies to reduce risk factors throughout the production chain to allow the production of better quality poultry meat, and consequent reduction of losses. Also, meat quality can be determined by scientific factors including composition, nutrients, colorants, water-holding capacity (WHC), tenderness, functionality, flavors, spoilage, contamination, etc. (Joo et al., 2013). Quality traits of poultry meat are categorized based on intrinsic (AQT and EQT) and extrinsic factors (RQT) (Joo and Kim, 2011) as discussed below.
The first impression consumers have of any meat product is its color and thus meat color is the most important AQT because it is used an indication of freshness and wholesomeness (Joo et al., 2013). If meat has an undesirable color, it could be rejected even before evaluation of its other properties; such as tenderness, flavor, texture, and taste. This is why meat color is of such importance at the point of sale. Basically, meat color is dependent on species, age, and muscle type. There are significant differences in color range among meat from different species, and the differences are due to the different content of myoglobin (Mb) in muscle (Joo et al., 2013). The higher Mb content in type I muscle fibers are due to the function of Mb to store and deliver oxygen in the muscle and this can be affected by factors such as exercise and diet of the animal as well as genetic and environmental factors (Joo et al. 2013).
Chicken meat discoloration is closely related to the decrease in muscle pH postmortem. Rapid postmortem decline in pH results in PSE-like meat (pale, soft and exudative characteristic), with pale appearance and lower WHC (Owens et al., 2000; Pietrzak et al., 1997). In the chicken meat, normal pH values (at 15 min postmortem) are around 6.2 to 6.5 (Berri et al., 2005; Kijowski and Niewiarowicz, 1978), whereas normal ultimate pH values are around 5.8 (Fletcher, 1999; Van Laack et al., 2000). If the pH value is low (< 5.8) and near to the isoelectric point of the myofibrillar protein leads to a decreased WHC and a discoloration of meat. While lower WHC in the meat system could also affect other important factors of AQT which is drip and purge loss (Joo et al., 1995). PSE is a serious defect in chicken meat because in meat system, water is not closely bound to proteins, and cell membranes are very permeable in pale meat (Rosenvold and Andersen, 2003) resulting to drip and purge loss. WHC is affected by many factors including rigor temperature and membrane integrity (Honikel et al., 1986), pre-slaughter stress, processing factors, and packaging (Payne et al., 1997). Additionally, WHC influences on other physical properties including texture and firmness of raw meat and eating properties of cooked meat. Therefore, WHC is very important to the meat producer and seller as well as the consumer, because it strongly influences consumers’ acceptance and the final mass of meat that sells by weight (den Hertog-Meischke et al., 1997).
Several studies found that the relationship between fastgrowth rate and muscle texture defect in poultry meat, with increasing growth rate and muscle size, could potentially increase the incidence of pectoral myopathies or deep pectoral disease (Lien et al., 2012; Velleman et al., 2003). Moreover, an increase was observed in susceptibility to stress-induced myopathies, which can impair meat quality and favor the occurrence of abnormalities such as PSE-like meat (Anthony, 1998; Duclos et al., 2007; Petracci et al., 2009; Strasburg and Chiang, 2009). At the present, a high increase of quality issue such as white stripping (Kuttappan et al., 2012; Petracci et al., 2013), poor cohesiveness and hypercontraction in breast meat (Petracci and Cavani, 2012), and woody breast (Chatterjee et al., 2016) has been observed at the poultry-industry level. The origin of these new quality defects is still not fully understood, but it can be hypothesized that there is a strict connection with the fast growth rate (Petracci et al., 2013).
In EQT, tenderness is the most important attributes that strongly influence consumer purchase for a poultry product. Many external factors contribute to the wide variation in meat tenderness such as the bird and environment, antemortem and/or postmortem handling conditions and cooking method. Age, strain, and sex as well as environmental stresses and feed restriction have all been shown to influence the variation in tenderness among meat samples (Owens et al., 2004; Sams, 2002; Smith and Fletcher, 1988). The intrinsic factors include the composition and contractile state of muscle fibers, the amount and solubility of connective tissue and the extent of proteolysis in rigor muscle (Joo et al., 2013). The contractile state of the muscle (myofibrillar component) is probably the most important influence in meat tenderness in market aged (6-8 wk) broilers (Owens, 2014). The contractile state of the muscle can be determined by measuring the length of the sarcomeres, with shorter sarcomeres length being strongly correlated with tough meat (Herring et al., 1967; Locker, 1960). Even though the number of connective tissues in epimysium, perimysium, and endomysium location increases during growth, the mass of muscle fibers increases more rapidly, resulting in dilution or reduction of the percentage of connective tissue in muscle. The tensile strength of connective tissue increases because of chemical crosslinking, which occurs in collagen with advancing age (Swatland, 1994).
Flavor is another important factor in EQT because people expect certain attributes such as savory in meat. Free sugars, sugar phosphates, nucleotide-bound sugars, free amino acids, peptides, and nucleotides contribute to the meat flavor, also other nitrogenous components such as thiamine are considered as the main water-soluble flavor precursors (Mottram, 1998). Fatty acid compositions in muscle play a vital role in the flavor development of poultry meat (Pérez-Alvarez et al., 2010) as well as glutamic acid was found to have a detectable effect on the taste of chicken meat and this may contribute to the differences in flavor between meats (Farmer, 1999; Wattanachant, 2008). However, chicken meat is liable to lipid oxidation resulted in the development of off-flavor (Hunton, 1995; Jayasena et al., 2013). Lipid degradation, mainly the oxidation of the fatty acid components of lipids, results in several hundred volatile compounds, including aliphatic hydrocarbons, aldehydes, ketones, alcohols, carboxylic acids, esters, some aromatic hydrocarbons and oxygenated heterocyclic compounds such as lactones and alkylfurans (Jayasena et al., 2013; Mottram, 1998). During cooking, Maillard-lipid interactions are considered to be the main reactions, which result in flavor and aroma compounds development. Around 500 volatile compounds have been identified in cooked poultry meat, and the majority of them are found in chicken meat (Brunton et al., 2002).
Intramuscular fat (IMF) is often recognized as a key factor for the development of sensory qualities of poultry meat particularly influence the juiciness, flavor, and tenderness (Hocquette et al., 2010). In chickens, IMF is not visible and not anatomically separable, which makes it difficult to investigate the mechanism of its deposition (Liu et al., 2016). As a crucial factor affecting meat quality, IMF has always been a focus of attention in meat-type poultry breeding. IMF is different between species, breeds, muscle types in the same breed, gender, age, and feeding, and the accretion rate of IMF content is not only dependent on the metabolic activity of adipocytes but also on the muscle growth rate and metabolic activity of other organs. For instance, tissue with a high glycolytic activity (e.g. white muscle) generally display a reduced development of IMF (Hocquette et al., 2009). This suggests that muscle development and growth is closely related to deposition of IMF, which is crucial in meat quality.
A quality meat must be a safe one and safety is always more important than the quality of meat. The microbial level in meat is the most important safety trait for meat products. Two different groups of microorganisms which are foodborne pathogens and organisms that are generally harmless to human health (Mead, 2004). Bacteria spoilage results off-odor development and the shelf-life of the product are mainly influenced by both number of bacteria present and history of temperature control at all stages of production, storage, and handling (Pooni and Mead, 1984). In general, visual and odor evaluation are a rapid assessment of meat spoilage by the consumer. The odorous substances found at spoilage in chill-stored poultry could be attributed to microbial growth (Mead, 2004). High microbial levels develop not only abnormal meat colors such as green and brown, but also off-odors such as putrid, sour, or sulfurous (Joo et al. 2013).
Salmonella and Campylobacter spp. are not only predominant foodborne pathogens in poultry meat, but also Clostridium perfringens, Escherichia coli 0157, and Listeria monocytogenes, together with those recognized recently, such as Arcobacter and Helicobacter spp. are implicated to human illness (Corry and Atabay, 2001). Therefore, application of the Hazard Analysis Critical Control Point (HACCP) system in the processing of meat has been developed and implemented in many countries as a management tool in the control of foodborne pathogens. This HACCP system can help to control contamination of carcasses with spoilage organism by strictly controlling the critical control point (CCP). The control measures can range from good farming/manufacturing practices and good sanitary/hygiene practices to targeted approaches, specially designed to minimize or eliminate carriage and shedding of key pathogenic bacteria (Troy and Kerry, 2010).
Chicken meat has several advantages over red meat, especially in nutrient content. The fat content in chicken breast contains less than 3 g fat/100g while dark muscle of chicken meat (skin off) is 5-7 g/100g. Chicken meat contains desirable monounsaturated fats and it is made up from about half of the fat from chicken meat, and only one-third from chicken meat contains less healthy saturated fats. Interestingly, chicken meat does not contain the trans fat that is commonly associated with coronary heart disease, but this trans fat can be found in beef and lamb. Also, poultry meat is a good source of polyunsaturated fatty acids (PUFAs), especially omega (n)-3 fatty acids. If the chicken feed contains 0.24 mg of selenium/kg feed, the selenium content in breast meat increase from 8.6 µg to 41 µg/100g. Selenium deficiency is becoming more widespread in humans diet and this selenium act as an antioxidant to prevent some forms of cancer (Farrell, 2013).
Animal welfare and ethics is a great concern around the world because many countries livestock animals are killed without pre-stunning. Most develop and many developing countries have legislation that requires pre-slaughter stunning, with the exception of authorized ritual slaughter such as Halal and Kosher (FAO, 2001). This normally can be linked to the religious matters and also it is an essential part of the religious life to consume only ritual slaughter meat. Even though, ritual slaughtering causes a public debate in animal welfare but handling and treatment of animal during restraint must not compromise animal welfare. Currently, some efforts are being made to produce agreed standards and certification of ritual slaughtering, for example, Halal standard. However, issues such as authenticity of Halal certification, illegal slaughter and unfit meat sale, lack of auditing standards, mechanical killing of animals (poultry), animal welfare compromises, labeling (stun/non-stun), existing self-appointed certification bodies in different countries compete with each other to produce their Halal meat, and etc. cause difficulty in implementation (Anil et al., 2006).
Muscle Fiber Characteristics of Poultry Meat
The muscle fiber characteristics such as a total number of fibers (TNF), cross-sectional area of fibers (CSAF), and fiber type composition (FTC) vary by various factors including species, breed, sex, genotype, rearing system, feeding system, growth performance, diet, age, and muscle location. If able to control these factors, we can manipulate TNF, CSAF, and FTC, and finally can produce good quality poultry meats. There is a difference in muscle fiber characteristics between species, for example, duck breast muscle is significantly different with chicken breast muscle (Kim et al., 2008). The smaller type IIA and IIB fibers in duck breast muscles indicate that TNF is higher in duck muscles compared to chicken muscles, as well as, CSAF type IIA and IIB are smaller in duck muscles compared to chicken (Fig. 1). According to Kim et al. (2008), muscle fibers of duck breast contained 73.3% type IIB and 26.7% type IIA, whereas chicken breast contained 100% type IIB muscle. This entire type IIB muscle fibers (glycolytic) of chicken breast muscle, capable of short bursts of activity for the “Fight or Flight” response (Petracci and Cavani, 2012). Other important factors that influence TNF, CSAF, and FTC of a given muscle within species are breed. In general, wild birds contain more oxidative fibers, less glycolytic fibers, and smaller fibers compared to domesticated birds (Ashmore et al., 1972; Scanes, 2014).
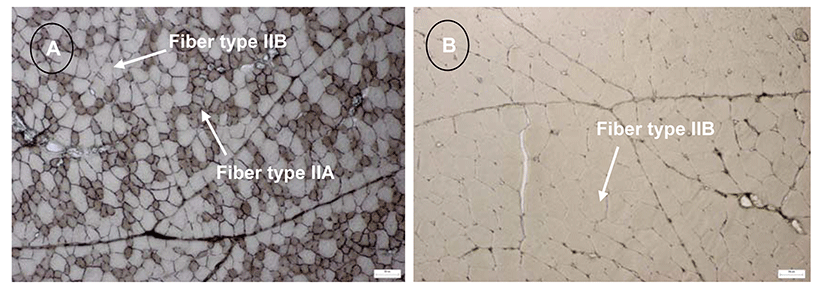
At birth, muscle is composed of oxidative fibers and the proportion of oxidative fibers decreases while the proportion of glycolytic fibers increases during growth. The decrease in type I and IIA fibers and an increase in type IIB fibers are due to the increase carcass weight. Ultimate muscle mass is significantly determined by the number of muscle fibers formed before birth and the size of those fibers after birth (Picard et al., 2006; Rehfeldt et al., 2008). Thus, an increase in muscle mass obtained by selection is mainly due to changes both the number (hyperplasia) and area (hypertrophy) of muscle fiber (Fowler et al., 1980; Remignon et al., 1995). An excessively high weight of carcass has influenced on pale and exudative properties in chicken breast because of muscle fiber characteristics, especially increased type IIB fibers. With regards to birds sex, Choi et al. (2016) reported that for quail muscle, greater muscle mass of the heavyweight male was accompanied by a higher percentage of type IIB fiber compared to heavyweight female (64% vs 51%). This could be due to the fact that muscle mass is mainly influenced by TNF, and glycolytic fibers exhibit largest CSAF, for a given TNF, an increase in the proportion of glycolytic fibers lead to an increase in muscle weight (Chen et al., 2007), which male muscles exhibit higher number than female muscles (Tůmová and Teimouri, 2009).
On the other hand, the adequate level of energy and the balance of nutrients play an important role in determining growth rate and feed efficiency in the postnatal period (Joo et al., 2013). However, the effect of feeding regime on the muscle fibers of poultry is still unclear. According to Chodová and Tůmová (2017), feeding regime had no effect on the number of muscle fibers per 1 mm2 in pectoralis major. Because, the CSAF immediately after the end of feed restriction was similar for both restricted and ad libitum fed groups (Chodová and Tůmová, 2017). However, Li et al. (2007) found that the glycolytic muscle fibers CSAF was reduced in lateral gastrocnemius muscle of restricted group after feed restriction at 14 days of age. These inconsistent results may be affected by various methods of feed restriction and different muscles the fibers of which were measured (Chodová and Tůmová, 2017). For instance, the gastrocnemius muscle has highly fast-twitch glycolytic fibers, while pectoralis major is composed of slow-twitch oxidative muscle fibers and is less sensitive to changes in nutritional status (Tesseraud et al., 1996).
Muscle fiber characteristics also are affected by muscle type, location, and function within an animal. Four types of muscle fibers are dynamic structures which exhibit high plasticity, fibers undergo type shift following an obligatory pathway I ↔ IIA ↔ IIX ↔ IIB (Pette and Staron, 2000; Schiaffino and Reggiani, 1996). Depending on the type and duration of the activity, FTC in muscle can be changed via physical exercise which induces a IIB → IIX → IIA → I transition in muscles when prolonged endurance in exercise (Lefaucheur, 2010). Additionally, in avian muscles, pectoralis major muscle has a high proportion of IIB fibers (Iwamoto et al., 2003; Roy et al., 2006), whereas the biceps femoris is composed of type I, IIA, and IIB (Papinaho et al., 1996). Type IIIA and IIIB fibers are not found in mammals but are found in muscles such as the plantaris and anterior latissimus dorsi of the avian species (McKEE, 2004).
Growth Rate on Poultry Meat Quality
Modern birds grow very fast due to genetic selection, efficient production systems, improved nutrition, and regular veterinary attention. Meat chickens reach a market weight as early as 6 wk and have high breast meat yields due to high demand for breast meat (Fanatico et al., 2007). However, selection for rapid growth and high pectoralis yield may have negative impact on sensory and functional qualities of the meat (Dransfield and Sosnicki, 1999; Le Bihan-Duval, 2003), pushing muscle fiber to their maximum functional size constraints (MacRae et al., 2006). Fanatico et al. (2007) studied the weight gain of the fast-growing birds exceeded that of the slow-growing birds even though the slow birds were started earlier in an attempt to reach a similar weight. Conventional diet has no significant difference in weight gain of both genotype, but, the low-nutrient diet reduced the weight gain of the slow-genotype but not the fast-genotype. Although the weight of the fast-genotype was maintained on the low-nutrient diet, breast yield was reduced. Plus, the fast-genotype exhibited superior breast yield (Fanatico et al., 2007; Mikulski et al., 2011), whereas slow-growing genotype had a higher wing and leg yields (Fanatico et al., 2008).
Slow-growing chickens had higher protein content and a lower fat content of breast meat (Fanatico et al., 2007; Mikulski et al., 2011; Wattanachant et al., 2004). Contrarily in duck muscle, Baeza et al. (2002) found that fast-growing ducks had increased protein and mineral contents and decreased moisture in breast muscle compared with slow-growing ducks. Thus, species difference affected the protein and fat content. The values of L* (lightness) and a* (redness) in the meat of slow and fast-growing chickens are not consistent. Fanatico et al. (2005) reported that slow-growing broiler had lower redness than fast-growing, and the indoor slow-growing bird was lower in redness than fast-indoor type broiler. Other authors have found slow growing birds are redder and darker than fast-growing or high-performance birds (Berri et al., 2001; Debut et al., 2003; Le Bihan-Duval et al., 1999). The difference in the redness might be due to a difference in muscle fiber type (Lonergan et al., 2003). Breast meat of fast-growth rate birds is paler and less red (Berri et al., 2001), and this can be explained by a lower level of heme (Baeza et al., 2002), while slow-growing birds normally have a redder meat than fast-growing because the slow-growing are typically aged (Gordon and Charles, 2002). WHC between slow and fast genotypes also has been studied. The outdoor slow-growth bird had higher drip loss than fast birds (Debut et al., 2003; Fanatico et al., 2005; Mikulski et al., 2011). This can be explained by the higher yield of breast muscle of fast-growing bird, and the bigger breast muscles are thicker and therefore do not lose as much water as thinner breast muscle (Fanatico et al., 2005).
The histological and biochemical modifications due to genetic progress have put more stress on the growing bird and impaired some meat quality traits (Petracci and Cavani, 2012). The defects commonly occur in fast-growing birds than slow-growing. Several studies reported that fast-growing strains exhibit a high incidence of deep pectoral myopathy or green muscle disease (Dransfield and Sosnicki, 1999; Petracci and Cavani, 2012). Even though this condition was first recognized in adult meat-type turkey and chicken muscle, it has become more common in meat-type growing chicken which has more muscle fiber type IIB.
The other emerging quality issue in poultry is the poor cohesiveness of meat due to the immaturity of intramuscular connective tissue (IMCT). This normally related to the very early slaughter age of modern chicken strains (Petracci and Cavani, 2012). The structural integrity of muscle fibers is maintained by three layers of IMCT, endomysium, perimysium, and epimysium (Nishimura, 2010). During breast muscle development in modern broiler breeds, an increase in the cross-section of muscle fiber is greater than that in endomysium and perimysium connective tissue (Dransfield and Sosnicki, 1999). This suggests that selection for rapid growth had created muscles that outgrow their life support systems and bring about muscle damage (Dransfield and Sosnicki, 1999). Thus, the formation of large intercellular spaces occurs and cause fluid lost from the postmortem muscle fibers (Swatland, 1990).
One of very new emerging meat quality problem is the appearance of white striping or striations in poultry breast fillets following the directions of muscle fiber (McKee et al., 2010). Although this is not linked to any particular eating attributes of cooked poultry, it would possibly lead to consumers not selecting the product due to its appearance. Histological observations indicate an increase in degenerative and atrophic fibers in breast fillets affected by white striping (Petracci and Cavani, 2012).
The other emerging challenge to the poultry industry is the woody breast condition that is a novel chicken muscle abnormality (Owens, 2014; Petracci et al., 2015). Woody breast is characterized by diffuse areas of hardness and overall muscle rigidity (Chatterjee et al., 2016). The meat with the wooden breast condition is hardened to the touch and exhibits hardened “ridges” along the ventral portion of the breast. This can be associated with muscle fiber fragmentation, hyalinization, myofiber swelling, necrotic muscle fiber replacement with connective tissue, macrophage infiltration, and the presence of irregular patches of adipose tissue (Chatterjee et al., 2016; Sihvo et al., 2014). Although the woody breast is closely associated with fastgrowth rates in broilers with high breast meat yield, the specific causes of myopathy are yet unknown. Observations showed that histopathological changes in white striped and wooden breast muscles had similar features (Ferreira et al., 2014; Kuttappan et al., 2013; Sihvo et al., 2014). Rapid growing and increasing breast meat yield seemed to be responsible for breast muscle overstretching and capillary density lowering, with ischemia, inflammation and reparative fibrotic response (Kuttappan et al., 2013). Consequently, this increases metabolic stress within the muscle tissue, resulted in the woody breast (Dalle Zotte et al., 2017).
The most common challenges to the poultry meat industry associated with the intensive selection for increased muscling is a development of pale, soft and exudative meat (PSE). Some believe the selection of fast-growing chicken has resulted in an increase of the PSE problem. This PSE-like poultry meat was mentioned decades ago, but there is no evidence to support or refute a genetic mutation in chicken and turkeys as like PSE pork. It was suggested that the postmortem muscle behavior of the fast glycolyzing group was fairly similar to the phenomenon seen in PSE pork muscle (Barbut et al., 2008). However, several studies have been conducted to investigate the main cause of the PSE-like condition in poultry (Owens et al., 2009; Petracci et al., 2009). Rapid growing strain of poultry exhibited an increased susceptibility to stress-induced myopathy (Mitchell, 1999; Sandercock et al., 2006) and these pathologies are attributable to alteration in intracellular calcium homeostasis (Sandercock and Mitchell, 2003; Sandercock et al., 2006). Consequently, sarcolemmal integrity changed and resulted in excessive myofiber hypertrophy and inadequate development of support tissues and vascular supply (MacRae et al., 2006; MacRae et al., 2007). These myopathies may have profound implications for poultry meat quality and the incidence of the specific condition such as PSE-like chicken meat (Petracci and Cavani, 2012).
Conclusion
Determination of a standard for good quality of poultry meat is difficult because poultry meat quality is very ambiguous and is not consistent with definition. It is dependent on many aspects of intrinsic and extrinsic, but the most important factor is to meet consumers’ need. In order to identify the definition of ‘poultry meat quality’, this term can be simplified by following categories which are AQT, EQT and RQT. The AQT in poultry meat includes skin and meat color, drip and purge, and texture, while EQT consist of tenderness, flavor, and juiciness. The components of RQT are safety, nutritional value and animal welfare. These poultry meat quality traits are dependent on muscle fiber characteristics such as TNF, CSAF, and FTC in meat. These quality traits are evaluated by the consumer before buying the meat products, and these traits contribute to the consumer’s expectation towards good quality meat.
Quality characteristics of poultry meat also can be associated with the muscle fiber of TNF, CSAF, and FTC. TNF and CSAF are major determinant factors of muscle mass as well as meat quality, while FTC in muscle strongly related to fresh meat quality. However, current demand for rapid growth and high breast yield in the poultry industry, cause alteration of muscle fibers to the maximum functional size constraint and gives the negative impact on merit poultry meat quality. This might lead to the quality problem such as idiopathic myopathies, IMCT, white striping, wooden breast, and PSE-like meat. Although many studies have reported the relationship between histochemical and biochemical characteristics on meat quality, still the relationship between muscle fiber, growth-rate factor, and meat quality are not yet fully understood. Therefore, more information and further strictly controlled studies are needed to understand this uncertain relationship.