Introduction
Liver disease remains as a major cause of morbidity and mortality throughout the world. World Health Organization (2014) has recently reported global liver disease burden and 5.9% (about 3.3 million) of all death worldwide were mainly attributable to alcohol. Excessive alcohol consumption is directly associated with an increased risk of ALD. In Korea, the amount of alcohol consumption has been increased from 8.38 L/capita in the year 2000 to 8.54 L/capita in the year 2009 (Bang et al., 2015). Moreover, the alcohol consumption per capita is also tends to increase gradually in the future, thereby accelerating the risk of development of ALD and liver-related mortality on heavy drinkers.
ALD is a complex advanced disease that associates with a wide spectrum of hepatic lesions, from steatosis and steatohepatitis to fibrosis and cirrhosis (Louvet and Mathurin, 2015). Steatosis and steatohepatitis are potentially reversible by timely treatment. Indeed, the reversibility of both steatosis and steatohepatitis has been demonstrated experimentally via animal models of ALD (Lu et al., 2012). ASH, in particular, is one of the most common form of all the alcohol-related liver lesions. A growing body of literature has suggested that multiple pathogenic factors are involved in the development of ASH. Excessive alcohol intake can induce the generation of reactive oxygen species that disrupts hepatocyte metabolism, which contributes to hepatocyte injury (Szabo, 2015). In addition to reactive oxygen species, bacterial endotoxin (lipopolysaccharide) and nitric oxide have also been recently recognized as major factors that contribute to the abnormal gutliver axis in ASH. Elevated levels of both bacterial endotoxin and nitric oxide mediated inflammation and altered immunology defense mechanisms and homeostasis in the gut-liver axis (Rao, 2009). These complications subsequently result in impaired intestinal barrier function and integrity, as well as liver damage in ASH. Thus, therapeutic strategies targeting specific pathways to restore liver function, and to reduce nitric oxide levels, development of intestinal hyperpermeability, and pro-inflammatory signal that contributes to inflammation in ASH could limit the progression of fibrosis.
Probiotics are “live microorganisms that, when administered in adequate amounts, confer a health benefit on the host” (Hill et al., 2014). Administration of host-derived probiotics are increasing globally, attributed to increased consumer interest in natural health-enhancing resources for treatment of various diseases. Despite host-derived probiotics have been well documented for the treatment and/or prevention of gastrointestinal disorders, a growing number of clinical studies propose that these microorganisms have therapeutic potentials beyond gut health. Probiotic microorganisms exert various gut health benefits mainly through the modulation of gut microbiota and production of bioactive metabolites. Owing to the close anatomical and functional relationship between gut and the liver; these could fundamentally extend the impact of probiotics on liver health. Interestingly, the interaction between the host-derived probiotic strains and the gut-liver axis has been the major focus of attention in the emerging trends for the treatment of ASH. It has been demonstrated that host-derived L. rhamnosus GG could reduce intestinal oxidative stress and prevent increased intestinal permeability, leading to ameliorated ASH in rats (Forsyth et al., 2009). Furthermore, oral administration of heat-killed L. brevis SBC8803 also capable of inhibiting gut-derived endotoxin migration into the liver, and thereby improve alcohol-induced liver injury in rats (Segawa et al., 2008). These animal studies collectively suggested that host-derived Lactobacillus strains have the potential to be represented as promising therapeutic agents to prevent ASH and its related complications. Although promising, more studies are needed to better delineate the protective effect of these strains on ASH.
Previous studies have reported that L. fermentum LA12 isolated from Korean infant feces exhibited immune modulatory activities and was capable of extending lifespan in a nematodal model organism (Lee et al., 2011); demonstrating its therapeutic potential for the treatments of inflammatory-related diseases. However, the possible protective effect of L. fermentum LA12 on ASH is remains to be described. Therefore, the aim of the present study was to evaluate the effect of daily oral administration of L. fermentum LA12 on intestinal barrier function and liver function in a rat model of ASH.
Materials and Methods
Pure culture of L. fermentum LA12 was obtained from the Food Microbiology Laboratory, College of Life Sciences and Biotechnology, Korea University (Korea). L. fermentum LA12 was activated successfully three times in Lactobacillus de Man, Rogosa and Sharpe (MRS) broth (Difco, USA) at 37°C for 20 h prior to use. Stock culture was suspended in sterile 50% (v/v) glycerol and stored at −80°C until further use.
This study was approved by the Korea University Institutional Animal Care & Use Committee (KUIACUC-20151023-1). Eight-week-old male Sprague-Dawley rats, weighing from 250 to 300 g, were purchased from Samtako (Korea) and acclimated for a week, at 24°C with 50-60% relative humidity and 12 h light-dark cycle. During acclimation, rats were fed with standard rodent diet (Samtako, Korea), water ad libitum, and housed individually in the polycarbonate cages.
Activated cell cultures 1% (v/v) of L. fermentum LA12 was inoculated into MRS and incubated at 37°C for 20 h. Pelleted cells were subsequently collected by centrifugation (8000 g, 15 min, 4°C) and washed thrice with 0.1 M PBS. The pelleted cells of L. fermentum LA12 was suspended with 0.1 M PBS, and adjusted to a density of 109 CFU/mL prior to use.
After acclimation, thirty-two rats (n=8 /group) were administered intragastrically gavage twice daily with ethanol or dextrose. Ethanol-fed rats received 50% (v/v) ethanol gavage twice daily starting with an initial dose of 2 g/kg/d. This dose was progressively increased every week to a maximum dose of 5 g/kg/d that was continued for 4 wk. Control rats were administered oral gavage with an isocaloric amount of dextrose. These rats were divided into four treatment groups: 1. dextrose; 2. dextrose + L. fermentum LA12 (109 CFU/mL); 3. ethanol ; 4. ethanol + L. fermentum LA12 (109 CFU/mL). At 5 weeks, the rats were sacrificed by CO2 inhalation. Blood samples were immediately collected by cardiac puncture, and serum was obtained via centrifugation (2500 rpm, 10 min) for further analyses. Liver and intestine were then harvested for further use.
Nitric oxide level in small intestinal tissues was measured using nitrate/nitrite colorimetric assay kit (Cayman Chemical, USA). Total nitric oxide (NO) level was expressed as the sum of nitrite (NO2) and nitrate (NO3). Absorbance of samples was measured spectrophotometrically at 550 nm.
Small intestine was isolated and kept in modified KrebsHenseleit bicarbonate buffer (KHBB; pH 7.4). A segment of the ileum was first ligated with suture, and 100 µL fluorescein isothiocyanate (FITC)-dextran (molecular weight 4 kDa, FD-4, 40 mg/mL) was injected into the lumen of the isolated distal ileal loop. The other end of the ileum segment was then ligated, and placed in KHBB, and incubated at 37°C for 20 min. The FD-4 that penetrated from the ileum segment into the incubation buffer was measured spectrofluorometrically at an excitation wavelength of 485 nm and an emission wavelength of 530 nm. The fluorescence emission of control group was normalized as 100%
Fresh liver and small intestinal tissues were harvested and fixed in 10% (v/v) formalin for 24 h at 4°C. The samples were then dehydrated with ethanol (three times 95% (v/v) ethanol for 1 h; twice 100% (v/v) ethanol for 1 h), and dealcoholized twice with xylenes (Sigma-Aldrich, Germany) for 1 h. The dealcoholized samples were then embedded with Paraplast® (Leica Microsystems, Germany), and cut into 5 μm sections using microtome CUT 5062 (Slee medical GmBH, Germany). For histological analysis, one section was stained with hematoxylin and eosin, and examined under the light microscope (Olympus, USA).
The mRNA expression of small intestinal tight junction proteins and tumor necrosis factor (TNF-α) was investigated using reverse transcription-polymerase chain reaction. Briefly, total RNA was isolated from samples (liver or intestine) using TRIzol® reagent (Invitrogen, USA) according to manufacturer’s procedures. One microgram total RNA was reverse transcribed in 20 μL reaction mixtures with the Super Script® ІІІ First-Strand Synthesis System for RT-PCR (Invitrogen, USA) with manufacturer’s procedures. The cDNA products were subsequently used for polymerase chain reaction. Oligonucleotides (Macrogen, Korea) sequences used for the amplification of each gene fragment of tight junction proteins and TNF-α were shown in Table 1. The RT-PCR products were then electrophoresed in a 1.5% agarose gel and visualized by ethidium bromide staining. The intensity of each band was determined using Kodak 1D image analysis software (Eastman Kodak Company, USA).
Endotoxin level in the serum was determined using Pierce Limulus Amebocyte Lysate (LAL) kit (Thermo Scientific, USA). A standard curve of endotoxin was made by using endotoxin of Escherichia coli. Absorbance of samples was then measured spectrophotometrically at 405 nm. Additionally, concentrations of aspartate aminotransferase (AST) and alanine aminotransferase (ALT) in the serum were also determined using Infinity AST and Infinity ALT Activity Assay Kits according to the manufacturer’s recommendations (Thermo Scientific, USA), respectively. Serum triglyceride was also measured using the Infinity Assay Reagent (Thermo Scientific, USA).
Myeloperoxidase activity in the liver was determined using the Myeloperoxidase Colorimetric Activity Assay Kit (BioVision, USA). Briefly, the liver was homogenized in myeloperoxidase assay buffer, and supernatant was collected by centrifugation (13 000 x g, 30 min, 4°C). Supernatant was subsequently mixed with myeloperoxidase assay buffer and myeloperoxidase substrate. The mixture was then incubated at room temperature for 1 h, and added with tetramethylbenzidine probe. Absorbance of samples was then measured at 412 nm.
Data were analyzed statistically with IBM SPSS software (version 22) (IBM Corp., USA). One-way ANOVA was used to analyze the statistical difference between sample means. The statistical level of significance was preset at α=0.05. The multiple comparisons of means were assessed by Tukey’s test. All data were mean values of replicates from eight separate runs (n=8), unless stated otherwise.
Results and Discussion
Specialized intestinal epithelial cells constitute biochemical- and physical barrier surfaces that can sense and respond to external stimuli, which contributed to the maintenance of the fundamental intestinal barrier- and immunoregulatory functions (Peterson and Artis, 2014). However, alcohol may impair epithelial barrier functions by inducing cellular and tissue oxidative damage. NO is a vital, key endogenous oxidant that mediates epithelial cell injury and intestinal dysfunction. Many studies found that chronic alcohol intake upregulated inducible nitric oxide synthase (iNOS) and produced excessive levels of NO, thereby leading to an intestinal barrier dysfunction in an animal model of ASH (Tang et al., 2009b). In parallel, our study also demonstrated that daily alcohol feeding significantly increased NO-mediated oxidative stress in the intestine (Fig. 1). Interestingly, once-daily administration of L. fermentum LA12 for 4 wk significantly decreased (p<0.05) intestinal total NO level by 28% in the alcohol-fed rats, but the level remained significantly higher (p<0.05) than that of healthy rats (control). Inhibition of intestinal NO production not only reversed diabetes-induced Klebsiella pneumoniae liver translocation, but also decreased the severity of alcohol-induced intestinal injury and development of ASH (Lin et al., 2017b; Tang et al., 2009b). Hence, these data suggested that L. fermentum LA12 treatment may protect against injurious effect of alcohol on the intestine and liver by attenuating intestinal oxidative stress.
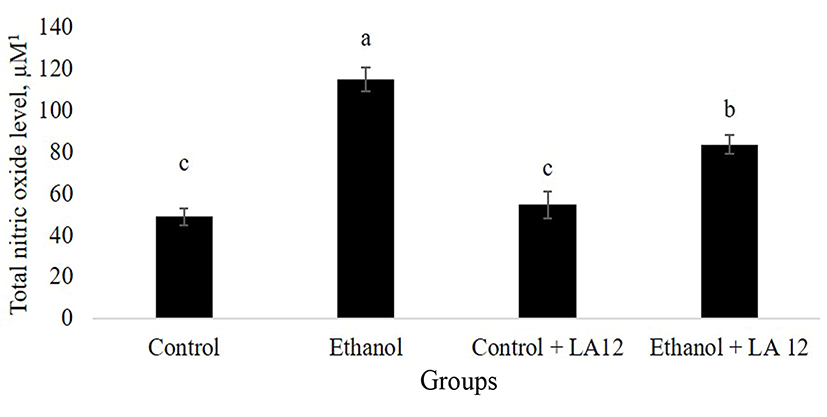
Purohit et al. (2008) have reported that alcohol-induced production of nitric oxide may also contribute to intestinal hyperpermeability to macromolecules. As shown in Fig. 2, intestinal permeability to FITC-dextran in alcoholfed rats was about 1.7-fold higher than control rats (p<0.05), demonstrating that alcohol-induced intestinal oxidative stress further increases intestinal hyperpermeability. In addition to alcohol, acetaldehyde, a highly toxic primary metabolite of alcohol metabolism, has also been proposed to play a potent role in the promotion of intestinal permeability (Rao, 2015). Alcohol feeding can promote the overgrowth of intestinal Gram-negative bacteriaencoding bacterial alcohol dehydrogenase and/or aldehyde dehydrogenase, which are capable of metabolizing alcohol into acetaldehyde (Malaguarnera et al., 2014; Nosova et al., 1998). Increased accumulation of acetaldehyde in the lumen may thus subsequently lead to an increase in intestinal hyperpermeability. However, L. fermentum LA12 significantly reduced (p<0.05) the permeability of intestine in alcohol-fed rats, and the value showed no significant difference compared to that of control groups. Similarly, Forsyth et al. (2009) have also demonstrated that once daily administration of probiotic strain, L. rhamnosus GG significantly improved alcohol-induced intestinal hyperpermeability, and ameliorated gut-liver injury in the rats. Therefore, this result indicates that L. fermentum LA 12 treatment could improve the progression of ASH by altering alcohol-induced intestinal hyperpermeability.
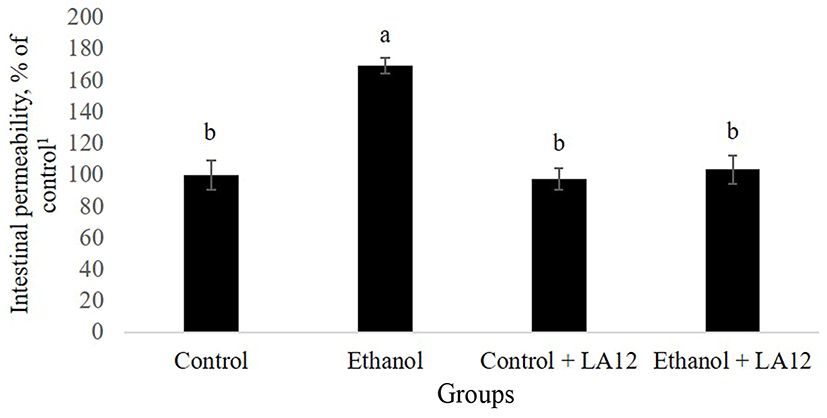
Several studies have consistently described that rats exposed to alcohol and alcohol-dependent subjects were associated with altered barrier functions and structural epithelial damage (Leclercq et al., 2017). Additionally, “leaky gut” could be an important determinant for the development of alcoholic liver disease; where intestinal permeability only elevated in alcoholics with liver diseases (Keshavarzian et al., 1999). These findings proposed that protection and restitution of gut barrier is crucial to prevent the progression to ASH. Histological analysis in this study showed that alcohol feeding caused abnormality in the small intestinal tissues compared to the control (Fig. 3A-D). In the control- and groups, the intestine appeared normal with intact epithelial barrier, organized enterocytes, and no signs of inflammation (Fig. 3A-B). However, alcohol feeding caused several signs of jejunal lesions, submucosa damage, and destruction of the tips of the villi, indicating alcohol-induced gut leakiness (Fig. 3C). These observations confirmed that the increased intestinal NO production and intestinal permeability as shown in Figs. 1 and 2 can further contribute to leaky gut. Upon 4 wk treatment, L. fermentum LA12 had clearly promoted villous recovery, increased crypt depth and villus height (Fig. 3D), leading to the alleviation of alcohol-induced intestinal barrier dysfunction. Hence, the improved intestinal permeability and decreased intestinal NO production in L. fermentum LA12-treated rats may be attributed to the restitution of structure and function of intestinal epithelial barrier.
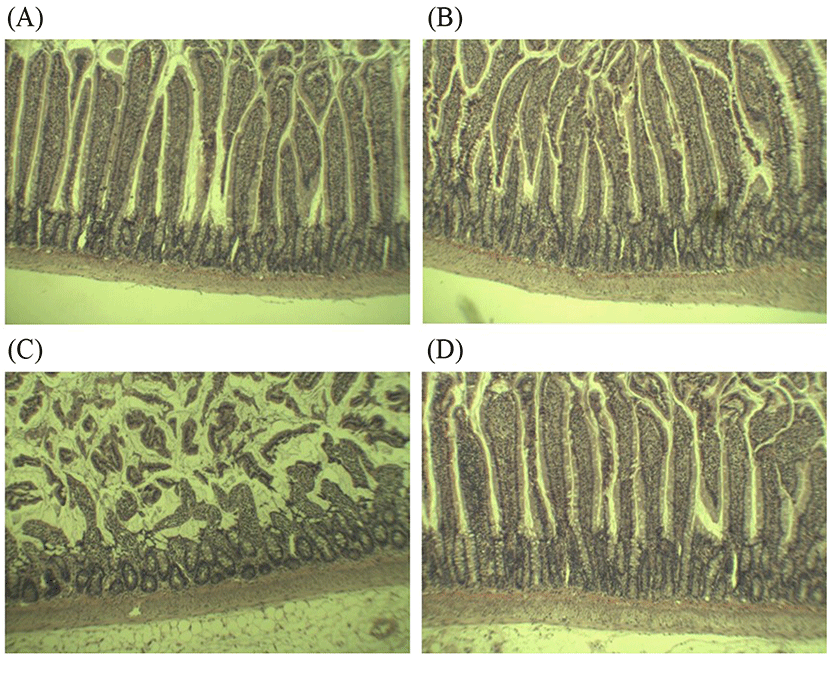
Intestinal tight junction proteins are multi-complex proteins that play an irreplaceable role in maintaining the intestinal mucosal barrier function. Tight junctions comprise of cytoplasmic scaffolding proteins zonula occludens 1-3 (ZO-1-3), and transmembrane proteins, namely occludin and claudin (Hartsock and Nelson, 2008). These intestinal tight junctions can be affected by exogenous factors, including alcohol and high-fat diet. Ingestion of alcohol has been shown to alter the assembly of tight junctions between the epithelial cells by decreasing the expression of occludin, and claudin-1, and ZO-1 (Li et al., 2012; Tang et al., 2008). In order to determine the possible protective mechanisms for alcohol-induced intestinal barrier dysfunction, the effect of L. fermentum LA12 on mRNA expression levels of ZO-1, claudin-1, and occludin in the small intestine were evaluated (Fig. 4A). Results revealed that alcohol feeding significantly down-regulated (p<0.05) mRNA expression of these three proteins compared to the control. However, we found that L. fermentum LA12 treatment significantly up-regulated (p<0.05) mRNA expression levels of occludin and claudin in alcohol-fed rats by about 1.1, 0.6-fold, respectively. The mRNA expression level of claudin in alcohol-fed group treated with L. fermentum LA12 were also no statistically significant differences from those of dextrose-fed groups. Additionally, the mRNA expression level of claudin in rats fed with dextrose and L. fermentum LA12 was significantly higher (p<0.05) than dextrose-fed rats, suggesting that L. fermentum LA12 can promote and regulate claudin-1 in both healthy- and defective mucosal barrier. These results collectively suggested that L. fermentum LA12 not only restored alcohol-induced intestinal barrier dysfunction, but also helped to maintain normal gut function by modulating intestinal tight junction proteins.
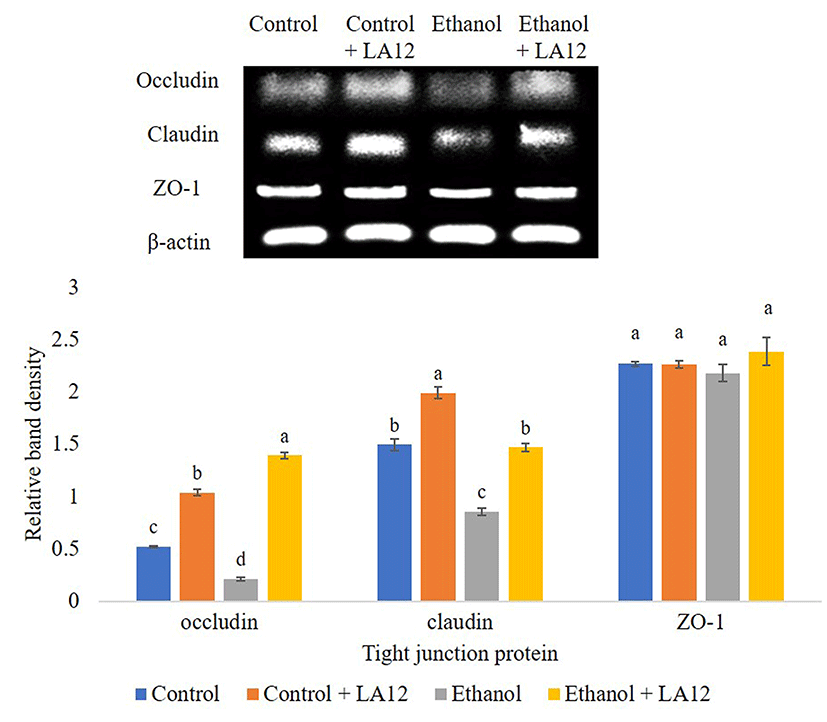
Daily alcohol feeding significantly affected intestinal oxidative stress and permeability (Figs. 1 and 2), thereby allowing intestine-derived endotoxin circulating in the blood (Fig. 5A). Previous studies have collectively suggested that endotoxin is a key cofactor promote liver damage in alcoholic subjects (Rao et al., 2004). However, as shown in Fig. 5A, L. fermentum LA12 is capable of reducing serum endotoxin levels (p<0.05) in alcohol-fed rats; thereby suggesting that L. fermentum LA12 may reduce systemic endotoxemia. Tang et al. (2009a) have suggested that attenuation of alcohol-induced oxidative cascade and tight junction structural integrity damage could prevent endotoxemia. Thus, the endotoxemia-limiting effects observed in ethanol + LA12 group could be attributed to the ability of L. fermentum LA12 that attenuated intestinal oxidative stress (Fig. 1) and restituted epithelial barrier functions (Figs. 2-4). Furthermore, serum triglyceride- (Fig. 5B) and liver enzymes analyses (Fig. 5C-D) demonstrated that alcohol feeding significantly impaired liver functions, in which the damaged hepatocytes spilled the triglyceride, AST and ALT into the blood (Wang et al., 2016). However, L. fermentum LA12 treatment significantly reduced (p<0.05) the serum level of triglyceride, AST, and ALT by 50.6%, 18%, and 24% respectively; in alcohol-fed rats. These results indicate that L. fermentum LA12 may play a protective role against liver damage.
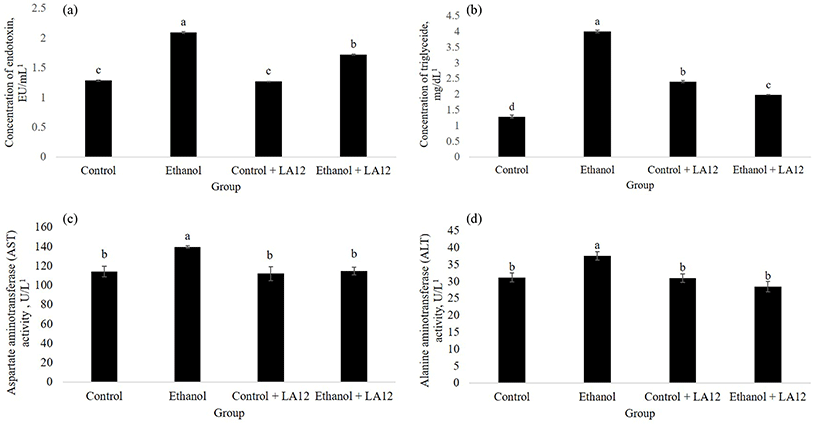
Oxidative stress and inflammation are key contributors to the progression of ASH. Myeloperoxidase (MPO) is a neutrophil enzyme involved in processing phagocytic material, as well as generates oxidants participate in molecular events responsible for various disease development. Chronic alcohol intake promoted hepatic MPO-generated oxidative stress, which can subsequently stimulate hepatic TNF-α production that lead to the development of ASH (Zhou et al., 2003). This was in tandem with our study, where alcohol treatment increased both hepatic-MPO activity (Fig. 6A) and hepatic mRNA expression level of TNF-α (Fig. 6B) in the rats. Interestingly, L. fermentum LA12 treatments for 4 wk significantly decreased (p<0.05) MPO activity and down-regulated mRNA expression of TNF-α in the liver. Lin et al. (2017) have proposed that hydrogen-rich water associated with antioxidant and anti-inflammatory effects can improve liver functions, thereby alleviating ethanol-induced fatty liver in mice. Altogether, these data suggested that L. fermentum LA12 treatment could improve liver function by ameliorating alcohol-induced oxidative stress and inflammation in the liver, subsequently preventing the leakage of liver enzymes (AST and ALT; Fig. 5) into the blood-stream.
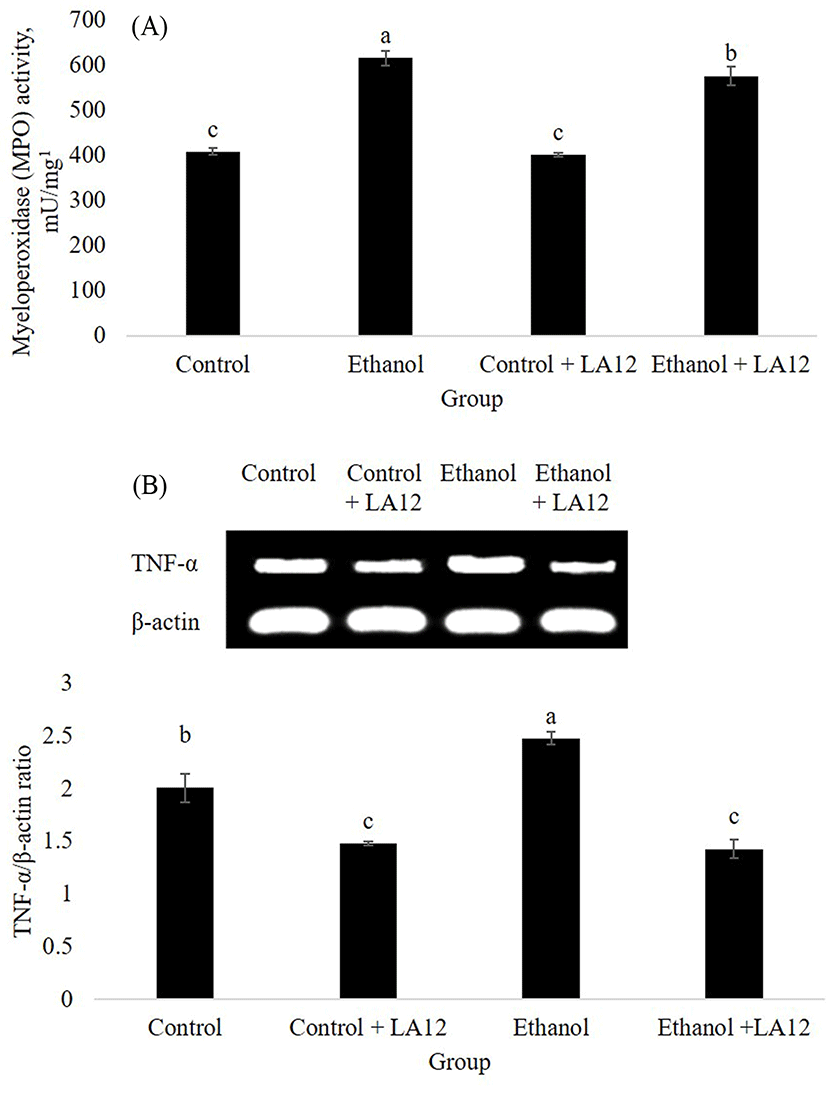
Alcohol can induce a wide spectrum of histologic changes in the liver. It has been reported that macro-vesicular steatosis is the first and most common histological change observed in 90% of alcoholic subjects (Mathurin et al., 2007). Fortunately, early formation of macro-vesicular lipid droplets is still manageable by following a prolonged period of abstinence from alcohol and/or prophylaxis (Frazier et al., 2011). Changes in the histological structure of the liver of four treatment groups in this study were illustrated in Fig. 7A-D. As shown in Fig. 7C, chronic alcohol intake result in the stimulation of hepatic neutrophil infiltration and formation of macro-vesicular lipid droplets, and subsequently induce liver injury and alter liver function (Figs. 5, 6). However, four-week L. fermentum LA12 treatment decreased neutrophil infiltration and formation of macro-vesicular lipid droplets in the liver of alcohol-fed rats, and showed no obvious histological difference as compared to the healthy rats (Fig. 7D). Alcohol intake often stimulated neutrophil transmigration to hepatocytes, and neutrophil accumulation in the liver subsequently exacerbates the liver damage by up-regulating inflammatory responses and increasing oxidative stress (Ramaiah and Jaeschke, 2007). These results indicate that administration of L. fermentum LA12 could improve hepatic steatosis via anti-inflammatory and anti-oxidant actions.
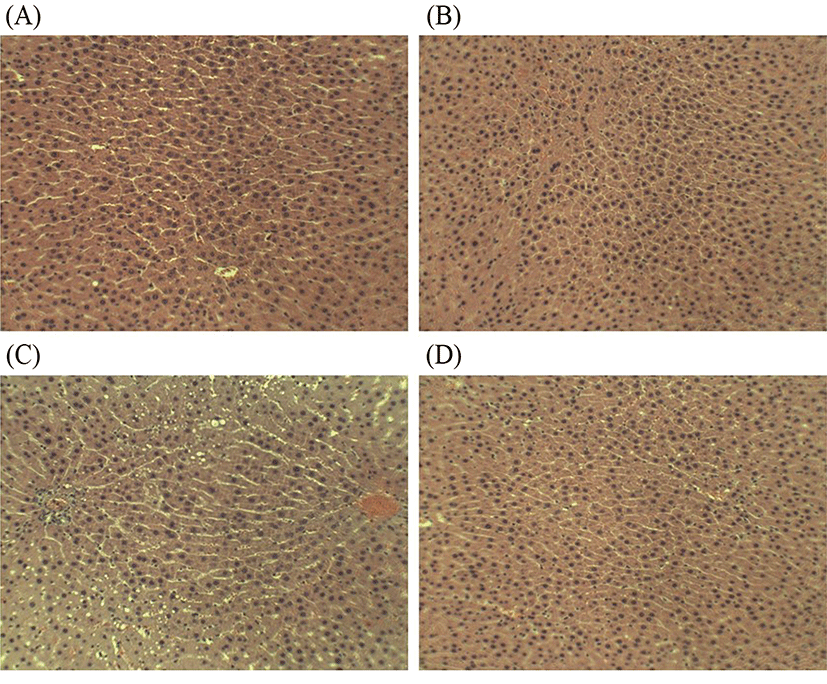
Conclusion
Taken together, results in this study suggested the potential use of L. fermentum LA12 for the prevention of alcoholic steatohepatitis via gut-liver axis. Four-week administration of L. fermentum LA12 was capable of stimulating restitution in intestinal barrier function and reducing alcohol-induced inflammatory mediators, thereby attenuating leaky gut and liver damage, and preventing the progression of ASH.