Introduction
Hypercholesterolaemia is a major risk factor for the development of cardiovascular diseases (CVD), heart disease and stroke. According to the American Heart Association, cardiovascular diseases are the leading global cause of death, accounting for more than 17.3 million deaths per year in 2013, representing 31% of all global deaths, and this number is expected to grow to over 23.6 million by 2030. Globally, 80% of CVD deaths take place in low- and middle-income countries and cost-effective medications such as statins remain unaffordable for much of the world (Benjamin et al., 2017).
Statins, inhibitor of the enzyme 3-hydroxy-3-methylglutaryl-coenzyme A reductase (HMGCR), are the most commonly used drug to reduce blood cholesterol levels, and are a well-established class of drugs in the treatment of hypercholesterolemia. HMGCR is a rate-limiting enzyme in the biosynthesis of cholesterol and catalyzes the reduction of HMG-CoA to mevalonate (Lennernäs and Fager, 1997). Regulation of HMGCR activity is the primary approach for controlling de novo synthesis of cholesterol. However, statins also exert side effects such as myopathy (specifically defined as muscle pain or weakness combined with large increases in blood concentrations of creatine kinase) and rhabdomyolysis affecting 0.1–0.2% of patients taking statins, especially when used in conjunction with other drugs (Bellosta et al., 2004) .Thus, there has been increasing development of various approaches to ameliorate this metabolic disease.
Hypercholesterolemia is a metabolic disorder that is primarily affected by dietary factors and lipid metabolism factors of the host. Animal products such meat, poultry, and eggs are the major sources of dietary fat and cholesterol amid increasing consumption annually and globally. Excess consumption of cholesterol-rich animal products is also one of the key factors that contributes to hypercholesterolemia. Therefore, efforts have been directed to improving the lipid profiles of the livestock via introduction of healthier feed and supplement.
Recent studies have reported that some strains of probiotics possess cholesterol-lowering capabilities with alleviation potentials of hypercholesterolemia-induced disorders (Liong and Shah, 2005b, Ooi et al., 2010b) . By definition, probiotic are defined as “live microorganisms that, when administered in adequate amounts, confer a health benefit on the host”(Morelli and Capurso, 2012). Lactobacillus is one of the main genus for probiotic microorganisms, has a long history of safe use, recognized by the US Food and Drug Administration (FDA) as generally regarded as safe (GRAS), and is approved for use in dairy products, functional beverages and nutritional powders (Bernardeau et al., 2006). Many strains of lactobacilli have also been incorporated into animal feed, with increasing evidence illustrating the efficacies of lactobacilli to produce healthier animals and thus healthier animal food products (Park et al., 2016).
Probiotics have been documented to lowers cholesterol through several mechanisms such as cholesterol assimilation, incorporation of cholesterol into cellular membrane, deconjugation of bile via bile salt hydrolase activity (Liong and Shah, 2005b), and more recently via the molecular approach by regulating of genes responsible for cholesterol homeostasis (Hong et al., 2016, Hu et al., 2013, Michael et al., 2017). Probiotics have been reported to regulate genes responsible for the intestinal transport of cholesterol (such as ABCG5, ABCG8, NPC1L1) as well as the genes responsible for the homeostasis of cholesterol in the liver (such as LDLR, and HMGCR). However, the exact mechanism remains unclear and more research is needed to investigate the regulation of the genes and the pathways. We hypothesized that probiotics exerted these effects via the AMP protein kinase (AMPK), the intracellular energy sensor that regulates the anabolic and catabolic pathways such as lipid homeostasis. Thus, in the present study, strains with potential cholesterol lowering ability was screened and selected. The effect of the selected strain on the gene expression of HMGCR along the AMPK pathway were determined in cultured human hepatoma HepG2 cell line.
Materials and Methods
All strains of Lactobacillus unless stated otherwise, were obtained from School of Industrial Technology, Universiti Sains Malaysia (Malaysia). Lactobacillus casei was isolated from Yakult fermented drink purchased from a supermarket in Penang, and was assumed to represent L. casei strain Shirota which was originally isolated from human feces. L. reuteri was isolated from Cardioviva purchased from www.amazon.com and was assumed to represent L. reuteri strain NCIMB 30242. L. plantarum DR7 and L. fermentum DR9 were isolated from fresh cow’s milk from Penang, and were obtained via courtesy of Clinical Nutrition Intl (M) Sdn. Bhd., Malaysia. Species identification was performed on all strains via 16S rRNA gene sequencing prior to use. All stock cultures were preserved in 20% glycerol (-20°C), activated in sterile de Mann, Rogosa, Sharpe (MRS) broth (Hi-media, Mumbai, India) for three successive times using 10% (v/v) inoculums and incubated at 37°C for 24 h prior to use. The cultures were centrifuged at 12,000×g for 5 min at 4°C and the supernatant was collected as the cell-free supernatant (CFS). All samples were kept at -80°C prior to analyses.
The assimilation of lactobacilli was determined as previously described (Liong and Shah, 2005a). Remaining cholesterol in the supernatant was quantified using Amplex Red cholesterol assay kit (Invitrogen, USA) as according to manufacturer’s instruction.
HepG2 (human hepatoma cell line), and HT-29 (human colorectal cell line), were cultured in Dulbecco's modified Eagle's medium (DMEM) (Gibco, USA) as previously described (Lew et al., 2013).
HepG2 and HT29 cells seeded in 12-well plates (1×105 cells per well) were incubated in serum free DMEM containing 1 mM cholesterol and 0.03% ox-bile for 24 h, at 37°C, with 5% CO2. The cells were then washed twice using cold phosphate buffered saline (PBS, pH 7.4). Lipids were extracted using 2 mL of hexane:isopropanol (3:2) for 30 min. The extracts were collected into a glass tube and evaporated under nitrogen gas. The amount of cholesterol uptaken and retained in the cells were extracted and quantified using Amplex Red Cholesterol Assay kit as according to manufacturer’s instruction. Intracellular protein was extracted from the cells in the 12-well plate using 2 mL of NaOH (0.1 N) with continuous shaking at 4°C for 16 h. Protein concentration was measured using Bradford’s method (Bradford, 1976). The intracellular cholesterol accumulation was expressed as per amount of intracellular protein content.
Total RNAs were isolated using TRISURE reagent (Bioline, UK) and was converted to cDNA by reverse transcription using RevertAid RT Kit (Thermo Scientific, USA) with random hexamer primer as according to manufacturer’s instruction. Briefly, 1 µg of total RNA was reverse transcribed and amplified by incubating the reaction mixture at 25°C for 5 min, followed 42°C for 60 min. The reaction was terminated by incubating the mixture at 70°C for 5 min. The cDNA was diluted 10 times using nuclease-free water and used as template in qPCR or stored at -80°C until use.
The mRNA levels of HMG-CoA reductase and AMPK were determined by real-time PCR analysis using the Agilent AriaMx Realtime PCR System (Agilent Technologies, USA). Twenty microliter of PCR reactions consisted of 10 µL of 2× SensiFAST SYBR mix (Bioline, UK), 0.8 µL each of 10 µM forward and reverse primers and 1 µL of cDNA. The primer sequences are shown in Table 1, using amplification conditions suggested by the manufacturer. The 18S rRNA gene was used as the housekeeping gene for normalization of data. The mRNA expression was expressed as percentage change relative to the control.
Target genes | Primer sequences (5’-3’) | TA (°C) | NCBI accession number or references |
---|---|---|---|
18S rRNA | F: CTC AAC ACG GGA AAC CTC AC R: CGC TCC ACC AAC TAA GAA CG |
58 | Lin et al. (2016) |
AMPK | F: GGC AGA AGT ATG TAG AGC AAT CA R: GAG TAA GTG CTT GTC ACA GGA T |
60 | NM_001355028.1 |
HMGCR | F: CCC AGT TGT GCG TCT TCC A R: TTC GAG CCA GGC TTT CAC TT |
60 | XM_011543359.1 |
The activity of AMPK was assessed using a cell-based ELISA (RayBio cell-based protein phosphorylation ELISA kit, RayBiotech, USA) according to the instructions of the manufacturer. Briefly, HepG2 cells (1×104 cells/well) were seeded in 96-well plates and incubated 24 h at 37°C and 5% CO2 in serum free DMEM. After washing with 200 µL PBS twice, 100 mL of fixative solution was added to each well and incubated for 20 min at room temperature with shaking. Then the plate was washed 3 times using 200 µL TBST and incubated with a 100 µL quenching buffer for 20 min at room temperature, followed by addition of blocking buffer for 1 h at 37°C. Next, the plate was washed 3 times using 200 µL TBST, and 50 µL of rabbit anti-phosphorylated AMPK-α1/2 (Santa Cruz Biotechnology; Thr172, 1:1,000 dilution in the blocking solution) was added and incubated for 3 h at room temperature with shaking. After washing, 50 µL of HRP-conjugated mouse antirabbit IgG (1:10,000 dilution in the blocking solution; Santa Cruz Biotechnology, USA) was added and incubated for 1.5 h at room temperature. After washing 3 times, 50 µL of TMB substrate (3,3,5,5-tetramethylbenzidine) was added to each well and incubated for 30 min with shaking at room temperature in the dark. Finally, 50 µL stop solution (H2SO4, 2N) was added, and the optical density was read at 450 nm.
Data were analyzed using SPSS version 23.0 (SPSS Inc, IL, USA). One-way ANOVA was used to study the significant differences between sample means, with a significance level at α=0.05. Mean comparisons were assessed by Duncan’s test. All data presented are mean values obtained from triplicates readings from three separate runs (n=3).
Results
Strains of lactobacilli were screened based on the cholesterol assimilation properties, with four strains (L. plantarum DR7, L. fermentum DR9, L. reuteri 8513d and Lactobacillus casei Shirota) exhibiting higher cholesterol assimilation ability compared to the control (Fig. 1; p<0.05). L. fermentum DR9, L. plantarum DR7, L. reuteri 8513d and L. casei Shirota exhibited higher reduction of total cholesterol from the culture media containing cholesterol micelle (61.6%, 64.4%, 65.3%, 72.7%, respectively) and were thus selected for subsequent analyses. L. reuteri NCIMB30242 exhibited moderate cholesterol assimilation ability (50.2%) but was also included in subsequent analyses, as this was the first commercialized probiotic strain aimed at reducing blood cholesterol level and thus served as a commercial control.
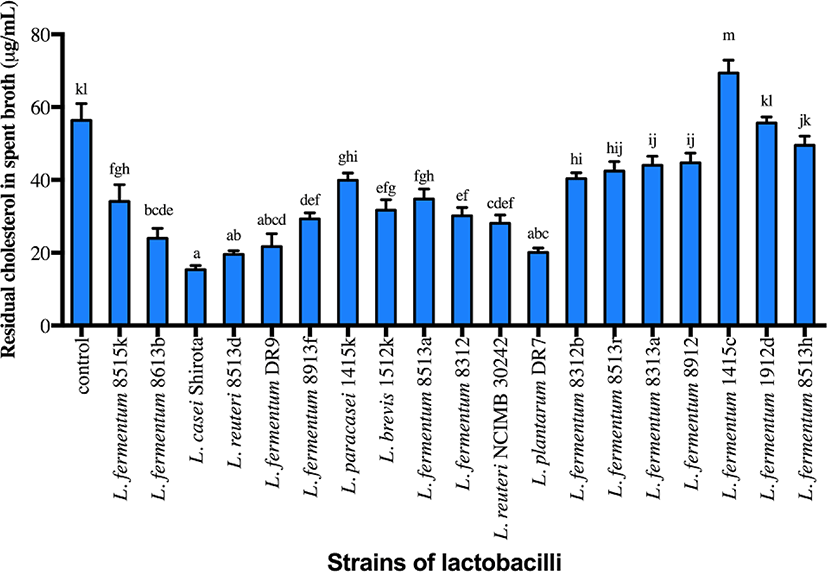
CFS of all strains except L. fermentum DR9 significantly reduced cholesterol accumulation in HT-29 cells (p<0.05) as compared to the control (Fig. 2). CFS of L. casei Shirota showed a higher reduction (36.1%) of cholesterol accumulation in HT-29 cells, followed by L. reuteri 8513d (28.5%), L. plantarum DR7 (28.0%) and L. reuteri NCIMB30242 (14.9%). Meanwhile, only CFS of L. plantarum DR7 significantly reduced (p<0.05) cholesterol accumulation in HepG2 cells (approximately 19.7%) as compared to the control. CFS of L. plantarum DR7 (CFS-DR7) reduced accumulation of cholesterol in both HT-29 and HepG2 cells, and was thus selected for further analyses.
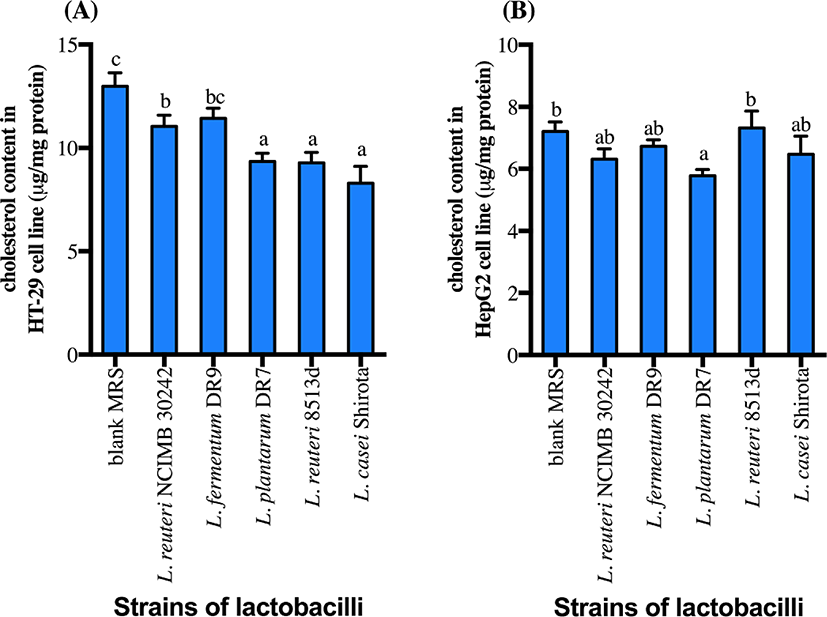
As liver is the main organ controlling cholesterol homeostasis, we further varied the concentrations of CFS-DR7 for evaluation on cholesterol accumulation and expression of HMGCR mRNA (a rate-limiting enzyme responsible for the biosynthesis of cholesterol) in HepG2 cells. Concentration tested varied from 10% to 50% (v/v), as higher concentration was not possible due to cell apoptosis at concentration above 50% (data not shown). Lovastatin (1 µM) was included as a positive control. Cholesterol accumulation in HepG2 cells was significantly reduced at all concentrations studied, with similar effects as compared to lovastatin (Fig. 3A; p<0.05). However, reduction in the gene expression of HMGCR mRNA was only significant at CFS-DR7 concentration above 30% (Fig. 3B; p<0.05). Thus, CFS-DR7 at concentration of 30% was used in all subsequent analyses.
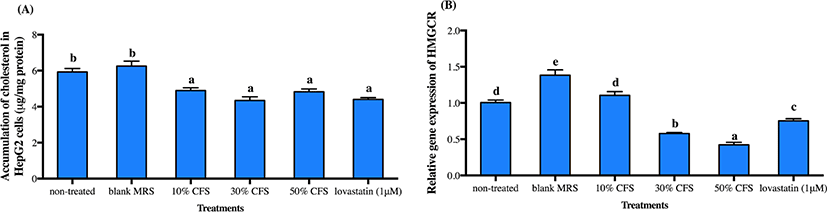
The effects of CFS-DR7 on the expression of HMGCR mRNA in the presence of an AMPK inhibitor (Compound C, 10 µM; as the negative control) and AMPK activator (AICAR, 1 mM; as the positive control) were subsequently evaluated (Fig. 4). CFS-DR7, at a concentration of 30%, significantly reduced the expression of HMGCR mRNA (p<0.05) as compared to the control, upon incubation for 3 h, at 37°C. Such a reduction was also statistically greater as compared to the positive control (1 mM AICAR; p<0.05), while lovastatin (1 µM) exerted insignificant changes on the mRNA expression of HMGCR (p>0.05). Compound C (10 µM) on the other hand, significantly increased the mRNA expression of HMGCR (p<0.05) as compared to the control. Upon treatment of HepG2 cells with CFS-DR7 in the presence of Compound C (10 µM), the mRNA expression of HMGCR was significantly higher as compared to the control (p<0.05), indicating a diminished effect of CFS-DR7.
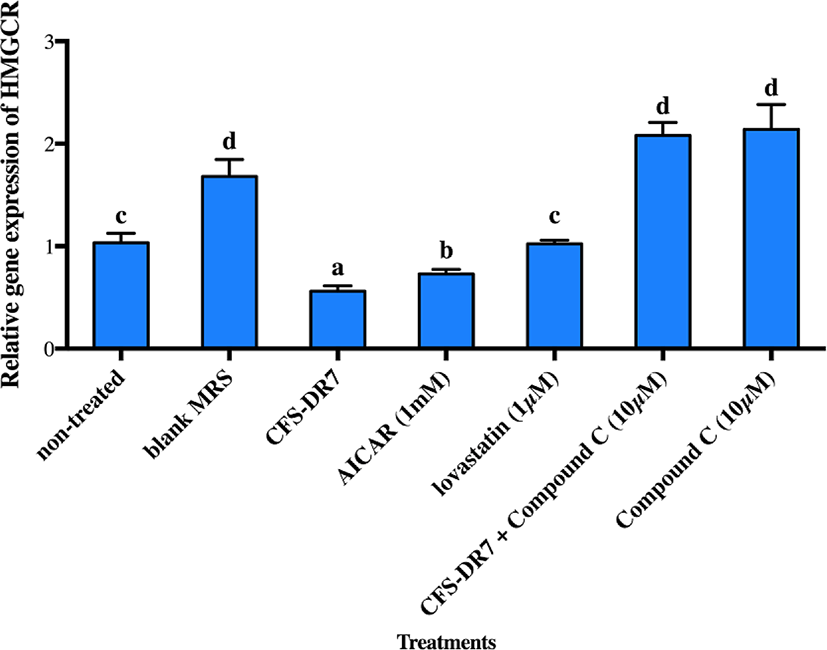
We further hypothesized that CFS-DR7 regulated the expression of HMGCR via increased expression of AMPK. However, CFS-DR7 did not affect the expression of AMPK mRNA (Fig. 5), indicating that the regulation was beyond a transcriptional level. Thus, we further investigated the effect of CFS-DR7 on the phosphorylation of AMPK. In general, phosphorylation of AMPK leads to activation of the enzyme, while dephosphorylation inactivates it. Our current data showed that CFS-DR7 significantly increased (p<0.05) the phosphorylation of AMPK to a similar level as the well-established AMPK activator (AICAR; Fig. 6), while the addition of AMPK inhibitor (Compound C) diminished such an effect. CFS-DR7 also significantly increased (p<0.05) the phosphorylation of AMPK to a level higher than lovastatin (1 µM).
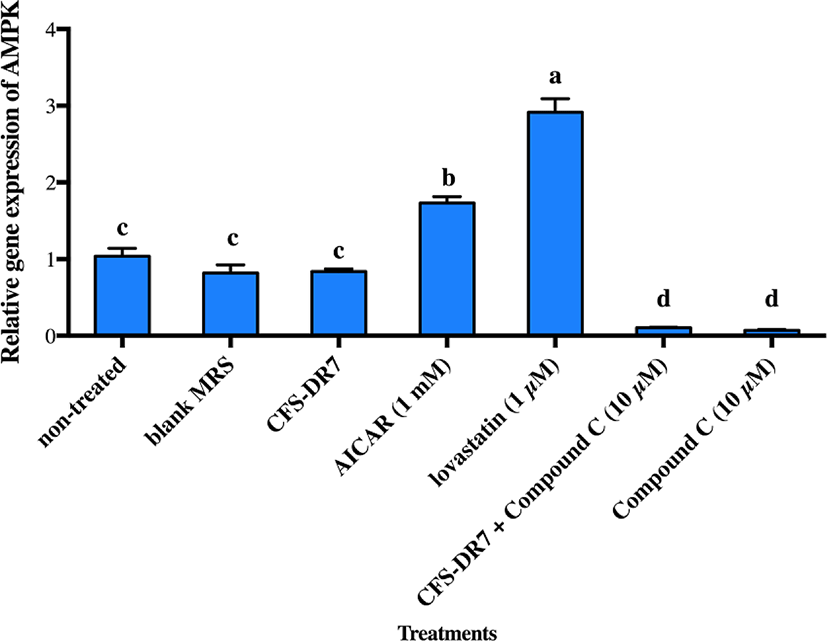
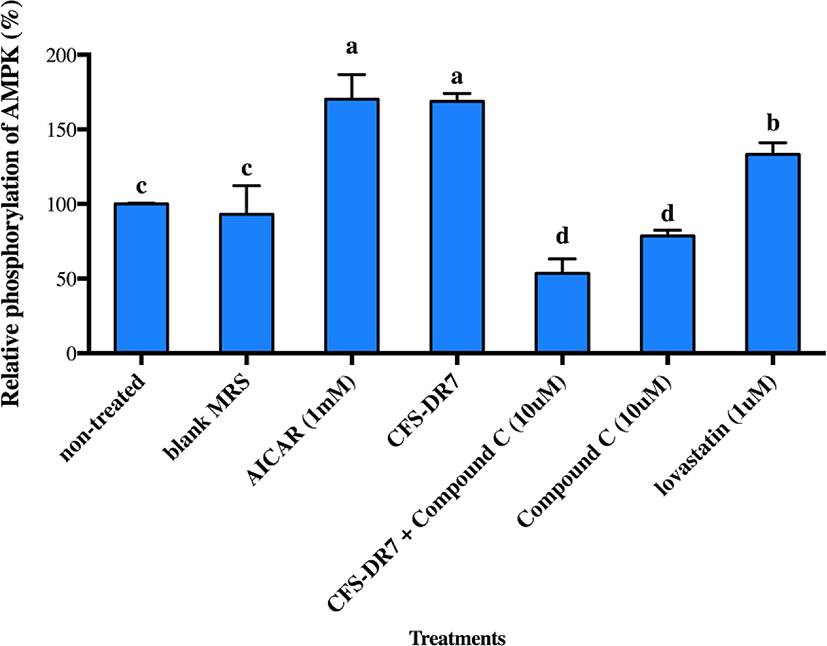
Discussion
The increasing interests on probiotics have expanded beyond human nutrition, to envelop agriculture applications as well. The incorporation of probiotics in animal feed have been reported to improve digestion, growth rate, product yield, host immune system, and reduced carcass contamination and mortality/morbidity (Park et al., 2016). Changes in livestock feed have also resulted in significant modification of consumer health (Weill et al., 2002).
Considering this, and the fact that probiotics have exerted various benefits beyond gut to comprise metabolic disorders including hypercholesterolemia, various efforts have emphasized on the efficacy of lactobacilli in regulating lipid profiles in both animals and human (Jones et al., 2012a; Jones et al., 2012b; Liong et al., 2007; Ooi et al., 2010a; Ooi et al., 2010b). Various mechanisms have been postulated, including deconjugation of bile acids by bacterial BSH activity, assimilation of cholesterol by live probiotic cells, absorption of cholesterol onto cellular surface of both live and dead probiotics cells, and alteration of lipid metabolisms via changes in roles of lipid transporters. Screening for in vitro cholesterol-lowering properties has become an important criterion in the selection of bacterial strains for in vivo probiotic investigations. It has been reported that the assimilation of cholesterol by lactobacilli in the small intestine could reduce intestinal absorption of cholesterol leading to cholesterol-lowering effects (Pigeon et al., 2002). In this study, strains were screened based on the ability to assimilate cholesterol. L. fermentum DR9, L. plantarum DR7, L. reuteri 8513d and L. casei Shirota were able to reduce above 60% of total cholesterol from the culture media containing cholesterol micelle. We have previously reported that the removal of cholesterol from growing media could be attributed to the incorporation of cholesterol into the cellular membrane and conversion of cholesterol to coprostanol (Lye et al., 2010).
Hypercholesterolemia is a metabolic disorder that is primarily affected by dietary factors and lipid metabolism factors of the host. Thus, the modulation of key intestinal cholesterol transport pathways and homeostasis of cholesterol in the liver are crucial factors that need to be evaluated to better understand hypercholesterolemia (Michael et al., 2017; Kim et al., 2014, Huang et al., 2014). In this present study, CFS of selected lactobacilli strains significantly reduced the amount of cholesterol accumulation in both intestinal and liver cells, with a more predominant effect from L. plantarum DR7. Lactobacilli have been reported to inhibit the expression of the cholesterol uptake transporter NPC1L1 in human intestinal epithelial cells and decreased the extracellular cholesterol uptake together with increased expression of apical cholesterol efflux proteins ABCG-5 and ABCG-8 (Michael et al., 2016).
To better understand possible targets and the mechanisms involved, we evaluated the expression of HMGCR, a rate limiting enzyme responsible for de novo synthesis of cholesterol in the liver (Hu et al., 2013, Chen et al., 2016). The CFS of L. plantarum DR7 significantly down-regulated the expression of HMGCR in human hepatoma cells, HepG2 at a concentration 30% (v/v) and above. While HMGCR has been reported as the most targeted sites for regulation of blood cholesterol, it is worth noting that AMPK, an energy sensor that play a role in sustaining cellular energy levels, has emerged as a potential therapeutic target for metabolic diseases. AMPK is the upstream kinase for the critical metabolic enzymes such as HMGCR and is activated by phosphorylation of a threonine residue within the activation loop segment of the α subunit (Thr172 in rat AMPK) (Hawley et al., 1996). Once activated, AMPK phosphorylates a broad range of downstream targets, resulting in the overall effect of increasing ATP-producing pathways whilst decreasing ATP-utilizing pathways (Carling et al., 2012). Mechanistically, AMPK directly phosphorylates and controls activity of numerous key metabolic regulators and transcription factors predominantly involved in control of glucose and lipid metabolism (Srivastava et al., 2012).
Considering that an effect on HMGCR was observed, and HMGCR is regulated by AMPK in the upstream, we further evaluated the possible effects of our lactobacilli CFS against AMPK. We did not observe significant changes in the gene expression of AMPK upon incubation with the CFS of L. plantarum DR7 suggesting that the regulation was beyond a transcriptional level. While gene expression studies revealed information on the AMPK mRNA transcript, studies on post-translational modification have indicated that phosphorylation eventually activates AMPK. In this study, we showed that CFS- DR7 at a concentration of 30% significantly increased the phosphorylation of AMPK, to a level similar to the well-established AMPK activator (AICAR), while the addition of an AMPK inhibitor (Compound C) diminishes such an effect. The level of phosphorylation was also higher than that exerted by lovastatin. Lovastatin, the first approved inhibitor of HMGCR, has also been previously reported to induce AMPK activation similar to metformin, a known inducer of this pathway (Ma et al., 2012). In this study, we are illustrating that the effects of cholesterol lowering properties by L. plantarum DR7 was feasible along the AMPK pathway, typically via phosphorylation of AMPK, leading to a reduced expression of the HMGCR gene. AICAR (5-aminoimidazole-4-carboxamide 1-b-d-ribo-furanoside) is transferred into the cell by the adenosine transporter and metabolized by adenosine kinase to 5-amino-imidazole-4-carboxamide 1-b-d-ribofuranosyl monophosphate (ZMP), an AMP analog that activates AMPK (Rattan et al., 2005). Meanwhile, Compound C (6-[4-(2-Piperidin-1-yl-ethoxy)-phenyl)]-3-pyridin-4-yl-pyrrazolo[1,5-a]-pyrimidine) is a cell-permeable pyrrazolopyrimidine agent that acts as a potent ATP-competitive inhibitor of AMPK (Zhou et al., 2001).
It was previously shown that the administration of Lactobacillus plantarum fermented soy milk increased phosphorylation of AMPK in the liver of rat models; however, it was unclear whether the effect was attributed to fermentation by Lactobacillus plantarum, as no significant difference in the phosphorylation of AMPK was observed as compared to non-fermented soy milk (Kim et al., 2014). Lactobacilli have also been reported to reduce the HMGCR mRNA expression in HepG2 cells, via another pathway related to NF-KB (Nuclear Factor-kappaB) (Chen et al., 2016). Although the NF-κB subunits are not directly phosphorylated by AMPK, but the inhibition of NF-κB signaling is mediated by several downstream targets of AMPK (Salminen et al., 2011). Here, we are reporting an alteration of cholesterol metabolism using CFS from lactobacilli that targeted a pathway involving the phosphorylation of AMPK. Considering that hypercholesterolemia is a metabolic disorder that is complex and affected by multifaceted factors, it is crucial to understand all possible factors that are involved. Each host could harbor various factors affecting lipid metabolism and thus, a better understanding and continuous discoveries of new pathways will pave the fundamentals for the development of new dietary interventions.