Introduction
For decades, Listeria monocytogenes has been one of the most studied foodborne pathogens, as it causes the severe human illness listeriosis (Low and Donachie, 1997; Schuppler and Loessner, 2010). This microorganism has been repeatedly isolated from both food and the environment, and it contaminates a wide variety of dairy products (Koch et al., 2010; Latorre et al., 2010; Massa et al., 1990; Pintado et al., 2005). It is also frequently present in the guts of cattle, poultry and pigs and can be transferred to ready-to-eat foods or raw meat products (Meyer-Broseta et al., 2003; Pak et al., 2002; Ryser et al., 1996). This bacterium can grow at low temperatures and is relatively tolerant of salt and low pH, rendering its control in foods difficult (Halimi et al., 2010).
To date, numerous studies have investigated the control of L. monocytogenes contamination in foods through use of antimicrobials either in formulations or external applications as dips or sprays (Barmpalia et al., 2005; Byelashov et al., 2008; Kim et al., 2015; Lee et al., 2017; Yoon et al., 2009). One promising option for the control of L. monocytogenes is application of lactic acid bacteria (LAB) or their products bacteriocins, which are safe to consume and are also inhibitors of pathogens. Bacteriocins are proteinaceous antimicrobial compounds, and most bacteriocins studied are from the LAB strains that are commonly associated with food, especially fermented dairy and meat products (Halimi et al., 2010; Lecompte et al., 2008; Mathur et al., 2017). Thus, bacteriocins or bacteriocin-producing LAB with anti-listerial activity have been employed to inhibit L. monocytogenes in foods. Marques et al. (2017) reported that film containing a bacteriocin-like substance from Lactobacillus curvatus P99 effectively controlled L. monocytogenes in sliced cheese. In addition, the combination of a cell-bound bacteriocin produced by Lb. curvatus CWBI-B28 with savory essential oils or oregano effectively reduced the number of viable L. monocytogenes in pork meat (Ghalfi et al., 2006).
Although bacteriocins with anti-listerial activity have been isolated from a wide variety of LAB species, including Lactobacillus spp. (Han et al., 2007; Larsen et al., 1993; Xiraphi et al., 2006), Enterococcus spp. (Feng et al., 2009; Seo et al., 2014), Streptococcus spp. (Heng et al., 2007), Leuconostoc spp. (Felix et al., 1994) and Weissella spp. (Papagianni and Papamichae, 2011), little information is available on those from Leuconostoc lactis, a major heterofermentative bacterium that produces diacetyl and exopolysaccharides in dairy foods (Saravanan and Shetty, 2016). In this study, we isolated and characterized a bacteriocin from Leuc. lactis SD501 with strong inhibitory activity against L. monocytogenes.
Materials and Methods
Strains isolated from kimchi were grown in MRS broth (BD Biosciences) at 37℃. L. monocytogenes ATCC 19114 was grown in tryptic soy broth (BD Biosciences) at 25℃. Other indicator strains were grown in the media listed in Table 1.
* Activity is expressed based on the diameter of the inhibition zone around the well. Degrees of inhibition around the well: −, no inhibition; +, up to 5 mm; +++, up to 20 mm.
ATCC, American Type Culture Collection; CCARM, Culture Collection of Antimicrobial Resistant Microbes; KCCM, Korean Culture Center of Microorganisms; KCTC, Korean Collection for Type Culture; KFRI, Kerala Forest Research Institute; KVCC, Korea Veterinary Culture Collection; TSB, tryptic soy broth; NB, nutrient broth; LB, Luria-Bertani; PDA, potato dextrose agar.
Genomic DNA from the bacterial strains was extracted and purified. Then, 16S rDNA was amplified via polymerase chain reaction (PCR) using the PCR Thermal Cycler Dice® Gradient (TaKaRa, Japan). The universal primers used for PCR amplification were 5'-AGAGTTTGATCCTGGCTCAG-3' (27F) and 5'-TACGGTTACCTTGTTACGACTT-3' (1492R) (Pavlova et al., 2002). Amplified 16S rDNA fragments from strain SD501 were visualized by 1% agarose gel electrophoresis. Then, the PCR products were purified using a commercial PCR cleanup kit for the purification of sequencing fragments (MACHEREY-NAGEL GmbH, Germany). The sequencing of the 16S rDNA fragment was performed by Cosmogenetech Co., Ltd. (Korea). A homology search was carried out using the NCBI BLAST search program (http://blast.ncbi.nlm.nih.gov/Blast.cgi).
LAB strains were isolated from kimchi and screened for their antagonistic activity against L. monocytogenes via the agar well diffusion assay (Lim et al., 2016). Briefly, isolates were grown in MRS broth at 37℃ for 24 h, and then cells were removed by centrifugation (8,000×g for 10 min at 4℃). The cell-free supernatant was adjusted to pH 7.0 using 3 N NaOH and sterilized using a disposable syringe filter (0.45 μm pore size; BioFACT, Korea) prior to the antimicrobial activity assay. Indicator strains (0.1%, v/v) were added to soft agar medium (0.75% agar) and mixed well. The agar plates were allowed to solidify, and the wells were punched into the agar plates using a sterile glass cylinder. These wells were filled with 200 μL cell-free supernatant from the isolates, and then the plate was incubated at the appropriate temperature for the indicator strains. Antimicrobial activity was detected based on the presence of a clear zone around the wells. All bacteria tested are shown in Table 1.
Bacteriocin production during bacterial growth was monitored in 250 mL Erlenmeyer flasks inoculated at 1% (v/v) with an overnight culture of strain SD501 incubated at 37℃. Samples were collected at 3- to 12-h intervals during a 72 h incubation period, and cell growth and antimicrobial activity were measured. Cell growth was represented by optical density (OD) measurements at 600 nm using a spectrophotometer (Shimadzu, Japan), and the antimicrobial activity of the cell-free supernatant after neutralization was expressed as arbitrary activity units (AU) per mL. One AU was defined as the reciprocal of the highest dilution that showed inhibition of growth of L. monocytogenes ATCC 19114, the indicator strain, in the agar well diffusion method described above. All experiments were performed in triplicate. The titer was measured using the formula AU/mL=[2x]×[1,000/V (μL)], where X is the number of the final dilution showing inhibition, and V is the volume of bioactive liquid (Chumchalova et al., 1995).
Anti-listerial bacteriocin purification was carried out via a three-step procedure using the supernatant of a 4 L culture of Leuc. lactis SD501 grown to the early stationary phase in MRS broth at 37℃. First, ammonium sulfate was gradually added to the cell-free supernatant (70% saturation) of Leuc. lactis SD501, which was stirred for 12 h at 4℃ and then centrifuged (8,000×g for 30 min at 4℃). The pellet was resuspended in 50 mM Tris-Cl buffer (pH 7.0) and dialyzed using dialysis membrane (Pre-wetted RC tubing [MWCO: 1 kD], Spectra/Por®, USA). The dialyzed product was mixed with Amberlite XAD-16N resin (Sigma-Aldrich, USA) that had been previously activated and equilibrated with 50% (v/v) isopropanol, and the substance was incubated for 12 h at 4℃ to allow to adsorb onto the resin. The matrix was packed into a column (1.2×30 cm, SciLab®, USA) and washed twice with washing buffer (40% ethanol). The active solution was recovered using elution buffer (70% 2-propanol and 10 mM acetic acid, pH 2.0).
The Sep-Pak C18 cartridge column (Millipore, USA) was initially rinsed and equilibrated using 100% acetonitrile supplemented with 0.1% trifluoroacetic acid. The equilibrated column was washed using distilled water containing 0.1% trifluoroacetic acid. The active solution was loaded onto a C18 cartridge column and eluted as an antibacterial fraction with acetonitrile containing 0.1% trifluoroacetic acid. Then, it was dried using a vacuum concentrator (Savant spd1010, Thermo Scientific, USA) to remove the solvent and finally dissolved in 50 mM Tris-Cl buffer (pH 7.0) for determination of the molecular weight of the active substance. The activity and protein concentration of the purified anti-listerial substance were determined using the serial twofold dilution method and Bradford protein assay (Bradford, 1976), respectively, and antimicrobial activity was assessed using the agar well diffusion assay described above (Table 2).
The substance purified from the SD501 culture supernatant was treated with the following enzymes, each at a final concentration of 5 mg/mL: α-amylase, α-chymotrypsin, lipase, proteinase K, pepsin and trypsin (all from Sigma). All preparations were incubated for 2 h at the appropriate temperatures, and residual antimicrobial activity against L. monocytogenes ATCC 19114 was examined using the agar well diffusion assay.
The effect of pH on the anti-listerial substance was evaluated by adjusting the purified substance to pH 1.0–10.0 (at increments of one pH unit) with sterile 3 N NaOH or 3 N HCl. After a 2 h incubation at 37℃, the samples were re-adjusted to pH 7.0 with 3 N NaOH or 3 N HCl and evaluated for anti-listerial activity using the agar well diffusion assay.
To determine the effect of temperature on the anti-listerial substance, the substance was subjected to 4℃, 25℃, 37℃, 50℃, 60℃, 80℃, 100℃, and 121℃. Residual anti-listerial activity was tested after a 15 min incubation at these temperatures using the agar well diffusion assay.
The molecular weight of the partially purified anti-listerial substance was determined using a tricine sodium dodecyl sulfate–polyacrylamide gel electrophoresis (SDS-PAGE) system, as described by Schägger and von Jagow (1987), using 4% acrylamide for the stacking gel, 10% acrylamide for the spacer gel and 16.5% acrylamide for the separating gel. The sample was mixed with sample buffer without SDS and boiled at 100℃ for 10 min. Electrophoresis was performed using vertical gels (1.5 mm thickness) in an electrophoresis chamber (PowerPac 300, Bio-Rad, USA) at 30 V for 12 h. After electrophoresis, one gel was placed in staining buffer (0.025% (w/v) Coomassie Brilliant Blue G-250 (Bio-Rad, USA), 10% (v/v) acetic acid, 45% (v/v) distilled water and 45% (v/v) methanol for 1 h, then destained with destaining buffer (10% (v/v) acetic acid, 45% (v/v) distilled water and 45% (v/v) methanol for 24 h. The other gel was fixed using destaining buffer, and then washed three times in distilled water for 3 h and then overlaid with 0.75% (w/v) soft agar inoculated with L. monocytogenes ATCC 19114 (0.1%, v/v). The plate was incubated at 25℃ overnight, and the gel was examined for an inhibition zone.
Results and Discussion
Presumptive LAB grown on MRS agar plates were selected, and their neutralized cell-free supernatants were evaluated for antimicrobial activity against L. monocytogenes ATCC 19114 using the agar well diffusion assay. Of 140 strains tested in this study, strain SD501 showed strong anti-listerial activity and was selected for further investigation. The 16S rDNA sequence of strain SD501 showed the most similarity (100%) to that of Leuc. lactis CAU7596 (data not shown). Therefore, this strain was confirmed to belong to the species Leuc. lactis and was designated Leuc. lactis SD501. The sequence determined in this study has been deposited in the NCBI database under accession number MF108705.1. To date, anti-listerial substances from Leuc. lactis strains have been reported by Cholakov et al. (2017) and Yehia et al. (2017). However, their studies lack data on the characteristics of the anti-listerial substances.
The relationship between the cell growth stage and anti-listerial activity of Leuc. lactis SD501 was determined (Fig. 1). Anti-listerial activity was first detected at 3 h, when the culture was in the early logarithmic phase. Anti-listerial activity reached a maximum (735 AU/mL) during the early stationary phase (9 h) and then decreased gradually, as previously reported for most bacteriocins from LAB (Arakawa et al., 2016; Chen et al., 2018; Makhloufi et al., 2013; Wulijideligen et al., 2012; Zamfir et al., 2016). Decreased antimicrobial activity after prolonged incubation of the bacteria could be attributed to processes such as proteolytic degradation by extracellular proteases, re-adsorption by the surface of the producing cell, or protein aggregation (Papagianni and Papamichae, 2011).
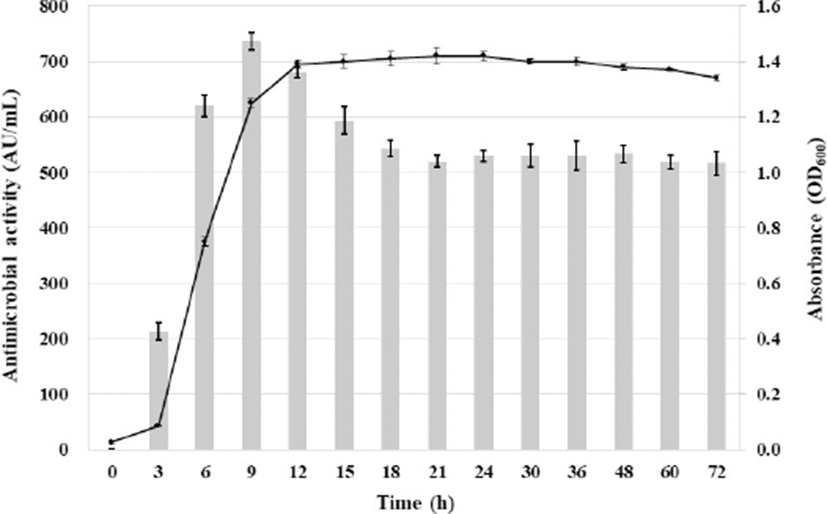
The antimicrobial spectrum of the purified substance from Leuc. lactis SD501 is shown in Table 1. The agar well diffusion assay showed that the antimicrobial substance from Leuc. lactis SD501 exhibited a narrow antimicrobial spectrum. It was particularly potent against L. monocytogenes and also inhibited Enterococcus faecalis. On the other hand, it was not active against any other Gram-positive or Gram-negative bacteria evaluated.
The effects of physicochemical treatments on the stability of the purified anti-listerial substance from Leuc. lactis SD501 are summarized in Table 3. The anti-listerial substance was sensitive to several proteolytic enzymes (proteinase K and ɑ-chymotrypsin), confirming its proteinaceous nature. However, it was resistant to catalase and amylase, indicating that carbohydrates are not bound to the substance, and its antimicrobial effect was not derived from hydrogen peroxide.
The effect of pH on the anti-listerial substance was assessed after adjusting the pH from 1.0 to 10.0 for 2 h and then re-adjusted to pH 7.0. The antimicrobial activity remained stable at pH values ranging from 1 to 10. Its activity was even increased after adjusting to acidic conditions before neutralization. Some bacteriocins, such as nisin, showed highest activity in acidic conditions. Tuncer and Ozden (2010) reported that antimicrobial activity of nisin-like bacteriocin from Lac. lactis was highest below pH 4.0. The influence of pH on the antimicrobial activity of bacteriocin could be due to changes in the conformation/oligomerization of the bacteriocin peptide as well as to changes in the surface charge of the target microorganisms (Abriouel et al., 2001). In other words, some bacteriocins form monomeric structure than oligomeric one at low pH and show higher antimicrobial activity (Alvarez-Cisneros et al., 2010).
Furthermore, the inhibitory activity was strongly resistant to high temperatures and was retained even after incubation for 15 min at 121℃. Similar results were reported for mesentericin ST99 from Leuc. mesenteroides (Todorov and Dicks, 2004) and leucocin A from Leuc. mesenteroides (Balay et al., 2017).
Bacteriocins that are active against Listeria with a wide pH stability and good thermostability similar to those of SD501, such as leucocin (Balay et al., 2017), acidocin (Kanatani et al., 1995), mesentericin (Todorov and Dicks, 2004), pediocin (Porto et al., 2017) and sakacin (Simon et al., 2002), are classified as Class II bacteriocins among four classes of bacteriocins (Alvarez-Sieiro, 2016; Balciunasa et al., 2013).
In Tris-tricine SDS-PAGE analysis, the active fraction of partially purified Leuc. lactis SD501 showed a single band corresponding to a molecular mass of approximately 7 kDa on a gel stained with Coomassie Brilliant Blue (Fig. 2A). In post-electrophoretic detection analysis, the inhibitory activity of that band was confirmed by overlaying the bacteriocin-containing gel with 0.75% (w/w) soft agar containing the indicator strain L. monocytogenes ATCC 19114 (Fig. 2B).
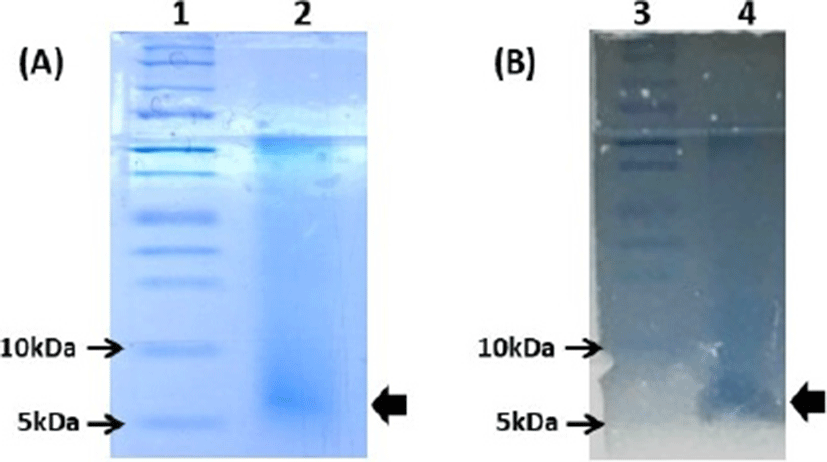
Overall, a small molecular size (<10 kDa), strong anti-listerial activity, wide pH stability and good thermostability are common characteristics of class IIa bacteriocins. Therefore, the anti-listerial bacteriocin from Leuc. lactis SD501 may be classified as a Class IIa bacteriocin, based on the accepted bacteriocin classification system (Alvarez-Sieiro, 2016; Balciunasa et al., 2013). The amino acid sequence, mode of action and genes involved in biosynthesis of SD501 bacteriocin need to be elucidated by future research.
Conclusion
Anti-listerial bacteriocin was obtained from Leuc. lactis SD501 isolated from kimchi, to our knowledge, which is the first characterized bacteriocin from the species Leuc. lactis. This bacteriocin with high thermostability and activity in a wide range of pH could be a promising biopreservative for the control of L. monocytogenes in foods, including dairy and meat products.