Introduction
Freezing has been recognized as the best preservative method to maintain the quality of foods for long-term storage. Nevertheless, frozen foods show quality deteriorations compared to fresh ones, and this is an inevitable phenomenon. The quality deterioration of frozen foods results from the tissue damage caused by ice crystal formation. To minimize the quality difference between fresh and frozen foods, it is essential to freeze foods quickly and to store them at a low temperature (Kim and Hong, 2016).
Until now, various quick-freezing techniques have been introduced and are commercially available. In the meat industry, up-to-date techniques such as pressure-shift freezing and electromagnetic freezing have been designed to freeze meat instantly, but these techniques also have limitations from technical and economical viewpoints (Choi et al., 2016; Hong and Choi, 2016; Kim and Hong, 2016). A simple method of quick freezing is to decrease the freezing temperature, and the effect of liquid nitrogen on the quality of frozen meat has been widely investigated. However, the occurrence of cold denaturation of protein and cracking on the surface of meat are disadvantages of using liquid nitrogen (Kim et al., 2015). Grujić et al. (1993) reported that meat frozen at a temperature lower than the eutectic point (<-70°C) had greater drip loss than meat frozen at a relatively higher temperature. Therefore, research regarding the relationship between the freezing conditions and quality of meat has indicated an optimum range of –18°C to –40°C, although various deep freezers in which the freezing temperature capacity ranges from –50°C to –100°C are currently available owing to technical development. According to the review of Zhou et al. (2010), –55°C was suggested as the ideal frozen storage temperature of foods.
Recently, domestic consumption of lamb has explosively increased with the popularization of Chinese restaurants. According to the report of Raes et al. (2004), lamb has an ideal fatty acid profile and has a larger amount of long chain polyunsaturated fatty acids than that of beef. This indicates that lamb is a good nutritional source for humans, however, lamb can be easily oxidized during distribution and storage. Therefore, effective preservative methods are necessary for lamb meat, and freezing is favorable to maintain the quality of lamb meat. Although numerous studies have been conducted to evaluate the quality of frozen lamb meat, these reports were focused on the influence of air blast conditions at –18°C (Muela et al., 2010; Muela et al., 2012), and there is no report on the effect of low temperature freezing on the physicochemical properties of lamb. Consequently, this study investigated the effects of deep freezing temperatures (below –50°C) and storage periods (up to 5 months) on the quality characteristics of lamb meat.
Materials and Methods
Total 77 muscles of chilled female lamb ribs (Ovis aries, 1-year old, 48-h post mortem), a product of Australia, were imported from a local supplier company (Nature’s Food Service Co. Ltd., Korea). Australian lamb was imported in refrigerated conditions and delivered by ship for 2 wk. After trimming off the visual fat and connective tissue, 25 muscles were used for visual observation and four meat strips (3×10×3 cm) from remaining each muscle were sampled and vacuum-packaged with poly-nylon pouches. The meat strips were randomly divided by five groups and two strips from each group were inserted thermocouples (k-type) in the geometric center of samples. All samples were then tempered at 2°C refrigerator prior to freezing (~3 h). In this study, chest-type deep freezers (model No. A255WD) of which storage temperature was ranged from –18°C to –60°C were kindly provided by LG electronics (Korea), while NF-400SF freezer (Nihon freezer Co., Japan) was selected to freeze and store lamb at –80°C. All chemicals used in this study were analytical grade.
Among five groups, one group (8 strips) was selected as a fresh control of which qualities were analyzed without storage. Remaining four groups (each 48 strips) were respectively frozen and stored for 5 months at four temperatures which were classified by normal freezing (–18°C), deep freezing (–50°C and –60°C) and eutectic freezing treatment (–80°C). During freezing, temperature profiles were recorded by connecting thermocouple to a data logger (Yokogawa, Japan). After storage, 8 strips of each treatment were thawed in a 4°C refrigerator, until the core temperature of the meat reached 0°C. Phase transition time was defined by the time from initial freezing point (–1.2°C) to –5°C in the geometric center of the sample (Yang et al., 2014).
At a given experiment day, 8 strips of each treatment were randomly selected and thawed to determine thawing loss and color. Among them, five strips were cooked to determine cooking loss and texture profile analysis. The remaining three strips were ground together and used for water-holding capacity (WHC), thiobarbituric acid reactive substances (TBARS) and total volatile basic nitrogen (TVBN) analyses.
Thawing loss was determined by measuring the weight loss before freezing and after thawing, and expressed as a percentage relative to the initial weight:
where W1 was the initial weight (before freezing) of the sample (g), and W2 was the weight of sample after thawing.One gram of the minced sample was wrapped in gauze, subsequently placed into a conical tube, and centrifuged at 3,000×g for 10 min at 4ºC. The WHC of samples was calculated as the ratio of the water remaining after centrifugation to the initial water content of the sample, using the following formula:
where W1 is initial water content of the sample before centrifugation, and W2 is the difference in the water content of the sample after centrifugation.The samples were vacuum-sealed using plastic bags and then cooked in a constant 80ºC water bath for 30 min. Then, samples were tempered at ambient temperature for 30 min. The samples were weighed before and after the heat treatment. Cooking loss of lamb was expressed as percent weight loss over initial weight, calculated as follows:
where W1 is weight of the sample before cooking and W2 is weight of the sample after cooking.For texture analysis, the samples used for measuring cooking loss were cut into 10×10×10 mm cubes. Texture was measured by a CT3 texture analyzer (Brookfield Engineering Labs Inc., USA), and the test parameters were set to: TA3/100 probe, TA/SBA fixture, test speed 2 mm/s, trigger load 5 g, and compression 70%, based on Caine et al. (2003).
Color parameters were determined using a CR-400 Chroma Meter (Konica Minolta sensing, Inc., Japan) directly on the lamb surface. The results are expressed as L*, a*, and b* values representing lightness (L*), redness (a*), and yellowness (b*). The color difference (ΔE) was calculated as follows:
where L1, a1, and b1 are values of the control and L2, a2, and b2 are the values after thawing. To observe the appearance, the frozen samples were placed on a matte black background and photographed using a camera (EOS 100D, Canon, Japan).Lipid oxidation is an important factor involved in meat rancidity, which can be quantified by the thiobarbituric acid reactive substances (TBARS) following a modified method based on Witte et al. (1970). Five grams of sample was diluted with 45 mL distilled water and blended for 60 s on high speed. The mixture was filtered through Whatman No. 1 filter paper. Filtrate (0.5 mL) was transferred to a test tube followed by addition of 4.5 mL TBA solution (0.25 N HCl, 15% TCA, and 0.375% TBA reagent). Afterwards, the tube was transferred to a water bath at 95°C for 15 min. The sample was centrifuged at 3,000×g and 4°C for 10 min. After that, 200 µL of the supernatant was pipetted into a 96-well plate. Absorbance at 535 nm was measured using a spectrophotometer (MultiskanTM GO Microplate spectophotemeter, Thermo Scientific, USA).
Total volatile basic nitrogen (TVBN) was determined according to Conway’s micro-diffusion method (Conway, 1950). Briefly, the sample (5 g) was homogenized in 20 mL of deionized water (DW) in a stomacher bag, using a slap-type homogenizer (WS-400, Hansol Tech. Co., Korea) at a speed set at 9 times/sec for 180 s. The homogenate was filtered through Whatman filter paper Number 1. Then, the filtrate (1 mL) was transferred to the outer chamber, and 1 mL of 0.01 N H3BO3 and 100 µL of Conway solution (0.066% methyl red: 0.066% bromocresol green, 1:1; using 99.99% alcohol as solvent) as an indicator were added to the inner part of the Conway dish. The Conway unit was sealed immediately after pouring 1 mL of 50% K2CO3 into the outer chamber. The dish was incubated at 37°C for 120 min. The VBN contents were determined following the addition of 0.02 N H2SO4 to the inner chamber of the Conway unit and were calculated as mg%. A blank test was conducted following the same process without adding sample.
where a is the titer for sample (mL), b is the titer for the blank (mL), f is the factor of H2SO4 (0.02 N), S is the sample weight (g), and c is the dilution ratio.
Completely randomized design was adopted to evaluate the effects of temperature and storage periods on the qualities of lamb meat. Means from three entirely repeated experiments (n=3) were analyzed by one-way analysis of variance (ANOVA) using SPSS statistical software (ver. 22.0). As a post hoc procedure, means were separated by Duncan’s Multiple range test at the significant level of p<0.05.
Results and Discussion
As expected, the freezing time of the lamb was different depending on the applied freezing temperature (Fig. 1A). The estimated phase transition time of lamb frozen at –18°C was 314 min. In general, quick freezing was defined as < 30 min to traverse the phase transition of the food center (Yang et al., 2014), hence, the –18°C treatment was classified as slow freezing. Meanwhile, the phase transition times of deep freezing treatment were 26, 16, and 10 min at –50°C, –60°C, and –80°C, respectively. It was expected that tissue damage of frozen lamb would be greater at –18°C treatment than at deep freezing treatments, which might cause quality differences between the two groups.
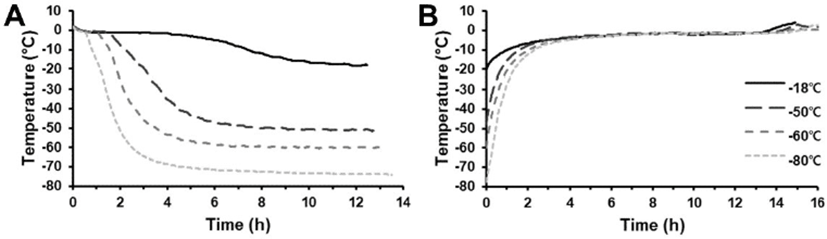
The thawing time of frozen lamb was similar among all treatments, but samples stored at lower temperatures tended to show longer total thawing times (Fig. 1B). However, phase transition time during thawing of all treatments ranged from 616–647 min, without a significant difference among treatments
As depicted in Table 1, all treatments showed 1.68%–2.67% thawing loss after 2 wk of storage, and lamb stored at lower temperatures showed significantly lower thawing loss (p<0.05). With storage, all treatments showed a significant increase in thawing loss. Particularly, thawing loss was greater when the lamb meat was stored at a higher temperature (p<0.05). In frozen meat, thawing loss is an important parameter and an indicator to predict the quality of the frozen meat. Previous reports indicated that decreasing freezing and storage temperature decreased thawing loss of meat (Kim et al., 2015; Kim and Hong, 2016), which was in accordance with this study. Conversely, Ngapo et al. (1999) reported that thawing loss of meat frozen at different rates did not change with extending storage periods, and Farouk et al. (2004) postulated that quick-freezing and very low storage temperature were not necessary. However, the former investigation adopted –18°C to –20°C storage temperature, at which the ice recrystallization would be more easily promoted compared to a lower storage temperature (Martino and Zaritzky, 1989). The latter research selected ultra-quick freezing (49.8 mm/h of freezing rate) for samples 170 mm long with 110 mm diameter. In this case, phase transition time in the meat center would take ~2 h, which was slower than that estimated in this study (26 min at –50°C). Eventually, the frozen lamb kept at lower than –50°C was highly stable during storage compared to that in the freezer maintained at –18°C.
a–e Means with different letters within the same row (storage periods) are significantly different (p<0.05).
Water holding capacity (WHC) of frozen lamb showed a similar pattern to thawing loss of lamb. Excluding –80°C treatment, all freezing treatments exhibited significantly lower WHC than 87.0% of the control after 2 wk of storage (p<0.05). With extending storage, changes in the WHC of frozen lamb showed different patterns depending on the applied temperature. Lamb stored at –18°C showed a gradual decrease in WHC with storage period and a significant decrease was shown after 3 months of storage (p<0.05). The WHC of lamb stored at < –50°C also tended to decrease with increasing storage period; however, the differences were not significant during 5 months of storage. Based on the results, it is obvious that quick freezing followed by low temperature storage (<-50°C) has the potential to minimize tissue damage of lamb meat.
Changes in cooking loss of frozen lamb showed different features depending on applied temperature. Lamb frozen and stored at –18°C tended to show high cooking loss during the storage period while the change was not significantly different. This result was normally in agreement with a previous study (Muela et al., 2010). However, the cooking loss of frozen lamb treated at a low temperature (<-50°C) was gradually decreased with storage period, and a significant difference was shown after 3–5 months depending on the applied temperature (p<0.05). The decreased cooking loss by deep freezing treatment is not clearly understood in this study.
Lamb used in this study was imported at a chilled state for 2 wk, reflecting that raw materials had an improved WHC prior to freezing because of aging (Oh et al., 2017). It is likely that the deep-freezing treatments showed an already 2.7%–6.4% thawing loss after 5 months of storage, and thus the cooking loss of frozen stored lamb might have a low cooking loss (Hong et al., 2007). Consequently, the present study demonstrated that the application of deep freezing has a potential advantage to minimize tissue damage of lamb meat, which would be beneficial to maintain the quality of frozen lamb for long storage periods.
Caine et al. (2003) and Ruiz de Huidobro et al. (2005) reported that TPA accounted for sensorial tenderness and juiciness of meat rather than Warner-Bratzler shear force. Hence, the TPA was adopted to compare the tenderness of frozen lamb stored for 5 months (Fig. 2). Hardness in normal freezing treatment (–18°C) was higher than that of the fresh control (p<0.05), while those of deep-freezing treatments were not differ from fresh control. Adhesiveness of lamb also showed a similar pattern, but the difference was not significant among treatment groups. Cohesiveness and gumminess of frozen lamb was distinctly lower than the fresh control (p<0.05), while there was no difference among freezing treatment groups. Springiness of all frozen lamb groups was greater than that of the control (p<0.05). Chewiness also showed a similar pattern to springiness, whereas lamb frozen at <-60°C showed lower chewiness than that frozen at >–50°C (p<0.05). Caine et al. (2003) reported that hardness, chewiness, and cohesiveness accounted for the meat tenderness. In this study, the effects of freezing and storage on the tenderness of lamb were highly temperature dependent. As shown in the water binding properties, normal freezing treatment (–18°C) had a high drip loss after thawing and cooking, which manifested as a film and hard texture compared to the fresh control. Although, the pattern in deep freezing treatments was similar to that of –18°C treatment, and minimizing the difference in drip loss during thawing and cooking would influence the fresh lamb-like tenderness of frozen lamb. Based on the results, deep freezing was effective to maintain textural properties of lamb, and more specifically, freezing and storage at a temperature lower than –60°C would be the optimal condition for lamb meat.
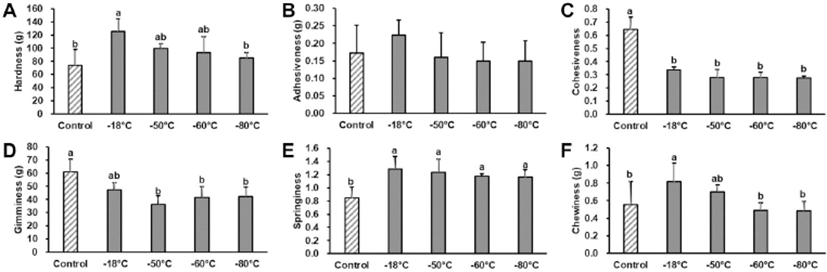
All CIE color parameters of frozen lamb were gradually decreased with storage period (Table 2). The lightness (L*) of frozen lamb did not differ from the fresh control for 5 months of storage, although deep freezing treatment tended to show a slightly lower L* than that of –18°C treatment. This would be due to the higher WHC of deep freezing treatment lamb since the moisture bound tightly with protein reflected lesser light than free water (Allen et al., 1998). The decrease in redness (a*) of frozen lamb was faster if lamb was frozen and stored at a relatively high temperature. A significant decrease in a* of frozen lamb was found after 2 months at –18°C and after 3 months at –50°C and –60°C, compared to 4 months at –80°C treatment (p<0.05). Yellowness (b*) of frozen lamb also tended to decrease with storage period, while the differences were not significant from the fresh control. The color change of lamb during storage would be the result of myoglobin oxidation, which could occur during frozen storage (Georgantelis et al., 2007). However, Yang et al. (2014) indicated that the metmyoglobin formation was greatly delayed at temperature lower than –50°C.
It was reported that oxidation of myoglobin is responsible for browning of meat during storage (Mancini and Hunt, 2005). Georgantelis et al. (2007) presented that the oxidative browning of the meat product still occurred during frozen storage, but Chin (2017) noted that the discoloration of meat was prevented at < –50°C. However, this study showed a significant difference in the color of frozen stored lamb meat, but the difference was not as high as visually detected (Fig. 3). Regardless of temperature, no frozen treatment groups showed any visualized discoloration during 5 months of storage compared to the fresh control. Consequently, the results indicated that freezing was favorable to maintain color stability of the lamb for 5 months of storage. However, it is possible that discoloration could be shown when lamb is stored for more than 5 months.
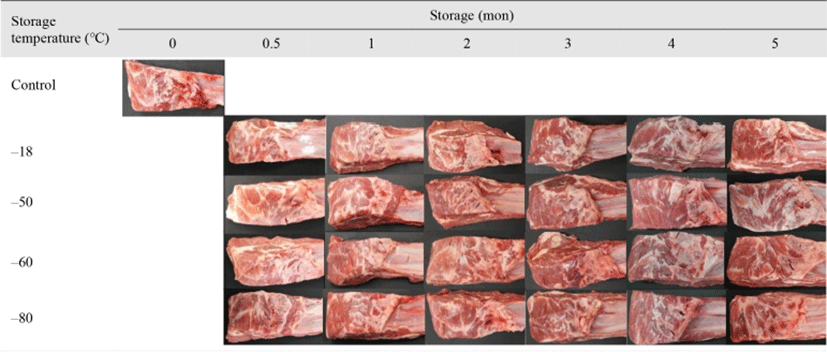
In spite of the high lipid content of lamb, lipid oxidation was effectively prevented by frozen storage for 5 months (Table 3). Initially, fresh lamb showed 0.29 mg MDA/kg, and the TBARS after freezing treatments ranged from 0.21–0.29 mg MDA/kg throughout 5 months of storage. The result was not consistent with Soyer et al. (2010), who reported that chicken meat was oxidized under frozen storage at –7°C to –18°C, regardless of temperature. The difference would be due to the storage condition (or packaging) of meat samples. In this study, lamb samples were vacuum-packaged with air-impermeable film prior to freezing, which prevented the contact of air on the surface of the lamb and resulted in stability from lipid oxidation. Because the rate of lipid oxidation is temperature-dependent, frozen storage of lamb meat would successfully inhibit the lipid oxidation and discoloration (Marie, 2015).
a–f Means with different letters within the same row (storage periods) are significantly different (p<0.05).
Meanwhile, freezing and storage temperature clearly influenced the TVBN of lamb. Comparing to 3.04 mg/100 g of fresh control, all freezing treatments showed higher TVBN (p<0.05). In particular, the higher the applied temperature, the higher the TVBN of lamb. During storage, all treatments showed a significant increase in TVBN (p<0.05), while the rate of increase in TVBN was profound when the applied temperature was high. It is known that the increase in TVBN is related to the reaction of microbial spoilage. It seems that the reaction can be generated under a temperature of <-18°C, since the increased TVBN of frozen samples has also been reported previously (Fan et al., 2009). Although the –18°C treatment showed 7.77 mg/100 g of TVBN after 5 months of storage, the value was lower than the spoiled level (35 mg/100 g) suggested by Connell (1990). Therefore, it was concluded that there was no concern about the lipid oxidation of lamb meat if the meat was not frozen, regardless of temperature, and applying a deep freezer could be a better choice to extend the freshness of lamb meat.
Conclusions
In this study, the effects of deep freezing and low temperature storage on the freshness of lamb meat were evaluated. Based on the results, it is clear that decreasing the temperature greatly improved the freshness of lamb meat. In the meat industry, decreasing the temperature for freezing and storage has been limited previously because of economic problems; however, recent advances in storage techniques have enabled the use of deep freezers for labile meats such as lamb. Still, selection of an efficient deep-freezing temperature is necessary for manufacturers and consumers, and this study demonstrated that –60°C was the optimum condition to store frozen lamb meat for 5 months without deterioration of quality and freshness.