Introduction
Calcium in bone powder exists as Ca10(PO4)6(OH)2 crystals or amorphous CaHPO4. Over 90% proteins in bone are triple-helix collagen, which is hard to be utilized by our body (Pang and Huo, 2017). By hydrolyzing, ionic calcium (Ca2+) get released from bone matrix and collagen turns into peptides. Ca2+ is an effective form of calcium that can be directly absorbed by intestinal tracts. As a bivalent mineral nutrient, Ca2+ plays key roles in maintaining the overall human health and it involves in many important physiological functions, such as bone growth, nerve conduction and muscle contraction (Bass and Chan, 2006; Guo et al., 2014). Bone collagen peptide preparations have various functions far beyond their nutritional importance, such as immune-enhancing, anti-osteoporosis, antibacterial, anti-hypertensive, antioxidant effects. It’s proved that most bioactive peptides are oligopeptides. Studies show that oligopeptides can enhance mineral absorption (Peng et al., 2017; Zhao et al., 2014; Zhang et al., 2017), and extensive hydrolysis is essential to render proteins immunologically unreactive (Cordle et al., 1991).
As a probiotic lactic acid bacteria (LAB), Lactobacillus helveticus confers a health benefit on the host (Sanders, 2008). It is suitable for dairy application and considered multifunctional. It is gaining increasing importance as a health-promoting culture in probiotic and nutraceutical food product (Giraffa, 2014). Since L. helveticus can proliferate in bone hydrolysate and secrete protease and peptidase, this might lead to a more extensive degrading of bone collagen and might generate much more oligopeptides, accompanied an increased liberating of free Ca2+. Meanwhile, many metabolites, such as vitamins, polysaccharides, organic acids produced by L. helveticus will endow SBH extra nutrients (Giraffa, 2014).
Food products with antioxidant activities are currently gaining increasing significance due to the fact that aging and many pathological processes are related to the detrimental effects of free radicals (Skrzypczak et al., 2017; Wang et al., 2014). Free radicals are highly active chemicals, accumulation of which causes oxidative stress to the body. Unfortunately, synthetic antioxidants somewhat pose potential healthy risks. Therefore, antioxidant food products derived from a natural source are extremely valuable (Skrzypczak et al., 2017).
Our preliminary study worked out a recipe to prepare SBH with high degree of hydrolysis (DH) (Han et al., 2016). The present study applied the selected L. helveticus as the suitable LAB to ferment the prepared SBH in an effort to allow more free Ca2+ and oligopeptides liberated and anti-oxidant activities enhanced through fermentation, and simultaneously harvest certain amount of viable probiotics and their beneficial metabolites in the fermented SBH. It’s expected that the resulted preparation would be rich in nutrition, with antioxidant effect and probiotic function.
Materials and Methods
L. helveticus (ATCC 15009) and Lactobacillus paracasei subsp. Paracasei (CGMCC 1.2284) are purchased from the culture collection center of China (CGMCC). Lactobacillus sakei was purified from the commercial starter Lyocarni BOM-13 composed of single bacteria strain L. sakei. Lactobacillus curvatus was isolated from the starter of Lyocarni VBL-97 composed of L. curvatus and 3 other food-grade bacterial strains. Pediococcus acidilactici was isolated from VBM-60 containing P. acidilactici, P. pentosaceus, Staphylococcus carnosus and S. xylosus. All above commercial starters were purchased from Danisco A/S, a Danish bio-based company. All LAB strains were activated in MRS broth.
Temperature (30℃, 35℃, 37℃, 40℃, 42℃, and 45℃), initial pH of MRS broth (4.0, 4.5, 5.0, 5.5, 6.0, 6.5, 7.0, 7.5, and 8.0), 1% carbon source (glucose, sucrose, fructose, lactose, soluble starch) were studied to determine the suitable protease-producing conditions for the tested LAB strains. The logarithm-stage culture of different LAB with equal OD600 was inoculated with equal volume into MRS broth (suitable pH) supplemented with 1% determined carbon source. After 24-hour incubation at the suitable temperature, the supernatant from centrifugation (8,400×g, 15 min, 4℃) was used to check the protease activity of each strain. The mixture of 450 μL supernatant and 50 μL 1% (w/v) BSA was added to 1.5 mL sodium acetate buffer (0.1 M, pH 7.0) and incubated at 37℃. The reaction was stopped by 10% trichloroacetic acid (TCA) 5 min later. Then OD280 was measured. A single unit of enzyme activity (1 U) here was stipulated as the quantity of the enzyme consumed to produce 1 μM tryptophan in one minute while hydrolyzing BSA at 37℃. The protease activity was calculated out by the equation below where K stands for the dilute times of the sample, W for the amount of generated Trp (μmol), V for the volume of the reaction system (mL), and T for the reaction time (min).
Build a system in which 12% sheep bone powder was firstly hydrolyzed by 4.25% alcalase (activity≥200 U/mg, Solarbio) at pH 9 and 45℃ for 400 min followed by adding 3.65% flavourzyme (activity≥20 U/mg, Solarbio) to the resulted mixture (adjusted to pH 6) and incubating at 55℃ for 240 min. The final mixture was autoclaved at 121℃ for 20 min. L. helveticus were gradually tamed by culturing in MRS broth with increasing proportion of SBH (supplemented with 1% glucose) from 10% to 100%. The finally tamed L. helveticus independent of MRS broth was used as the starter in the following single-factor tests and responsive surface design tests.
Polypeptides are those that less than 10 kDa and can not precipitate in 10% TCA. Samples were pretreated by adding 10% TCA and centrifugated at 5,000×g for 15 minutes. Then the total soluble nitrogen (NT, mg) and amino acid nitrogen (NAA, mg) in the supernatant were determined by traditional Lowry method and neutral formaldehyde titration method, respectively.
Obtaining rate of oligopeptide (%) was expressed as the percentage of the total soluble nitrogen in 15% TCA (Lowry method) accounting for the total nitrogen of the sample (Kjeldahl method). DH (%) can be reflected by the total number of free amino groups. In the equation below, the amount of the specific amino acid before treating and released at time t during hydrolyzing are marked as L0 and Lt, and the amount of the specific amino acid liberated after 24-hour hydrolyzing in 6 mol/L HCl at 120℃ was labeled as Lmax. The content of Ca2+ was detected by EDTA compleximetry.
The sample was fully mixed with same volume of 0.1 mM DPPH and kept in the dark for 30 min at 25℃. In the control sample volume was replaced with Milli-Q water and in the blank replaced with 95% ethanol. In the following equation, A1, A2, and A0 represent the absorbance at 517 nm of the tested sample, the blank and the control, respectively.
Hydroxyl radical (•OH) scavenging activity was determined in the light of the reported work (You et al., 2011). The reaction system consists of 600 μL of 5.0 mM 1,10-phenanthroline, 5.0 mM FeSO4, 15 mM EDTA, along with 400 μL 0.2 M sodium phosphate buffer (pH 7.4) and 800 μL 0.01% H2O2. The mixture was remained at 37℃ for 60 min and the absorbance at 536 nm was measured. •OH scavenging activity (%) was interpreted as (As–A0)×100 /(Ac–A0) where As, A0, and Ac were the absorbance value of the sample, the blank and glutathione (Sigma–Aldrich, St. Louis, MO, USA) solution in the absence of H2O2, respectively.
The scavenging activity for the radical of ABTS was assayed using the decolorization methodology (Aazam and Fatemeh, 2016). Firstly the working solution of ABTS was prepared as below. Equal volume of ABTS (14 mM) and potassium persulphate (4.88 mM) were mixed and kept at ambient temperature for 14 hours in the dark, then the mixture were diluted with 0.1 M phosphate buffer (pH 7.4) to get an absorbance of 0.70±0.05 at 734 nm. Then 200 μL working ABTS was mixed with 10 μL sample or 10 μL phosphate buffer (blank), The absorbance was detected 5 minuntes later. In the following equation, AABTS and Atest represent the absorbance of the working solution of ABTS (0.70±0.05) and the sample.
After fermentation, samples of each dilution were spread onto MRS agar plates in triplicates and cultured for 48 h. The viable counts of L. helveticus in 1 mL of crude fermented SBH were calculated.
Based on the range of the variables determined through preliminary single-factor tests, 29 tests in the central composite design (CCD) by Design-Expert version 7.0 were carried out in random order (Table 1). Data from 29 tests were analyzed using response surface methodology (RSM). Design Expert was applied for statistical analysis. Data were modeled by multiple regression analysis adopting backward stepwise analysis. radj2 was used to judge the goodness-of-fit of the regressive model. Statistical significance of the terms in the model was analyzed by ANOVA for each response.
Data were presented as mean±standard deviations. Correlation coefficient between 4 parameters in Table 5 were determined by Pearson's Correlation Coefficient Test (n=29) using IBM SPSS statistics V 21.0. Statistical significance of the hydrolysis related parameters and radical-scavenging abilities before and after fermentation were examined by analysis of variance (ANOVA) using Statistix 8.1.
Parameters | ORO | Ca2+ content | Colony count |
---|---|---|---|
Yield of polypeptides | 0.719** | 0.749** | 0.768** |
Ca2+ content | 1 | 0.810** | 0.691** |
Colony count | 1 | 0.767** |
Results and Discussion
It can be seen from Fig. 1 that peak values of protease activity (U/mL) in the crude enzyme preparations increased gradually in single-factor tests. The relative suitable conditions of L. helveticus for protease production (listed in Table 2) and thus appropriate ranges for CCD were determined. The ranges for fermenting temperature, pH, inoculation amount and incubation time were 35℃–40℃, 6.5–7.0, 2%–4%, and 35–40 h, respectively. Results also suggested that glucose is the suitable carbon source for L. helveticus to produce protease, so we supplemented 1% glucose into SBH in later fermentation studies.
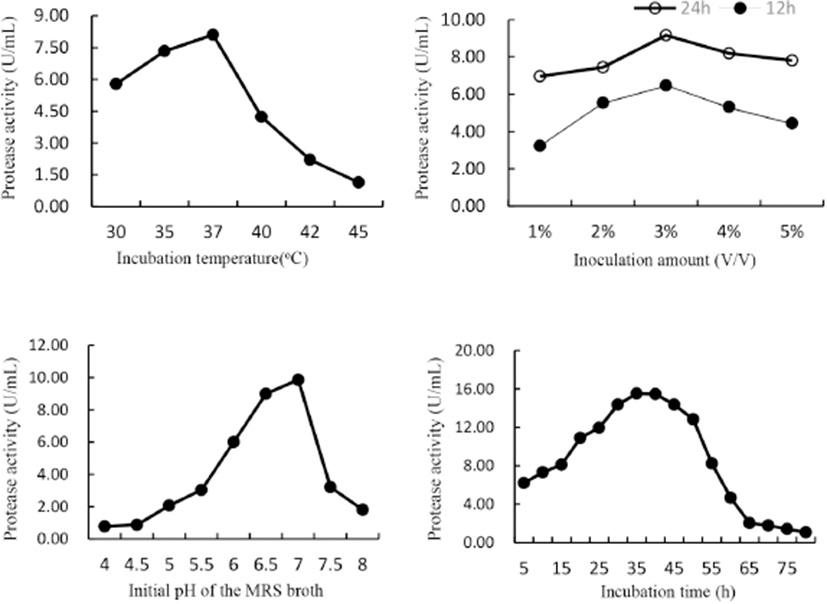
Suitable protease-producing conditions for other 4 LAB strains and the corresponding protease activities (Table 2) were also determined using the same method as L. helveticus. Since L. helveticus displayed the biggest potentiality among the 5 tested LAB strains, it was selected as the starter for SBH fermentation. Other studies also reported the high proteolytic and peptidolytic activity of L. helveticus (Nielsen et al., 2009). In milk L. helveticus developed a proteolytic system that made it thrive (Griffiths and Tellez, 2013) and can produce extracellular protease which initiated the degradation of casein into oligopeptides (Slattery et al., 2010). Various intracellular peptidases degraded peptides into amino acids, which in turn can be converted into various cell structural constituents (Griffiths and Tellez, 2013). This proteolytic system also explains why L. helveticus can thrive in SBH and liberate extraneous oligopeptides during fermentation.
Total of 29 tests listed in Table 1 were carried out to optimize the four variants in CCD, the results were showed in Table 3. By multiple regression analysis the second-order polynomial equation was obtained. Y (Ca2+)=2,011.6–62.58X1–53.5X2–37.17X3+6.08X4–170.38X12–42.76 X22 –28.51X32–75.63X42–12X1X2–74.75X1X3–28.5X1X4–47.75X2X3+63.25X2X4–33.5X3X4.
Tests of 19 combinations listed in Table 1 were performed and the results obtained were showed in Table 3 where A, B, C, and D represented total yield of polypeptide (mg/g), the obtaining rate of oligopeptide (%), ionic Ca2+ content (mg/100 mL) and viable counts of Lactobacillus helveticus (×109 CFU/mL).
Results of ANOVA analysis (Table 4) suggested that the model was significant, and the lack-of-fit showed no significance. The coefficient of the variation (CV) and radj2 were 1.03 and 0.93, respectively. All these results implied the reliability and the precision of the experimental values, as well as high consistency between the experimental and the predicted values. Table 4 also suggested that 2 linear coefficients (X1, X2), quadratic term coefficient of X22 and 2 cross coefficients (X1×X4, X3×X4) were significant.
The set of optimal conditions (pH 6.1, 3.0% inoculation volume, culture at 36.0℃ for 29.4 h) determined by RSM were also the conditions applied to verify the applicability of the model in predicting the optimal responsive values. A mean value of 19.8 mg/mL (n=3) was obtained in the verifying experiments, which was quite close to the predicated 20.3 mg/mL by the model. Therefore the model was validate and the optimal conditions obtained were reliable.
Results of correlation analysis based on the data of CCD design (n=29) suggested that all parameters were positively correlated with each other with statistical significance (p<0.01) (Table 5). It can be deduced that during fermentation, more free Ca2+ were released along with the further extensive degrading of proteose, peptone and polypeptide into more polypeptides and oligopeptides. In turn, these released peptides and Ca2+ enhanced the multiplication and the metabolism of L. helveticus. At the end of fermentation, 1.1×1010 CFU/mL L. helveticus can be detected in the fermented SBH hydrolysates.
It can be seen in Fig. 2, when SBH was fermented under the optimized set of conditions, all levels of tested parameters increased obviously (p<0.05 or p<0.01). It is necessary to be addressed that the acidic condition could facilitate the chelating reaction between peptidess and liberated Ca2+ (Han et al., 2015), and the initial pH of SBH was 6.1 in this study. It’s also reported that peptides capable of chelating minerals generated during the process of bone collagen hydrolysis (Torresfuentes et al., 2011). Ca-peptide chelate formed in SBH was verified by plane scan analysis (Han et al., 2015). So more Ca2+ and peptides were liberated from bone than the detected levels in this study and the soluble calcium in SBH or fermented SBH was existed both in free and chelated forms, this is the same in milk or dairy product which is a well accepted good source of dietary calcium (Giraffa, 2014). Since bio-fermenting by L. helveticus can further boost the liberation of free ionic calcium from bone powder, the fermented SBH would be a good calcium supplementary product with high bio-availability, and the level of calcium in it was obviously higher than milk or dairy product.
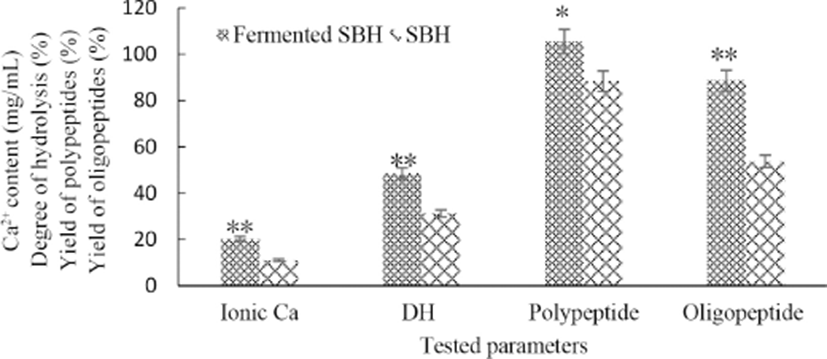
DH can be considered as the percentage of the hydrolyzed bonds of peptide. After fermentation, DH increased significantly (p<0.01), this indicated an incomplete and limited proteolysis by protease during SBH preparation and more new peptide bonds were destroyed during later fermentation, which inevitably led to a notable lifted polypeptides (p<0.05) and oligopeptides content (p<0.01) in the final fermentation. These changes were the results of metabolic activities of L. helveticus and their proteolytic and peptidolytic actions on the proteose, peptones and longer peptides that alcalase and flavourzyme unnable to further hydrolyze (Elfahri et al., 2014).
DH was a widely accepted parameter in evaluating the functional activities of protein hydrolysate (Kristinsson and Rasco, 2000). The increased DH will inevitably affect the length and the amino acid sequence of peptides (Jamdar et al., 2010). Since the size and the composition of peptides were directly related to their bioactive activities (You et al., 2011), the biological activity of SBH were unavoidable altered. This was evidenced by many studies, for instance, the antioxidant activity of porcine blood plasma protein hydrolysate and peanut protein hydrolysates increased with the increase of DH (Jamdar et al., 2010; Liu et al., 2010).
In this study, as shown in Fig. 3, compared to non-fermented SBH, both DH and the antioxidant ability of fermented SBH to scavenge DPPH (p<0.05), hydroxyl radical (p<0.01), ABTS radicals (p<0.01) were notably increased. In addition to changes in quantity, amino acid composition and the length of the peptide, metabolites such as extra-cellular polysaccharides secreted by L. helveticus, free amino acids liberated during fermentation might contribute to this increased extra antioxidant ability. Free radical-scavenging activity of milk and skimmed milk powder solution were also notably increased when fermented by L. helveticus (Rong et al., 2017). So fermentation by L. helveticus can further increase the anti-oxidant activity of the food matrix.
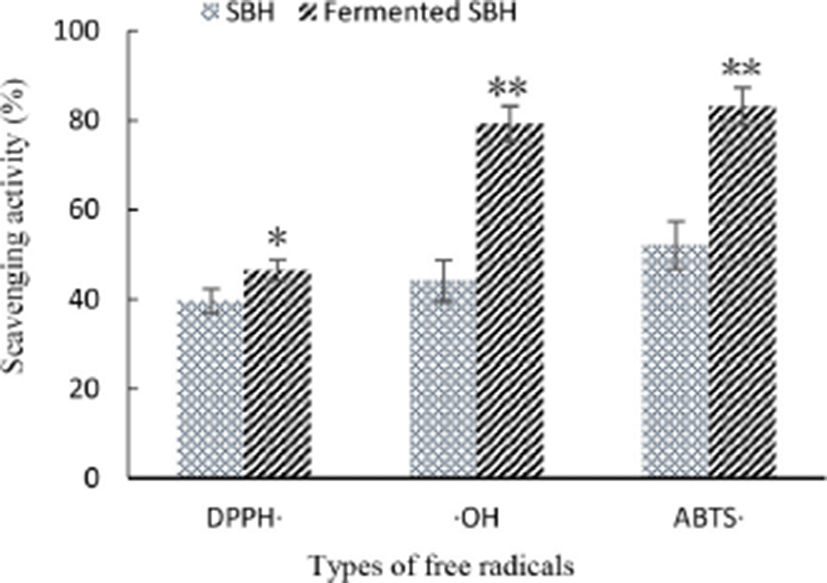
Conclusion
L. helveticus fermentation can further increase the DH, promote the further release of oligopeptide and free Ca2+, hence boosted the bio-availability and the nutritive value of SBH. The increased DH of SBH by L. helveticus fermenting caused an elevated anti-oxidant activity, accompanied by 1.1×1010 CFU/mL viable counts of L. helveticus. Hence the fermented SBH is both probiotic, nutraceutical and functional, which can be developed as a dietary “antioxidants+Ca+probiotic” supplementary product.