Introduction
Intestinal microbiota plays a crucial role in the health of the host from humans to animals. The intestinal microbiota consist of about 1,000 different microbial species, and the intestinal tract are microbial habitats with a high population density (Qin et al., 2010; Tannock, 2001). The composition and metabolic activity of microorganisms in the intestines are affected by diet, age, stress and antibiotics et al. (Biagi et al., 2010). Furthermore, intestinal flora provides nutrient substrate, affects the immune system, and prevents the multiplication of intestinal pathogens (Hooper et al., 2001). The regulation of human intestinal flora has attracted considerable scientific and commercial interests, and probiotics have been widely used to improve intestinal health.
Probiotics are live microbial additives that beneficially influence the host by maintaining the balance of healthy intestinal microbiota (Fuller, 1989). Probiotics are known to improve intestinal health through a variety of mechanisms, including intestinal pathogen displacement, production of antimicrobial substances, and immune modulating response (George Kerry et al., 2018; Lee et al., 2003). The most commonly used probiotics are lactic acid bacteria such as Bifidobacterium and Lactobacillus. They are also known to be representative beneficial intestinal bacteria (Holzapfel et al., 1998).
Bifidobacterium are gram positive, anaerobic, non-spore-forming, and non-motile bacteria (Prasanna et al., 2014). They play an important role in humans and account for about 3% to 7% of adult microbial bacteria (Lahtinen et al., 2011). They are known to possess many function such as production of antimicrobial agents, reduction of serum cholesterol level, synthesis of vitamin B, enhancement of immune system, and anti-carcinogenic activity (Martinez et al., 2013). Bifidobacterium also improves intestinal health by controlling the microbial activity in the gastrointestinal tract (Marteau et al., 2002). It was reported that the intake of Bifidobacterium infantis aids in the treatment of irritable bowel syndrome (O'Mahony et al., 2005). Furthermore, Lee et al. (1999) found that intake of B. longum HY8001 by healthy people improved their intestinal microbial composition and had a protective effect against colon cancer (Lee et al., 1999).
Composition and metabolic activity of intestinal microbiota affect intestinal SCFAs. SCFA is an essential component of bowel health and is associated with a variety of health conditions (Gibson and Roberfroid, 1995). In addition to functioning as an energy source, SCFA improves the risk of metabolic syndrome, promotes intestinal motility, regulate immune system and reduces plasma cholesterol (Hemalatha et al., 2017). It is known that lactic acid bacteria (LAB) such as Bifidobacterium and Lactobacillus participate in the production of SCFA (den Besten et al., 2013). Several studies demonstrated the effects of LAB on the intestinal health in companion dogs (Baillon et al., 2004; Biagi et al., 2007; Strompfova et al., 2014). In this experiment, changes in intestinal LAB and SCFA were simultaneously analyzed.
Cheese is a good source of protein, calcium, and vitamins for dogs. Even if cheese has a bad reputation because of high energy or salts, eating small amounts of cheese shouldn’t be a problem in healthy dogs. The majority of cheeses is safe for the majority of dogs. Queso Blanco cheese is one of the easier cheeses to make and does not requires careful handling. Also, it is not difficult to add B. longum technically. In this experiment, Queso Blanco cheese containing B. longum was used for the first time to confirm the improvement of intestinal health in companion dogs. Therefore, the purpose of this experiment is to investigate whether the administration of cheese containing B. longum KACC 91563 improves intestinal microbiota and SCFA by controlling the composition and metabolic activity of intestinal microbiota in the healthy companion dogs.
Materials and Methods
Queso Blanco cheese was made according to Ham et al. (2007) with some modification. Four hundreds grams of citric acid in 20 L of water was added to 100 kg of skimmed milk, and salt was not added. B. longum KACC 91563 for QCB cheese was added before molding. Each cheese was stored at 4℃ and fed in a month (QC Moisture 54.7%–55.1%, Protein 36.9%–37.4%; QCB Moisture 53.6%–54.2%, Protein 37.1%–37.5%). The daily intake of B. longum cheese (5.0×108 CFU/10 g cheese) for dogs was maintained at 10 g/kg of body weight on a daily basis for 8 weeks.
Moisture and protein contents were analyzed using a Food Scan (Food ScanTM Lab 78810, Foss Tecator Co. Ltd., Denmark) according to the method suggested by the Association of Official Analytical Chemists (AOAC, 2006).
Fifteen healthy companion dogs (6 females and 9 males) in the Veterinary Medical Center at Chungbuk National University (Cheongju, Korea) were chosen and randomly divided into 3 experimental groups of 5 dogs each. All animal experiments were approved by the Institutional Animal Care and Use Committee of Chungbuk National University (Approval no. CBNUA-1104-17-01). The dogs were 1 to 13 years old, and the body weight range was 2.5 to 35 kg. Breeds included Bulldog, Welsh Corgi, Dachshund, Yorkshire Terrier, Silky Terrier, Poodle, Shih Tzu, Labrador Retriever, Spitz, Cocker Spaniel, and Miniature Pinscher. QCB group was fed with 10 g of Queso Blanco cheese containing B. longum KACC 91563 and QC group was fed with 10 g of Queso Blanco cheese devoid of B. longum KACC 91563 daily for 8 weeks per kg of body weight. A control group (Control) was not fed with any kind of cheese. Fresh fecal samples were collected 5 times, before intake of cheese (week –2 and week 0), during cheese intake (week 4 and week 8), and after cheese intake (week 10), respectively.
Intestinal microbiota analysis was performed by the Mitsuoka method (Mitsuoka et al., 1969). Two different non-selective agar plates, BL for anaerobes and TS for aerobes, and 5 selective agar plate, MacConkey (Escherichia coli and Salmonella spp.), TATAC (Enterococcus spp., and Streptococcus spp.), LBS (Lactobacillus spp.), BS (Bifidobacterium spp.), and NN (Clostridium spp.) were used for detection of intestinal microbiota. BL, LBS, BS, and NN agar plates were anaerobically incubated at 37℃ for 48 h in an anaerobic jar using the steel wool method (Mitsuoka et al., 1969). TS and MacConkey agar plates were incubated aerobically at 37°C for 48 h. After incubation, each plate was examined for bacterial colonies. The colonies were identified based on their morphology, gram staining, spore formation, aerobic growth and 16S rRNA gene sequence. The number of bacteria per gram of stool was calculated and was converted to a logarithmic equivalent (Mitsuoka et al., 1969).
The acetic acid (≥99% purity), propionic acid (≥99% purity), butyric acid (≥99% purity), valeric acid (≥98% purity), isovaleric acid (≥99% purity), 2-methylvaleric acid (internal standard, IS) (≥98% purity) and indole (≥99% purity) were purchased from Tokyo Chemical Industry (Tokyo, Japan).
Analysis of the volatile compounds by HS-SPME method was modified from previously described method (Bianchi et al., 2011). The feces sample (50 mg) was mixed with 280 μL of methanol, 20 μL of IS (5 mg/mL) and 30 μL of 0.9 M H2SO4 solution in 20 mL climp vial (Shimadzu, Tokyo, Japan). The vial was immediately covered with a vial cap. The sample vial was heated on hot plate for 30 min at 60℃. At the same time, a SPME fiber (50/30 μm divinylbenzene/Carboxen/ polydimethylsiloxane (2 cm); No. 57348-U, Supelco Inc., PA, USA) was pre-conditioned using SPME fiber conditioner (Field forensics, Inc., FL, USA) for 15 min at 250℃. After sample conditioning, the fiber was inserted into headspace. The volatile compounds were concentrated on fiber for 15 min at 60℃. The fiber was injected in injector hole. The volatile compounds were detached from the fiber and analyzed by using gas chromatography coupled with quadrupole mass spectrometer (GC-qMS). The GC-qMS was performed with GCMS-QP2010 Ultra system and auto sampler AOC-20i (Shimadzu). The volatile compounds were separated in equipped DB-5 column (0.25 mm×0.25 μm×30 m; Agilent, Palo Alto, CA, USA). The helium was used as carries gas, and column flow rate was 1.3 mL/min. The injection, interface, and ion source temperatures were 250℃, 260℃, and 250℃, respectively. The column program was as follows: initial temperature was 60℃ for 3 min. Next, temperature was increased to a rate of 40℃/min up to 260℃, and maintained for 5 min. In full scan mode, the scanned mass range was 30–250 m/z. Selected ion monitoring was performed by monitoring 43 m/z for acetic acid, 60 m/z for butyric, valeric and isovaleric acid, 74 m/z for propionic and 2-methylvaleric acid, and 117 m/z for indole. Split ratio was 10:1. The mass spectra were analyzed using the Lab solutions GC-MS solution software version 4.11 (Shimadzu). Peak identification was based on comparison with retention time and mass spectra fragmentation of standard. Quantitative analysis of volatile compounds was proportionally calculated with ratio of the standard to IS peak area.
Data was analyzed using SPSS 12.0 for Windows (IBM, USA). Significant differences between the groups were tested by Student’s t test or ANOVA (p<0.05). To demonstrate difference between groups partial least-squares discriminant analysis (PLS-DA) was performed using SIMCA-P version 14.1 (Umetrics, Umea°, Sweden). The significant differences of metabolites (p<0.05) were determined with Student's t-test using Metaboanalyst (www.metaboanalyst.ca). The box plot also was obtained within Metaboanalyst.
Results and Discussion
The composition and metabolic activity of intestinal microbiota have a great influence on the health of the host. Probiotics are widely used because they regulate beneficial bacteria and harmful bacteria in the intestines to maintain healthy intestines (Butel, 2014). Effects of Queso Blanco cheese containing B. longum KACC 91563 on the composition of intestinal microbiota of healthy companion dogs are shown in Table 1, 2, and 3. Before administration of Queso Blanco cheese (–2 and 0 week), Enterococcus (7.8±1.95 to 9.4±1.23) and Lactobacillus (8.9±0.79 to 9.8±0.76) were the major LAB, but Bifidobacterium was not detected in all groups before administration of Queso Blanco cheese, and there was no significant difference in total bacterial counts among three groups (Table 1). At 4 and 8 weeks, Bifidobacterium was not detected in either the control or the QC groups (Table 2). However, Bifidobacterium was only detected on the 8 week after the administration of the cheese containing B. longum in the QCB group (8.4±0.55). And Enterobacteriaceae was significantly decreased to 7.2±0.92 than that of QC and Control group. The increase of Bifidobacterium and decrease of Enterobacteriaceae were considered due to administration of Queso Blanco cheese containing B. longum. The intake of probiotics is known to increase the number of lactobacilli and bifidobacteria in the small intestine (Brigidi et al., 2001). Similarly, the administration of B. animalis B/12 significantly increased the number of LAB in healthy dogs compared to the control group (Strompfova et al., 2014). Matsumoto et al. (2000) and Bartosch et al. (2005) reported that the intake of Bifidobacterium spp. in the elderly increased the frequency of defecation and the number of intestinal Bifidobacterium spp. in the intestines (Bartosch et al., 2005; Matsumoto et al., 2000). Changes in the frequency and number of Bifidobacterium in the intestines have been used as a major index for the improvement of intestinal microbial counts.
Probiotics control host microbiota by limiting and preventing colonization of intestinal pathogenic bacteria. They inhibit pathogenic bacteria by producing bacteriocin SCFA, hydrogen peroxide (H2O2) and diacetyl (George Kerry et al., 2018). The number of Enterobacteriaceae was significantly (p<0.05) decreased in the QCB group (7.2±0.92) compared to QC group (8.4±0.64) and control group (8.7±0.37) at 8 weeks of the cheese administration. In addition, significant (p<0.05) difference was observed in the QC group (8.8±0.30) and control group (9.3±0.37) compared with the QCB group (7.9±1.08) on number of Enterobacteriaceae at 10 weeks (Table 3). Clostridium was not detected in the QCB group during the 8 and 10 week, whereas Clostridium was isolated in the QC group (6.2±0.00 and 4.7±0.00, respectively) and the control group (2.7±0.66 and 3.6±2.30, respectively). Similarly, Baillon et al. (2004) and Biagi et al. (2007) reported that administration of LAB in adult dogs improves the composition of gastrointestinal microbial population and is highly effective in inhibiting the growth of harmful bacteria such as Enterobacteriaceae and Clostridium in the intestine (Baillon et al., 2004; Biagi et al., 2007). The O'Mahony study also showed a significant decrease in Clostridium spp. bacteria at the 6th week of administration of B. animalis AHC7. In fact, the increase in bifidobacteria is known to be accompanied by a decrease in clostridia, and it has also been confirmed in this study (Gibson et al., 1995). The total bacterial count in all groups remained relatively constant throughout the experiment (Fig. 1). No detectable changes were detected in the number and frequencies of Bacteroidaceae throughout the experiment (Table 1, 2, and 3).
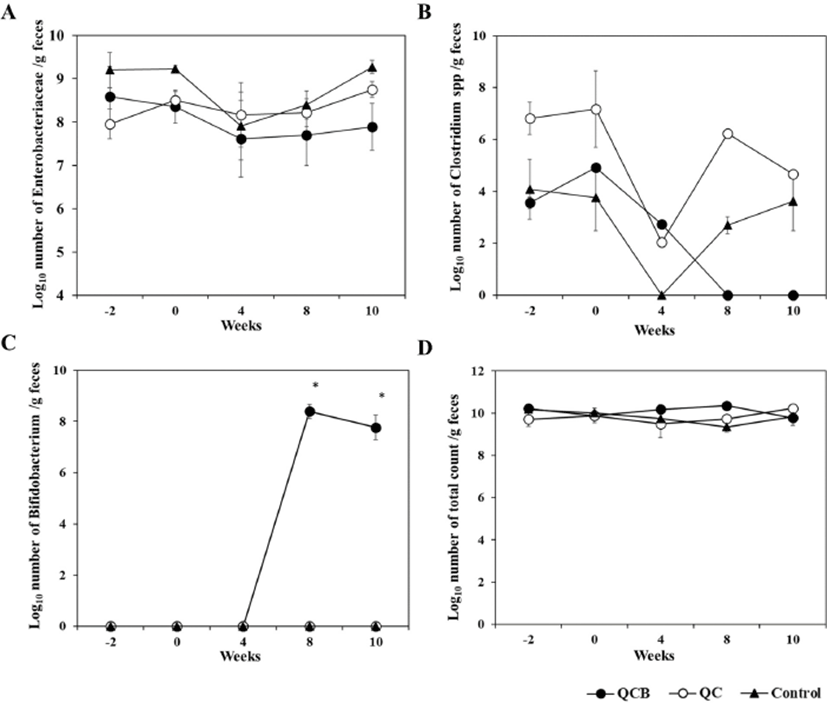
Intestinal enteropathogenic microorganisms such as enterotoxigenic E. coli, Shigella, and Salmonella induce diarrhea. Intestinal microorganisms such as lactobacilli and bifidobacteria, which produce lactic acid, inhibit enteropathogenic microorganisms, thereby preventing and treating intestinal diseases (Hilton et al., 1997; Sullivan and Nord, 2002). Lee et al. (1999) has shown that administration of LGG relieves symptoms of irritable bowel syndrome (IBS) and possess a therapeutic effect against gastrointestinal disorders (Lee et al., 1999). In this experiment it was confirmed that the number of Enterobacteriaceae decreased in feces of dogs fed with cheese containing B. longum. Further studies will be needed to establish the suitability of using B. longum for the prevention of intestinal diseases using intestinal disease models.
The current study demonstrates that administration of B. longum increases beneficial intestinal bacteria and reduces harmful bacteria such as Enterobacteriaceae and Clostridium in healthy companion animals. These changes in the number of intestinal microbiota suggests that B. longum KACC 91563 may control the intestinal microorganisms that affect gut homoeostasis.
The volatile compounds such as SCFAs and indole are end products generated by intestinal microbiota (den Besten et al., 2013). In addition, the volatile compounds are known as essential marker for host health in intestine (Nicholson et al., 2012). Recently, these compounds are analyzed using SPME-GC-MS method because this method allows that rapid, relatively simple and specific quantitative analysis of trace element (Goldmann et al., 2005). Therefore, in this study, SPME-GC-MS method was applied to confirm SCFAs and indole in dog feces. As a result, the six metabolites such as acetic, propionic, butyric, valeric, isovaleric acid and indole were identified in dog feces.
The multivariate analysis, such as principal component analysis (PCA) and PLS-DA are useful tool for characterizing the data in a large number of variables (Cabaton et al., 2013). The PCA as an unsupervised method sets the data by regardless of the origin of variation. However, when more than two groups are included in the analysis, PLS-DA is more appropriate than PCA (Lindon et al., 2004). The PLS-DA as a supervised method is an alternative to PCA because it predicts the data by the significant predictor variables (Kalivodova et al., 2015). In this study, PLS-DA was performed with data of metabolites obtained from week –2 to week 10 among QCB, QC and Control. As a result, the difference between the groups was only shown at 8 weeks. In score plot of PLS-DA model derived from metabolites in dog feces between QCB and Control groups, each point means feces samples fed QCB or Control diets. The two groups were separately clustered (Fig. 2A). Likewise, the biochemical compositions of QCB and QC groups were differently shown each other in score plot (Fig. 2B). In the loading plot between QCB and Control groups, acetic acid and propionic acid mainly affected the separation between two groups (Fig. 2A). Also, the two metabolites were significantly higher in the QCB group than in the Control group (p<0.05) (Fig. 3A). SCFA has an important correlation with total intestinal bacteria, lactobacilli, and bifidobacteria (den Besten et al., 2013). In general, Bifidobacterium spp. produces high levels of SCFA such as propionic acid, butyric and acetic acid and SCFA inhibits pathogens by reducing intestinal pH (Gibson et al., 2004; Roberfroid et al., 2010). The increased levels of acetic acid and propionic acid in QCB group correlated with increased levels of Bifidobacterium spp. compared with control group at 8 weeks. Similarly, Lactobacillus plantarum MA2 was found to raise the concentration of propionic acid in the feces of rat (Wang et al., 2009). Many researcher reported that the administration of probiotic cheese significantly increased the acetic acid concentration compared to the control group and confirmed by the presence of bifidobacteria (Ong et al., 2006; van Zanten et al., 2012). These results demonstrated that the increased level of acetic and propionic acids produced by bifidobacteria. Strompfova et al. (2014) also showed that the administration of B. animalis B/12 in companion dogs resulted in an increase the production of SCFA, such as acetic acid and valeric acid, while fecal coliforms decreased. In this study, Bifidobacterium was increased, while Enterobacteriacea was decreased in the QCB group compared to other groups at 8th week. These results are related to the bactericidal action of acetic acid produced by Bifidobaterium. In the loading plot of the QCB and QC groups, butyric acid was increased in QC group than in the QCB group and had only significant difference (p<0.05) (Fig. 2B and 3B). Clostridium spp. bacteria are known to produce butyric acid in cheese or milk (Brandle et al., 2016). In the QC group, Clostridium spp. was detected at the 8th week in analysis of intestinal microbiota, and this result may be related to a significant increase in the production of butyric acid. The QC group increased acetic acid production more than QCB group, but there was no significant difference.
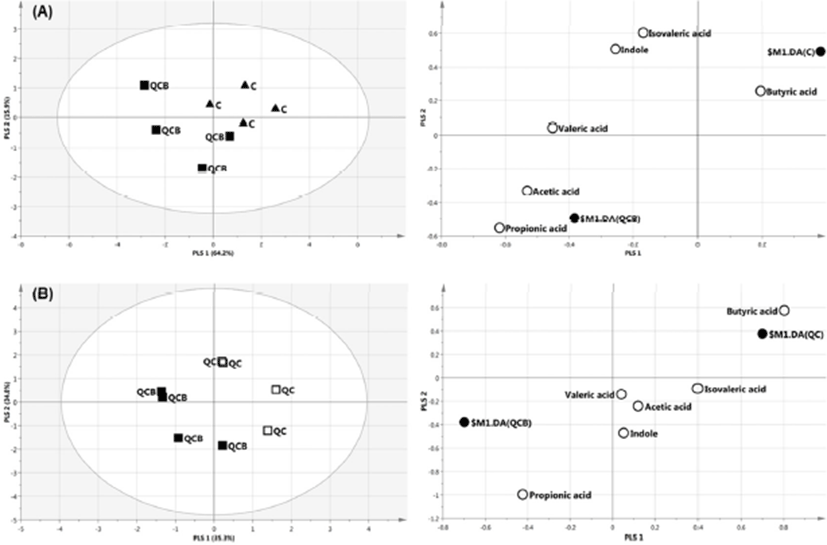
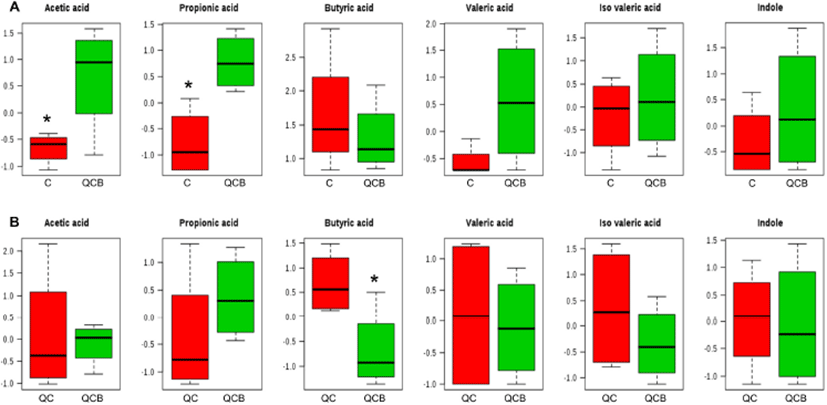
Conclusions
The current study indicates that administration of B. longum KACC 91563 for 8 weeks significantly increased the beneficial intestinal bacteria such as Bifidobacterium and reduced harmful bacteria such as Enterobacteriaceae and Clostridium (p<0.05). SCFA such as acetic and propionic acid were significantly higher in the QCB group than in the Control group (p<0.05). In conclusion, this study demonstrates that administration of Queso Blanco cheese containing B. longum KACC 91563 had positive effects on intestinal microbiota and metabolites in companion dogs. These results suggest that Queso Blanco cheese containing B. longum KACC 91563 could be used as a functional food for companion animals and humans.