Introduction
Clostridium difficile is an anaerobic Gram-positive, endospore-forming bacteria that causes deadly intestinal infections (Liu et al., 2014). C. difficile infection begins with spore germination in the gut of infected persons and subsequent toxin production causing symptoms ranging from mild diarrhea to severe and life-threatening pseudomembranous colitis in infected patients (Surawicz et al., 2013). Clostridium difficile-associated disease (CDAD) is one of the main causes of healthcareassociated infections worldwide. The recent increase in CDAD has placed a rising clinical and economic burden on health systems (Yin et al., 2015). Current treatment for CDAD that typically includes metronidazole, vancomycin, and fidaxomicin, is usually effective; however, disease recurrence occurs in many patients (Cornely et al., 2012; Louie et al., 2011; Mullane et al., 2011). Antibiotics treatment is notorious for high causal rates of CDAD, because C. difficile have antibiotic resistance. To address this issue in the treatment of CDAD, new strategies such as probiotics are being explored.
Probiotics are nonpathogenic microorganisms that can resist digestion within the small intestine of the host and reach the colon alive (Gan et al., 2014); in addition, they confer beneficial health-effects when administered in adequate amount. Dairy-based foods containing intestinal species of lactobacilli, bifidobacteria, and others strains are the major source of probiotics for humans (Kim et al., 2012). Bifidobacteria are indigenous members of the human intestinal microbiota, comprising up to 90% of all bacteria in fecal samples of breast-fed infants (Salazar et al., 2012; Yuan et al., 2008). Bifidobacteria has the potential for use in enhancing digestive health and preventing disease. In particular, bifidobacteria are known to confer enteric protection against pathogenicity by inhibiting the growth of pathogens, including Escherichia coli O157:H7 (Asahara et al., 2004), Helicobacter pylori (Collado et al., 2005), Listeria monocytogenes (Touré et al., 2003), and Clostridium difficile (Kondepudi et al., 2012). In this study, we aimed at evaluating probiotics as a new treatment method for reducing the CDAD symptom caused by Bifidobacterium spp.
Materials and Methods
Clostridium difficile PCR ribotype 027 was obtained from the Department of Pathobiology, University of Guelph (Canada). Prior to the experiments, stock culture was stored at −80°C in brain heart infusion (BHI) broth (Difco Laboratories, USA) containing 17% (v/v) glycerol as a cryoprotectant and supplemented with 0.5% yeast extract and 0.1% ʟ-cysteine (BHIS). The strain was subcultured in BHIS broth and incubated overnight at 37°C under anaerobic conditions. Bifidobacterium longum ATCC 15707 was grown under anaerobic conditions at 37°C in blood-glucose-liver (BL) broth.
C. difficile growth in co-culture with B. longum ATCC 15707 was investigated. For each pair of species, three combinations of the initial cell concentrations were assessed. Viable counts and pH were determined at four timeintervals. All flasks contained 105 cells of C. difficile. Decimal dilution series (105 to 108 CFU/mL) of B. longum ATCC 15707 cultures were adjusted to equal volume. Experiments were conducted in triplicate and repeated three times. C. difficile cultures were grown in MRS broth and B. longum ATCC 15707 were grown in MRS broth supplemented with 0.1% ʟ-cysteine at 37°C under anaerobic conditions. For enumeration of viable cells, samples were inoculated onto the CCFA agar (for C. difficile) and onto the BL-NPNL agar (for B. longum ATCC 15707), respectively, and incubated anaerobically at 37°C for 48 h (George et al., 1979; Teraguchi et al., 1978).
C57BL/6 female mice (5-7 wk old) were purchased from Jackson Laboratory (Bar Harbor, USA). Sixty female mice were housed in groups of 4 per cage under the same conditions, in sterile cages containing bedding, food pellets, and water. For each experiment, mice were randomly assigned to a given treatment group. Each experimental condition was tested in triplicate. Animal experiments were conducted in accordance with the guidelines of the Institutional Animal Care and Use Committee of Chonnam National University (CNU IACUC-YB-2012-45). The mouse model of C. difficile infection (CDI) was based on a previously reported method (Yun et al., 2014) with slight modification. An antibiotic mixture comprising kanamycin (0.4 mg/mL), gentamicin (0.035 mg/mL), colistin (850 U/mL), metronidazole (0.215 mg/mL), and vancomycin (0.045 mg/mL) was prepared in sterile drinking water. All antibiotics were purchased from Sigma-Aldrich. The antibiotic cocktail was administered for 3 d, after which, the animals were provided with regular autoclaved drinking water. A single dose of clindamycin (10 mg/kg) was administered intraperitoneally 1 d before the C. difficile challenge. Thereafter, the animals were infected by oral gavage with 7.0 Log CFU/mL of C. difficile PCR ribotype 027. We examined the effects of orally administered B. longum ATCC 15707 in the mouse model of CDI. The treatment groups received B. longum ATCC 15707 cells (~108 CFU/mL), or heat-killed B. longum ATCC (~108 CFU/mL) by gavage from 1 d to 4 d. The animals were monitored daily for symptoms such as diarrhea, hunched posture, and weight loss (Fig. 1). Body weight and survival data were collected daily on days 0 through 4. On day 2, some of the mice were euthanized, and the colons were removed for measuring morphometric, biochemical, and histological indices of colitis. The disease status of the animals was also assessed by monitoring C. difficile in the mouse feces using a PCR assay targeting the tcdA gene (Yun et al., 2014).
Histopathological analysis was performed to evaluate mucosal damage and inflammation induced by the toxins. Some mice were euthanized on day 2, and the colon was excised to measure morphometric and histological indices of colitis. Cecal and colonic tissues were collected from the mice, fixed in biopsy specimen-embedding cassettes using freshly made ice-cold 4% paraformaldehyde or Carnoy’s fixative, and incubated overnight at 4°C. After the fixation, the samples were washed twice with PBS and dehydrated prior to processing for embedding and sectioning. For histological analysis, deparaffinized 6-μm-thick sections were stained with hematoxylin and eosin (Yun et al., 2014).
The experiments were repeated thrice. The data are expressed as mean±standard error. The differences between means were evaluated by using one-way analysis of variance followed by the Bonferroni procedure for multiple-group comparison. Survival was evaluated using the Kaplan-Meier method. Differences with p<0.05 were considered as statistically significant.
Results and Discussion
As shown in Fig. 2, B. longum ATCC 15707 exerted a growth-inhibitory effect on C. difficile when co-cultured. The growth inhibition of C. difficile was based on the growth curve of C. difficile per inoculum density of B. longum ATCC 15707 (105 to 108 CFU/mL). The higher the inoculum density of B. longum ATCC 15707, growth of C. difficile has been suppressed. The efficacy was observed in all samples at the log phase of B. longum ATCC 15707. In addition, the growth inhibition of C. difficile occurred at pH values of <5.5 (Fig. 3), indicating that the inhibitory effect was possibly due to organic acid-production by bifidobacteria. Bifidobacterium species can produce different relative amounts of acetate, lactate and formate under the same conditions (Bezkorovainy et al., 1989). In this study, the main end product was usually lactic acid, which is in agreement with other reports of growth inhibition due to the ability of lactic acid bacteria to lower pH and produce organic acids (Annuk et al., 2003; Ridwan et al., 2008; Røssland et al., 2003; Tejero-Sariñena et al., 2012; Yun et al., 2014). Tejero-Sariñena et al. (2012) showed that the probiotic strains producing organic acid could inhibit the growth of pathogenic bacteria including S. typhimurium, E. coli, E. faecalis, S. aureus and C. difficile; and Yun et al. (2014) reported that the production of organic acids by Lactobacillus acidophilus could inhibit growth of C. difficile. The results of our study indicated that B. longum ATCC 15707 produced lactic acid that led to the lowering of pH and consequently, the inhibition of C. difficile.
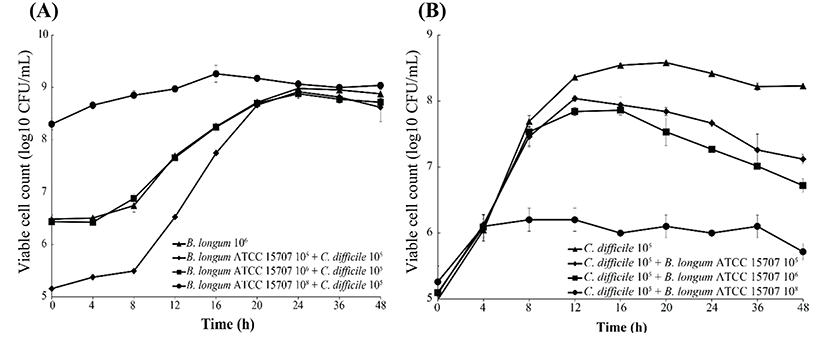
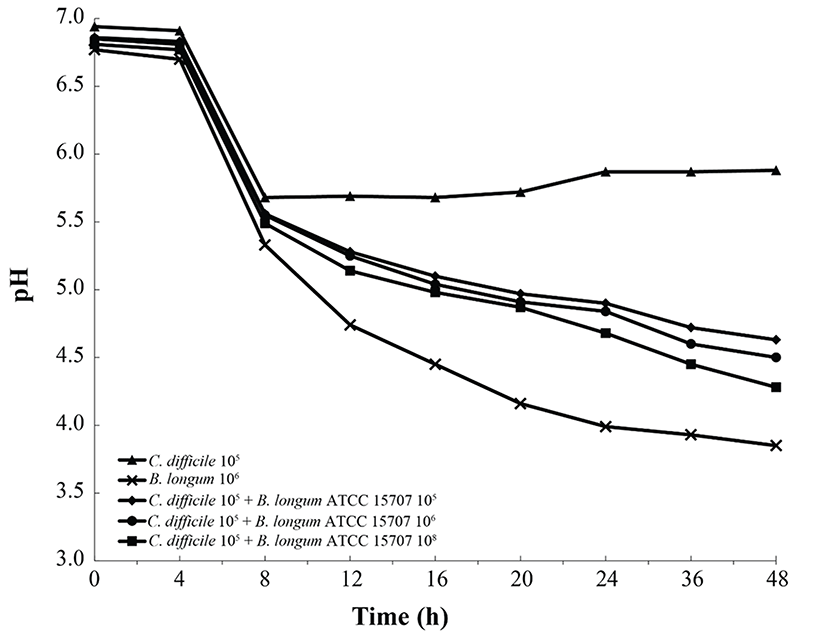
We used a model of CDAD to determine the effects of B. longum ATCC 15707 on infection with C. difficile. This model has been previously used in studies on the effect of pre-and/or probiotics on CDI. Before infecting the mice with C. difficile, an antibiotic cocktail (kanamycin, gentamicin, colistin, metronidazole, and vancomycin) was used to disrupt the intestinal microbiota; and subsequently, B. longum ATCC 15707 was administered by gavage, in order to evaluate the effect of probiotics. All infected mice showed symptoms of CDAD, such as weight loss, hunching posture, and diarrhea. Consistent with some reports, most mice became moribund on days 2 or 3 post-infection. Nonetheless, the B. longum ATCC 15707-treated CDI mice showed a higher survival rate than that of the untreated CDI mice. As shown in Fig. 4, B. longum ATCC 15707 protected mice from death; whereas, untreated CDI mice showed 64% mortality; mice treated with live cells of B. longum ATCC 15707 showed 30% mortality; and mice treated with dead cells of B. longum ATCC 15707 showed 38% mortality. Probiotics have gained interest as a potential therapeutic modality for the prevention and treatment of CDAD. Probiotics, including Saccharomyces boulardii and Lactobacillus rhamnosus GG, have been most frequently evaluated in the prevention and treatment of CDAD in adults and children (Biller et al., 1995; Buts et al., 1993; Guslandi et al., 2000). Studies have shown that probiotic yogurt or mixed probiotics decrease the risk of CDCD (Hickson et al., 2007; Plummer et al., 2010; Szajewska et al., 2001). Moreover, various studies have indicated that probiotics induce improved gut health, via defending the gut against colonization by exogenous microorganisms (Wolvers et al., 2010), producing anti-microbial compounds including short chain fatty acids and bacteriocins that suppress the growth of pathogenic bacteria (De Vuyst et al., 2009), increasing the immunity of the host through their cell wall components (Matsuguchi et al., 2003). Our results suggested that organic acids produced by B. longum ATCC 15707 and cell membrane of B. longum ATCC 15707 could protect the CDAD mice. However, despite the reduced mortality in the B. longum ATCC 15707-treated CDI groups, body-weight profiles of the untreated CDI groups were more favorable, as compared to that of the treated groups because the measurements for that period could only be performed on surviving mice.
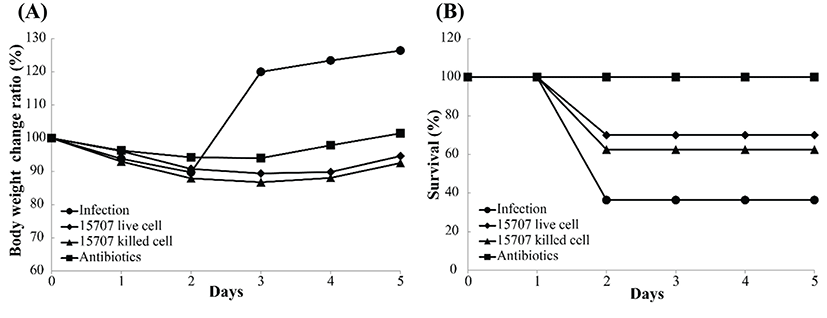
We investigated whether B. longum ATCC 15707 protects gut tissue from damage in the course of infection with C. difficile. In mice infected with C. difficile, colonic histopathology includes submucosal edema, epithelial necrosis, mucosal proliferation, and the presence of inflammatory cells (Chen et al., 2008). Histologic analysis of the colon and cecum of the infected mice revealed that B. longum ATCC 15707 alleviated C. difficile infection-induced damage to the lower intestinal tract tissues. The infection group showed inflammatory cell infiltration, edema, and epithelial cell loss by C. difficile toxins in the colon and cecum (Fig. 5); whereas, the intestinal tissues and epithelial cells in both live and dead cell B. longum ATCC 15707-treated mice maintained structural integrity. This result suggested to have prevented the damage of intestinal tissue by preventing the growth of C. difficile due to organic acids produced by B. longum ATCC 15707. Also, a specific component of consists of the B. longum ATCC 15707 suggests that can prevent intestinal tissue damage caused by C. difficile infections. Similarly, some studies have shown that CDI symptom was alleviated after pre- and/or probiotics treatment (Yun et al., 2014; Yun et al., 2015). Thus, the finding that the epithelial cell loss in the lower intestinal tract was significantly reduced in CDAD mice following B. longum ATCC 15707 treatment, suggested that B. longum ATCC 15707 protects intestinal epithelial cells from apoptosis.
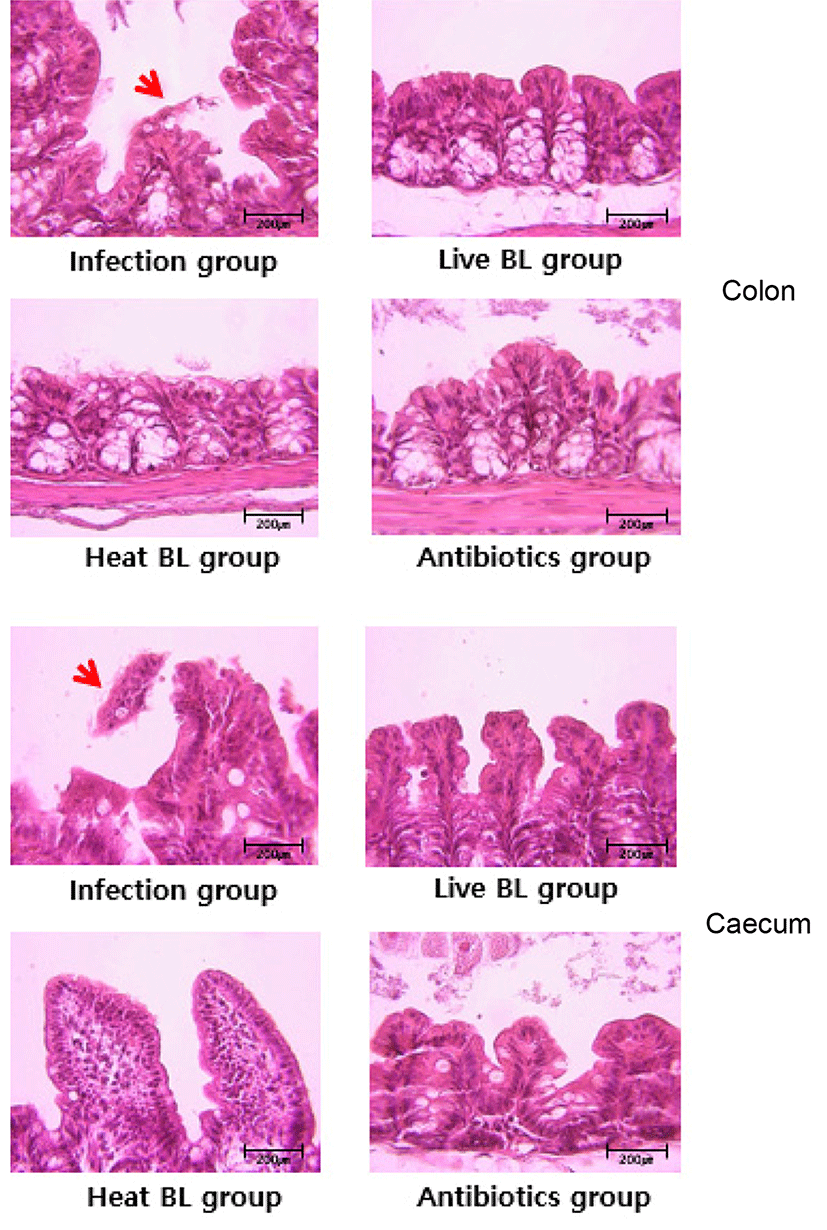
Conclusions
B. longum ATCC 15707 produced organic acid, which led to the lowering of pH and consequently, growth-inhibition of C. difficile when cocultured. B. longum ATCC 15707 also alleviated CDI symptoms in the CDI mouse model, which may be related to the reduced pH caused by organic acid-production by the probiotic bacterium. In addition, the cell wall components of B. longum ATCC 15707 were effective in alleviating CDI symptoms. Thus, this study supported that B. longum ATCC 15707 has potential for use in prevention as well as treatment of CDAD. Thus, B. longum ATCC 15707 may be utilized as an antipathogenic agent for CDAD.