Introduction
Metabolic syndrome (MetS) is a cluster of metabolic abnormalities characterized by abdominal obesity, dyslipidaemia and elevated blood pressure (Eckel et al., 2005; Kaur, 2014). According to National Health and Nutrition Examination Survey (NHANES), the overall prevalence of MetS was around 33% in the past ten years in the United States and it has aroused extensive concerns (Aguilar et al., 2015). Dyslipidemia is the major cause of MetS and often characterized by high low-density lipoprotein (LDL) cholesterol, low high-density lipoprotein (HDL) cholesterol in serum, impaired glucose tolerance (IGT) and imbalance of fatty acids (FAs) metabolism (Anderson et al., 2015). In recent years, increased FAs contribute to higher visceral adiposity and insulin resistance, further leading to liver steatosis (Kotronen et al., 2009). Several studies have reported that life modification particular dietary compounds, may serve as an effective way in MetS prevention by modifying FAs metabolism (Shin et al., 2017; Silva Figueiredo et al., 2018).
In recent years, a variety of research has demonstrated that daily egg consumption can prevent the development of lipid metabolism disorder in MetS (Erami et al., 2016; Missimer et al., 2017). Moreover, lots of research reported there were greater improvements with whole egg consumption compared with yolk-free egg substitute in individuals with MetS, including decreases in plasma triglycerides (TG) and body fat accumulation (Lemos et al., 2018), increases in HDL particles and diameters (DiMarco et al., 2017), as well as less TG accumulation (Yang et al., 2012). These studies suggested that there are some components in egg yolk contributing to improvement of lipid metabolism disorder. HDL from egg yolk (EYHDL), the second largest lipoprotein in egg yolk, possesses important functional activities, such as antioxidant activity (Karlsson et al., 2015), anti-inflammatory (Kassaify et al., 2005) and hypotensive activities (Ansell et al., 2005). It is believed that plasma HDL is responsible for reverse cholesterol transport from extrahepatic tissues to the liver and can mediate FAs metabolism (Karlsson et al., 2015). Despite no definite evidence that EYHDL played the similar important role as serum HDL, some studies have speculated that EYHDL may effectively prevent lipid metabolism disease due to their effect on modifying serum lipoprotein profiles and FAs metabolism. Besides, the fact that daily egg yolk consumption could increase plasma HDL-C level and promote favorable shifts in plasma HDL lipid composition in MetS patients, which revealed the potential regulation of EYHDL (Brossard et al., 2013). However, few nutritional interventions have studied the effects of EYHDL intake on lipid metabolism in individuals with MetS and the related mechanism is not clear. Accordingly, the objective of this study was to investigate the effects of EYHDL diet on lipid profile and FAs composition in serum, liver and feces to evaluate the time dependency of these parameters changes, and potential mechanism in high fat diet (HFD)-induced obese mice.
Materials and Methods
Fresh eggs laid within 24 h were purchased from a local farm (Jiufeng Farm, Wuhan, China). EYHDL was obtained according to the method of Luo et al. (2010) with some modifications: In brief, yolk was diluted with an equal mass of deionized water and mixed with a magnetic stirrer for 1 h. After centrifugating at 10,000×g for 15 min, the precipitate was collected and homogenized with 100 g/L NaCl solution in the ratio of 1:9. This mixture was adjusted at pH 4.0, homogenized with 4% PEG 6,000 w/w and centrifuged at 10,000×g for 15 min. Discarding the supernatant, the sediment was collected for further purification. The extraction ratio of crude EYHDL was 83.27% with purity of 82.16% under optimal extraction condition of pH 4.5 and 4% PEG6000 precipitation. EYHDL purity was calculated by HDL-C commercial test kit (Jiancheng, Nanjing, China). The purification was conducted according to previous studies (Lei and Wu, 2012; Zhang et al., 2011). Crude EYHDL extract was prepared at 5 mg/mL with buffer A (0.05 M carbonate-bicarbonate buffer pH 9.6) at a flow rate of 2 mL/min and filtered through 0.45-μm membrane (Millipore, Billerica, MA, USA) before loading to a Toyopearl DEAE-650M column. The column was first equilibrated with buffer A for 4 column volumes (CVs) and then sample solution was injected into the column. The elution was performed with butter A for 2 CVs and then with butter B (0.5 M NaCl in buffer A) for 2 CVs. The eluate was monitored at 280 nm and collected in fractions, then dialyzed against distilled water.
3-week-old KM male mice weighing 18–22 g were purchased from the College of Veterinary Medicine Huazhong Agricultural University (Wuhan, China) for all pharmacodynamic studies. The animal use protocol has been reviewed and approved by the Animal Ethical and Welfare Committee (AEWC) of Huazhong Agricultural University (No. HZAUMO-2016-045). Mice were housed in cages each containing five animals in a temperature-controlled room (21±2°C), relative humidity (60±5%) and light cycle (12 h light/dark) with free access to food and water. After 7 days of acclimation on laboratory chow, all mice were randomized into three groups (n=20 per group): ND (D12450B, 10% energy derived from fat, 20% from protein and 70% from carbohydrates; 3.85 kcal/g), HFD (D12492, 60% energy derived from fat, 20% from protein and 20% from carbohydrates; 5.24 kcal/g) and HFD+EYHDL (EYHDL, 0.6 mg/g EYHDL in HFD). The ingredient and FAs composition of ND and HFD can be seen in Table 1 and 2. All mice received isometric vehicle (0.6 mg/g mice EYHDL in EYHDL; PBS in ND and HFD), via orogastric gavage through a 50 mm Gauge feeding needle (Feiyang Biotechnology Co. Ltd., Wuhan) for a total of 100 days. The physiological states of mice were monitored every day. Body weight and food intake were recorded once a week. After 60 and 100 days of dietary intervene, 10 mice in each group were euthanized and sacrificed by decapitation following a 12 h fasting period.
Blood samples were harvested from eyepit and centrifuged at 4°C after standing at room temperature for 30 min. Serum aliquots for biochemical and lipidomics analysis were collected into Eppendorf tubes, followed by being frozen by liquid nitrogen and stored at –80°C for further analysis. The concentrations of HDL-C, LDL-C, TC and TG were measured by using commercial kits (BioAssay Systems, Inc. USA). Epididymal adipose tissues were collected and weighed. The livers of all mice in all groups were removed and divided. Liver fragments measuring 1 cm×0.5 cm×0.2 cm were fixed in 4% paraformaldehyde fixative solution for histopathological examination. Another piece of liver (100 mg) was rapidly frozen by liquid nitrogen and stored at –80°C for FAs analysis. The remaining liver fragments were stored in RNA solution (Thermo Scientific, USA) for RNA extraction and gene expression analysis. Fecal samples were collected from each mouse during the last three days before sacrifice. And fresh fecal pellets were collected at the same time of the day (mid-morning). Fecal samples transferred into tubes, then immediately frozen by liquid nitrogen for further analysis. The internal organs, including liver, kidney, spleen, perirenal adipose, perisplenic adipose, and epididymal adipose were removed for weight and all organ indexes calculations using the following formula: Index (%)=organ weight (g)/body weight (g)×100.
Before glucose and insulin tolerance tests, half of the mice in each group were fasted for 8 h. Blood was collected from the tail vein and fasting blood glucose was determinated by using an Accu-Chek blood glucose monitor (Roche). After gavaging with a standardized glucose dose (2 mg/g body weight), blood glucose was measured at the time of 15, 30, 60, and 120 min. For ITT, overnight starved mice were given human insulin (0.75 mU/g) by intrapulmonary injection. The blood glucose concentration was monitored at the time of 15, 30, 60, and 90 min after injection. Both GTT and ITT curves were plotted. The area under the blood glucose curve (AUC) was calculated according to the formula:
where BG is blood glucose.
For histopathological evaluation of the liver and adipose tissue, the paraformaldehyde-fixed liver and adipose tissue samples were dehydrated by graded concentrations of alcohol and embedded in paraffin wax blocks, slicing into 5 μm thickness in a rotary microtome, and finally stained with hematoxylin eosin for morphology. Histopathological images were photographed by bright field microscopy (Nikon Eclipse CI). The total areas of macrovesicular and microvesicular steatosis have been evaluated for the assessment of severity of fatty liver and liver steatosis.
Total lipids from serum (50 μL), liver (50 mg) and faeces (50 mg) were extracted according to the method of Folch et al. with some modifications (Folch et al., 1957). All the oil samples (20 mg) were vortex-mixed and heated at 60°C for 30 min after adding 2 mL of NaOH-MeOH solution (0.1 mol/L). After saponification, the mixture solution was cooled and transferred to a separation funnel. The 2 mL of 14% BF3-MeOH solution was added to the sample and heated at 60°C for 15 min. An n-hexane/1.76% NaCl solution/distilled water (2:1:2, v/v/v) solution was added to the sample solution and the mixture was vigorously shaken. After centrifugation at 5,000×g for 10 min, the upper phase (FAMEs) was collected and the lower phase was extracted again with n-hexane. The extraction solutions were combined and concentrated to dryness under nitrogen, and the residue was dissolved in mobile phase for further analysis.
FAs methyl esters were analyzed by Agilent 7890 GC-MS system. The DB-23 column (60 m×250 μm×0.25 μm) was used in the gas chromatography system. In brief, the temperature program conditions were as follows: the initial temperature of the program was maintained at 130°C for 1 min, followed by increasing it to 170°C at the rate of 6.5°C/min, then to 215°C at the rate of 2.75°C/min, holding for 12 min, finally to 230°C at the rate of 4°C/min, maintaining for 3 min at this temperature. The quantitative analysis and identification of FAs methyl esters in the samples were carried out by comparing the retention time of 37 FAs methyl ester standards (Supelco 37 Component FAME Mix, Supelco, Bellefonte, PA).
The genes involved in lipid metabolism were analyzed by quantitative reverse transcription polymerase chain reaction (qRT-PCR). Total RNA was extracted from liver using animal total RNA isolation kit (Thermo Scientific, USA) according to the manufacturer’s instructions. The reverse transcription reaction was conducted using a revertAid first strand cDNA synthesis kit (Thermo Scientific, USA). The quality and concentrations of RNA samples were checked spectrophotometrically. Using reversed cDNA as a template, RNA expression was quantified using SYBR green polymerase chain reaction reagents. The fold changes of the target gene expression of SREBP-1c, Acaca, Fasn, Scd1, Elov16, Dgat1, Dgat2, GPAT, CD36, Cptla, Fgf21 and MTP were calculated by the 2−ΔΔCt method and GAPDH served as the internal control. The sequences of the genes involved in the present study were obtained from Gene bank (www.ncbi.nlm.nih.gov/Genbank), and the sequences of primers used are listed in Table 3.
All data are expressed and analyzed using the SPSS Statistics 17.0 (IBM, Armonk, NY), as mean±standard error of the mean (SEM). Comparisons between groups were performed with One-way analysis of variance (ANOVA) followed by Dunnett’s analysis methods. s-PLSDA analyzes and heatmap were performed using MetaboAnalyst 4.0 (http://www.metaboanalyst.ca/faces/home.xhtml) (Chong et al., 2018). Differences were considered significant when the p-value was<0.05 and very significantly when the p-value was<0.01.
Results
During the whole experimental period, body weight and food consumption changes of mice were shown in Fig. 1. Body weights of mice in all groups increased constantly during whole dietary intervention period and were significantly higher in HFD after 60 days compared with mice in ND and EYHDL (p<0.01). At the end of 100 days, EYHDL markedly suppressed the body weight gain of mice compared with HFD (p<0.01) (Fig. 1A). Comparing with the food intake of each group (Fig. 1B), there was no noticeable trend observed in food consumption of mice among three groups in the first 28 days. During the following days, the average dietary intake of mice in ND was obviously higher, approximately twice than that in two other groups (p<0.01). The accumulation of abdominal fat tissues, liver and main fat indexes were determined in samples collected on day 60 and 100 (Table 1). In comparison to ND, the liver index significantly increased in HFD while decreased in EYHDL after 100 days (p<0.01). The level of epididymal fat of mice in HFD was notably higher than those of mice in ND (p<0.01). Expectedly, EYHDL restrained the accumulation of epididymal fat significantly (p<0.01) but did not affect perirenal and perisplenic adipose after 60 days (p>0.05).
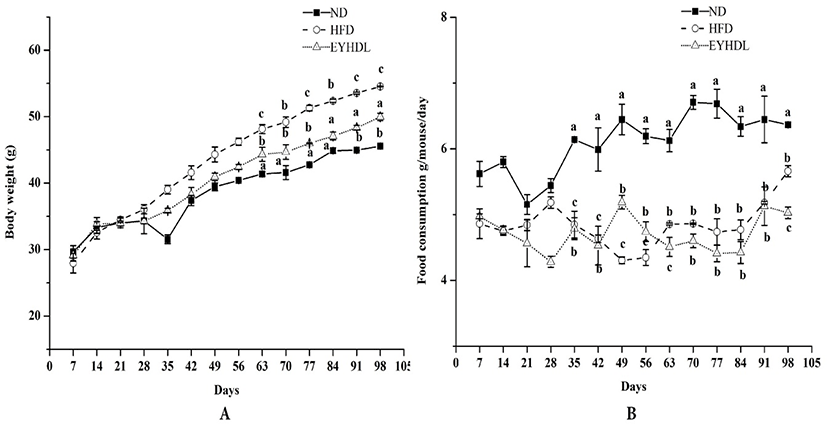
To investigate the effects of EYHDL on the total lipid profile in serum, the concentrations of HDL-C, LDL-C, TC and TG on day 60 and 100 were examined (Table 2). HFD significantly increased the serum concentrations of HDL-C, LDL-C (p<0.01) and TG (p<0.01) compared with ND, while EYHDL significantly reduced the levels of LDL-C (45.11 vs 57.01 mg/dL) and TG (3.01 vs 3.31 mg/dL) compared with HFD. Moreover, mice fed with EYHDL also showed a markedly trend toward increased HDL-C compared with mice in ND and HFD. However, no significant difference in TC content was observed among these groups at the end of 100 days (p>0.05).
At the end of 60 and 90 days, the TG and TC level in the liver of HFD group were significantly higher, 1.77 and 2.30 times higher than in the ND group on 100 day, respectively (p<0.01; Fig. 2A and B). Both the hepatic TG and TC level decreased in EYHDL compared with HFD (p<0.05), showing that EYHDL can decrease hepatic TG and cholesterol accumulation. In order to investigate the effects of EYHDL on the lipid excretions, we collected and analyzed the contents of TC and TG in dry fecal samples. After 100-day treatment, the dried fecal TG excretions in HFD and EYHDL (16.44 vs 15.10 μmol/g) were greater than in ND (7.88 μmol/g, Fig. 2C). The fecal TC levels excreted in ND, HFD and EYHDL were 5.22, 7.06 and 8.39 μmol/g dry feces, respectively (Fig. 2D), indicating EYHDL intake increased TC excretions compared with HFD group.
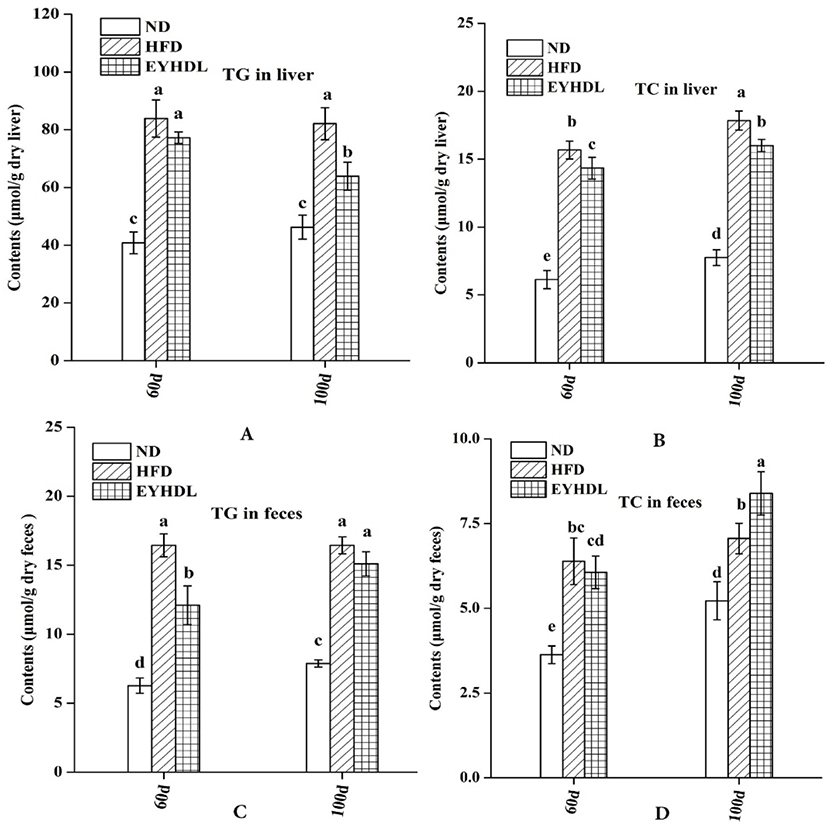
Before observing the histopathology of livers, we compared liver morphology of mice in each group. The mice in ND had normal liver appearance with fresh red color, soft texture and shiny surface, while livers of mice in HFD were obviously different with pale yellow, slightly rough texture and macroscopic fat particles on the surface of liver. Histopathological analysis revealed liver sections from mice in ND had normal morphological appearance, with abundant cytoplasm and no signs of hepatic steatosis and cellular infiltration, that was polygonal, radially arranged, clearly demarcated on day 60 and 100 (Fig. 3). In contrast, hepatocyte of mice in HFD were occupied by loose cytoplasm and many different sizes of microvesicular steatosis on day 60, and the evasion of macrovesicular fatty change was more severe on day 100. Compared with HFD, EYHDL treatment markedly alleviated the proliferation of microvesicular and macrovesicular steatosis on day 60 and 100, although there was also a small number of regions of microvesicular and macrovesicular steatosis on day 100. These results indicated that the inhibiting effects of EYHDL on hepatic steatosis were less effective over time. Fig. 3 also shows adipocyte sizes in epididymal fat tissue in typical images, and average area of adipose cells. The averaged adipocyte sizes were significantly greater in HFD than in ND group (p<0.01), but significant decrease was observed in EYHDL compared with HFD on day 100.
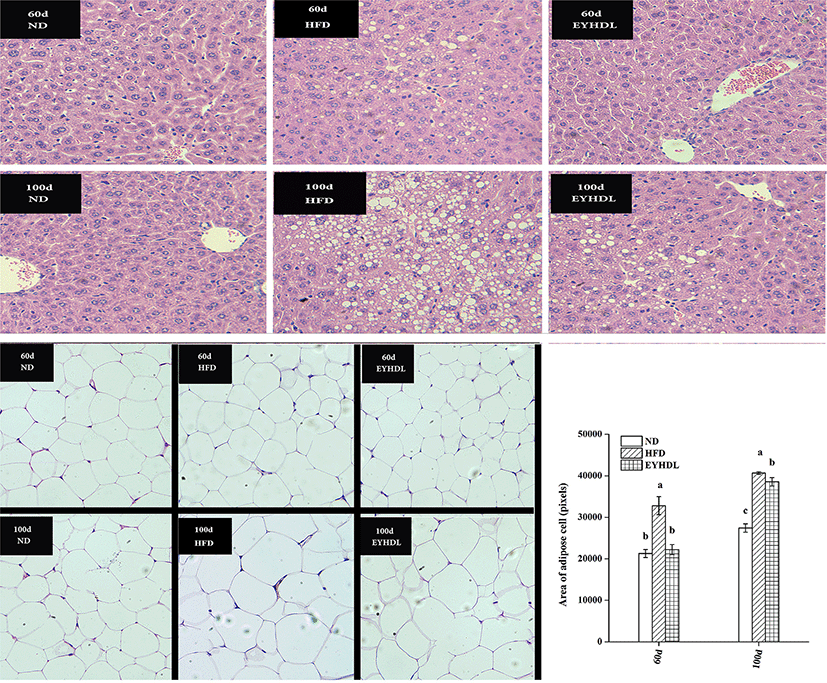
To observe the effects of EYHDL on glucose tolerance, GTT and ITT were carried out on the 60 day and 100 day. Before 2 h of orally administered with glucose, the fasting blood glucose level of mice was significantly higher in HFD than in other groups (p<0.05). Although all groups could rapidly return to baseline after its initial rise at 0.5 h, the blood glucose level in HFD reached around 10 mmol/L, which was significantly higher than ND and EYHDL (p<0.01) (Fig. 4A and C). Insulin tolerance tests (Fig. 4B and D) also revealed significant differences between mice in ND and HFD. These differences indicated HFD preceded the development of IGT, but not high enough to cause diabetes (Martineau et al., 2015). By contrast, the EYHDL mice showed notable improvements in insulin tolerance compared with HFD mice. Furthermore, the total AUC of GTT and ITT were significantly greater in HFD while substantially decreased in EYHDL mice (p<0.01) (Fig. 4E and F). These results suggested that animals fed with HFD appeared IGT and the intake of EYHDL could improve IGT in HFD-fed mice.
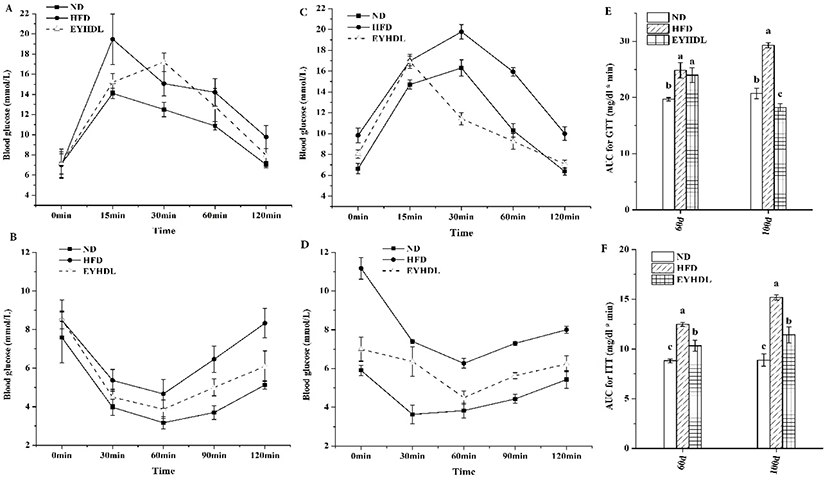
The FAs of liver, serum and fecal samples on day 60 and 100 were analyzed by GC-MS. In our study, a total of 9, 15, and 19 free FAs species were detected in serum, liver and fecal samples, respectively. We conducted sPLS-DA model to analyze FAs profile in serum, liver and fecal samples in three groups. According to the sPLS-DA score plots (Fig. 5), the ND, HFD and EYHDL groups were all clearly discriminated from each other by PC1 and PC2, based on the model applying PC1 and PC2 values of 61.5%–98.5% and 0.3%–8.5%, respectively. ND and HFD mice were clearly separated according to the score plot, indicating that these two groups had distinct FAs profiles. Besides, there was a distinct difference between FAs profile in all samples of day 60 and 100, which proved that the FAs in each group could be affected by the time. In order to assess variation of FAs composition associated with HFD diet, we selected the specific FAs that are most strongly associated with HFD and HFD+EYHDL intake. In the loading plots (Fig. 6), the FAs including C16:1, C18:0 C22:6 and C16:0 in serum (Fig. 6A), C17:1, C18:3 and C16:1 in liver (Fig. 6B), and C20:1 and C21:0 in feces (Fig. 6C) are the most contributors for the discrimination of all groups. All these contributor FAs increased significantly in HFD compared with ND (p<0.01) and a shift in EYHDL was also observed as result of a decrease in the concentrations of, mainly, C16:1 in serum, C18:3 in liver and C21:1 in feces.
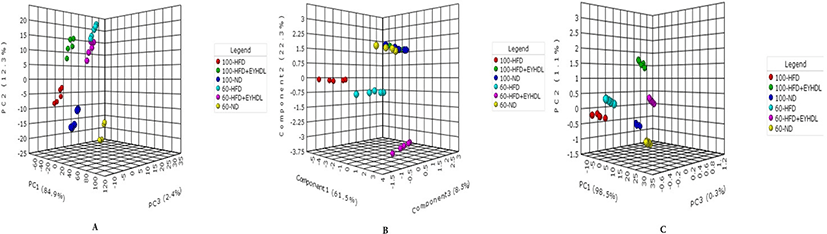
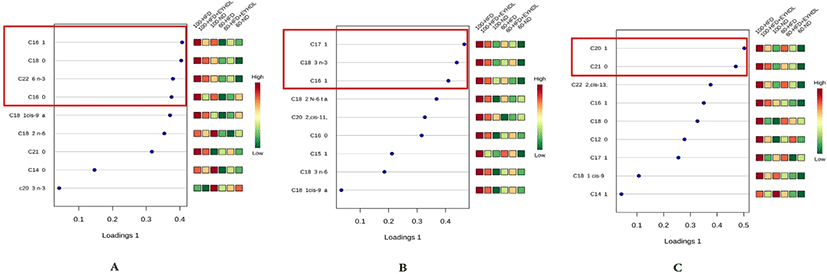
It can be clearly observed that the identified FAs with markedly differentiated abundance in all groups in heatmap (Fig. 7). In serum samples (Fig. 7A), there were higher levels of FAs in serum on 100 d than those on 60 d. The C16:0, C16:1 and C18:1 level in the HFD group were significantly higher than those in the ND and HFD+EYHDL group (p<0.05). In liver samples (Fig. 7B), HFD significantly increased the monounsaturated fatty acids (MUFA) such as C16:1, C17:1 and C20:1 compared with ND and EYHDL (p<0.05). Of note, the contents of polyunsaturated fatty acids (PUFA) including C18:3 and C22:6 diminished significantly in HFD+EYHD (p<0.01). In the meantime, the C16:1 decreased markedly while the level of C18:0 did not change in EYHDL (p<0.05). In addition, C20:1, a downstream product of SFA desaturation and elongation, also significantly decreased in liver with EYHDL treatment. More kinds species of FAs (19) were detected in fecal samples, the concentrations of C18:1, C16:1 and C16:0, the three most abundant FAs in fecal samples, significantly increased in HFD (p<0.05) while decreased in EYHDL compared with HFD group (Fig. 7C).
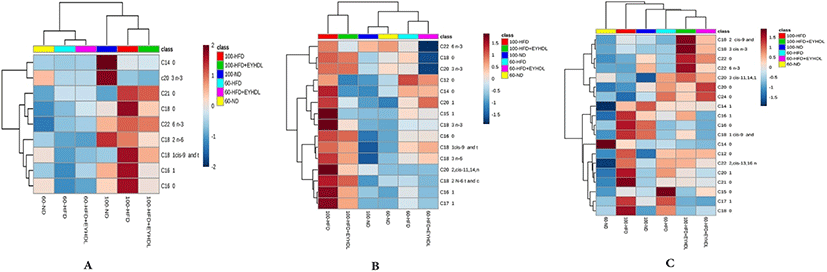
We further compared the changes of different FAs species in Table 3. As expected, the total FAs in serum, liver and fecal samples in EYHDL markedly decreased by 10.5%, 9.8%, and 27.4%, respectively, compared with those in the HFD group (p<0.05). The levels of total SFA and MUFA in the liver and feces were decreased noticeably, while level of total PUFA especially n-6 PUFA in feces increased in EYHDL (p<0.01). The levels of PUFA species in serum (54.89 vs 58.54) and in liver (35.85 vs 39.82) were decreased while increased significantly and feces (3.44 vs 1.70) in EYHDL compared with HFD (p<0.01). The ratio of MUFA/SFA reduced in liver while drastically increased in feces in EYHDL (p<0.01) compared with HFD group. The lower n-6/n-3 PUFA ratio in serum and liver was also observed in EYHDL (p<0.05), while the n-6/n-3 PUFA ratio in feces had no significant difference among all groups (p>0.05). EYHDL induced a significant increase in the ratio of PUFA/SFA in feces on day 60 and 100 in comparison with HFD (p<0.01).
To investigate the potential molecular mechanism of hepatic lipid accumulation, the expressions of hepatic genes related to FAs metabolism were investigated (Fig. 8). Compared with HFD, SREBP-1c downregulated 3.87 and 9.43-fold in EYHDL on day 60 and 100, respectively (p<0.01). The gene expression of Elovl-6 also decreased 3.67-fold in EYHDL than in HFD. The expression of Acaca, Fasn and GPAT decreased significantly, while Scd1 increased 3.28-fold in EYHDL than in HFD, leading to less contents of SFA in EYHDL and further neutralizing the upregulation effect of the HFD (p<0.05). Notably, the hepatic Cptla mRNA level was induced by HFD to 6.24-fold of ND on day, and EYHDL alleviated the significant upregulation. At the same time, the hepatic mRNA expression of Dgat1 and Dgat2, also known as specific enzymes that catalyze the final step of TG synthesis, decreased 2.01-fold and 1.78-fold in EYHDL, and the expression of CD36 was markedly decreased in EYHDL than in HFD (2.96 vs 6.30 fold than ND group) on 100 day.
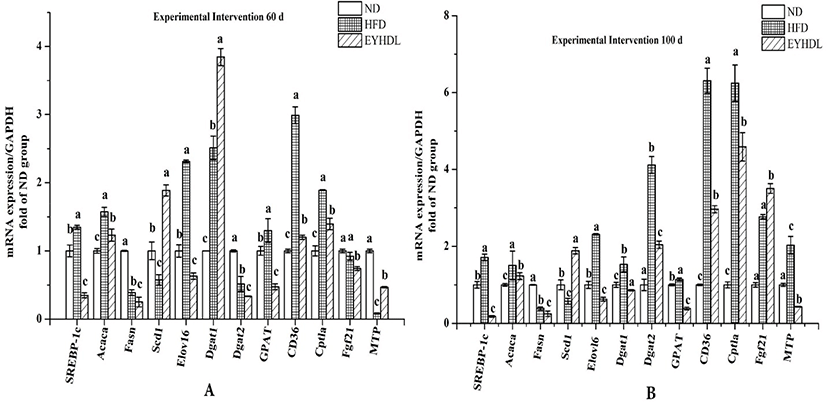
Discussion
Long-term dyslipidemia is the main cause for type 2 diabetes, nonalcoholic fatty liver disease (NAFLD) and other cardiovascular diseases, which have drawn widespread attention (Huang et al., 2017). Targeting the excessive lipid accumulation and metabolism in dyslipidemia may lead to successful therapies that reduce the prevalence of MetS (Farrell et al., 2015). It has been demonstrated that daily egg feeding can potentially improve reverse cholesterol transport, type 2 diabetes risk and CVD (Blesso, 2015; Richard et al., 2017). EYHDL, a potential component regulating the dyslipidemia, seems to play a crucial role in the treatment of obesity and MetS. From now, the potential mechanism is not clear and few studies focusing on the effect of EYHDL. In this study, we used HFD to induce profound symptoms of dyslipidemia in KM male mice, including increased body mass and mesenteric fat accumulation, impaired the blood tolerance. We assessed the effects of EYHDL on alleviating dyslipidemia caused by HFD, as well as genes expression involved in FAs metabolism. These changes suggested that EYHDL may have potential in ameliorating the dyslipidemia in HFD-induced obese mice.
It is reported that favorable lipoprotein profile including high HDL-C, low LDL-C and TG can reduce the risk of atherogenic dyslipidemia (Kontush, 2015; Orsoni et al., 2016). Numerous studies demonstrated that daily egg consumption has been associated with improvements in high lipoprotein particles characteristics (Shin et al., 2017; Yang et al., 2012). In this study, HFD significantly increased the body weight and decreased food intake compared with ND, we speculated the significant lower food consumption in HFD than in ND was attributed to the distinct amount of calorie contained in food (Dossi et al., 2014). Notably, EYHDL significantly lowered the feed intake, leading to lower weight gain. Possibly, EYHDL affected feed intake by modulating appetite and feed conversion efficiency (Neave et al., 2018; Omasaki et al., 2017). We have proposed that lowered feed intake combined with lower level of liver index and epididymal fat may be major contributors to the lowering of body weight. The intake of exogenous HDL (EYHDL) could markedly decrease the level of serum LDL-C and TG, which will inhibit the TG accumulation in liver (Kapourchali et al., 2016). Although higher HDL-C in serum was observed in EYHDL as we expected, but the effect in HDL-C in serum was not marked. Despite the related mechanism was not clear, previous research reported that phospholipid feeding has been associated with increases in HDL-C in animal and human studies (Blesso, 2015). We assumed that high phospholipid contained in EYHDL may be partly responsible for the observed improvements in HDL-C (Blesso et al., 2013). The hepatic TG, cholesterol accumulation and the proliferation of steatosis were also significantly inhibited by EYHDL. In addition, the intake of EYHDL also moderately alleviated IGT of mice in HFD. The inhibiting effect of EYHDL on hepatic steatosis were observed but the effect was less effective over time. We speculated that the effect of HFD might be associated with the conditions of growth pattern, homeostasis, and biological adaptation of the mice, which was also observed in previous studies (Eftekhar et al., 2015; Huang et al., 2017).
We also measured the adipocyte size in each group, which has been shown to be an indirect sign of inflammation and also providing a potential link between obesity, insulin resistance and metabolic dysfunction (Spencer et al., 2014). In the current study, the averaged adipocyte sizes were significantly greater in HFD than in ND group (p<0.001), which indicated increased triglyceride storage caused by HFD could lead to hypertrophy of adipocytes (Poret et al., 2018). The significant decrease in the average area of adipocyte sizes was observed in EYHDL showed that the smaller adipocyte sizes were closely related to the less body mass and mesenteric fat accumulation.
Disorders of lipid especially FAs metabolism can further develop to dyslipidemia and the changes in FAs classes are important signals for the conformation of steatotic liver and obesity (Eisinger et al., 2014). To further investigate the effect of EYHDL on lipid metabolism, especially FAs metabolism, a lipidomic approach has been used to further define altered FAs species in the liver, serum and fecal samples. It is believed that high levels of FAs can result in increased intracellular TG synthesis and formation of lipid droplets in serum and liver (Listenberger et al., 2003). Here, we reported that 100 days of EYHDL feeding in obesity-prone KM mice led to lower serum levels of FAs such as C18:0 and C16:0 compared to HFD diet-fed mice. It has been confirmed that the serum FAs are derived from hydrolyzed TG and lipoproteins and lipolysis in adipose tissue (Angrish et al., 2013). This indicated EYHDL could alleviate the accumulation of TG in serum and adipose tissue, which is consistent with the results of histology of adipose tissue.
Our study has shown a decrease in hepatic saturated acid proportion (C14:0 and C12:0), MUFA (C16:1, C16:0 and C18:1) and PUFA (C18:3 and C18:2) in EYHDL. Because the hepatotoxic effect of saturated acids is usually happened in fatty livers, a significant decrease in C14:0 and C12:0 proportions in EYHDL may potentially alleviate liver injury caused by HFD (Stanković et al., 2014). We also found increased level of C16:1 and C18:0 in both serum and liver. High levels of C16:1 and C18:0 could be explained by the mechanism: a major part of the palmitic acid (C16:0) generated by fatty acid synthase is converted into stearic acid (C18:0) via carbon chain elongation Elovl6 (Dória et al., 2014). Scd1, catalyzing SFAs to △-9 MUFAs, is converted C16:0 to C16:1 or converting C18:0 to C18:1 (Chen et al., 2016). The higher ratio of C16:1 and C18:0 in HFD is ascribed to the increased expression of Elovl6 and decreased expression of Scd1. These results are in agreement with the reports that C16:1 and C18:0 increased in mice with idiopathic fatty liver and humans with NAFLD or diabetes (Zhang et al., 2017). Whereas the treatment of EYHDL alleviated the increase of C16:1 and C18:0 and achieved similar results to those in ND, which was also associated with the improvement in expression of Elovl6 and Scd1. Elovl-6, a target of SREBP-1, is a transcription factor governing FAs synthesis (Kumadaki et al., 2008). Being consistent with previous research, we claimed that C16:1 and C18:0 can be used as indicators for predicting advanced fatty livers (Murase et al., 2011). The n-6/n-3 PUFA ratio was found significantly increased in the human steatotic liver mostly due to the decreased level of n-3 PUFA (Jelińska et al., 2017). On the contrary, the lower n-6/n-3 PUFA ratio in serum and liver in EYHDL could have beneficial influence on the lipid clearance, which was also observed in healthy subjects (Jump, 2011). The lower n-6/n-3 PUFA ratio in EYHDL might be explained by the downregulation of hepatic Scd1, Elovl 6 mRNA compared with HFD. Apart from assessing the expression of Elovl6 and Scd1, we also measured the expression of genes involved in fatty acid synthesis. Acaca and Fasn are key rate-limiting enzymes in de novo fatty acid synthesis and its expression can be supressed in EYHDL (Kim et al., 2002). This enhanced expression was relevant to the less hepatic lipid accumulation and decreased serum levels of LDL-C and TG. The present results suggested that expressions of Acaca and Fasn were suppressed markedly in EYHDL. In addition, SREBP-1c is an essential nuclear transcription factor that can regulate the expression of lipid metabolism genes in liver and it is also involved in FAs and TG synthesis (Li et al., 2015). EYHDL led to lower hepatic SREBP-1c, Cpt1a and Fasn mRNA expressions, associated with obesity and fatty livers, which might explain the inhibition of microvesicular steatosis and macrovesicular steatosis. Collectively, our data showed that EYHDL could inhibit de novo synthesis of fatty acid through suppressing genes (Acaca and Fasn) associated with de novo fatty acid synthesis and alleviate the accumulation of lipid in mice maintained on HFD (p<0.01).
In fecal samples, the levels of total FAs were significantly higher in HFD and EYHDL, showing that higher fat intake will accelerate more FAs excretion through lipid metabolism. At the same time, EYHDL did not increase the total amount of FAs in fecal samples, which showed the amount of FAs excretion couldn’t be promoted by EYHDL. Although no higher FAs excretion was observed in EYHDL, there was higher level of PUFA such as C18:2 and C20:3 excretion. Although the biological significance was not clear, this may indicate that EYHDL could promote more PUFA excretion in HFD diet after the gastrointestinal tract, which will further change the production and metabolism of FAs by intestinal bacteria. It was reported that PUFA especially n-3 PUFAs could reduce the adherence of most probiotic lactobacilli (Broin et al., 2015). The higher level of PUFA in HFD showed that high fat intake will exert a negative effect on the metabolism and growth of lactobacilli, while EYHDL seems not to aggravate the negative impact and the mechanism needed to be further investigated.
In conclusion, this study confirmed EYHDL could modulate de novo FAs synthesis and attenuate the metabolic abnormality and fatty liver disease caused by HFD. These favorable effects were confirmed to be attributed to the decreased TG in serum and hepatic lipid accumulation as well as the improved blood tolerance. The results on FAs analysis and expression of related genes provided more detailed information on the regulation of EYHDL. However, the mechanisms on regulating lipid metabolism by EYHDL need to be further investigated. More and more diet intervention strategies to prevent and treat obesity have arisen widespread attention. There is a big dispute about the nutritional evaluation of egg especially egg yolk. These results have advanced the studies of the therapeutic potential of EYHDL and may promote the recommendations for egg yolk intake or purified EYHDL in the future.