Introduction
Myofibrillar proteins (MPs) is an important source for food proteins, composed of 55%–60% of total proteins in meat. Appropriate processing of MPs can be to form a stable network structure, gel property, sensory characteristic, and consequent better meat quality. Protein oxidation, however, unavoidably occurs to some extent during curing processes or storage, which results in a poor organoleptic and processing quality, and even raises health concerns such as cardiovascular disease and colon cancer with the excessive consumption of oxidative proteins (Kim et al., 2013). Oxidation can cause the modification of side-chain groups of proteins, large molecular protein crosslinking and conjugation, as well as significant negative changes in protein functions including gel property, water holding capacity (WHC), emulsifying, color, and texture of meat products (Estevez and Luna, 2017). Thus, it is urgent to improve the property of MPs in antioxidation and gel forming in the meat industry. With the increasing trend of health awareness, production of rabbit protein ingredients is growing throughout the world due to the low levels of fat, cholesterol, and sodium, but high levels of protein, all of which certainly well meet the health requirements of modern consumers (Cullere and Dalle Zotte, 2018; Dalle Zotte and Szendro, 2011; Escriba-Perez et al., 2017).
Tetramethylpyrazine (TMP) is a bioactive ingredient found in cereals and cereal products, enriched in genus Ligusticum, natto, and fermented cocoa beans (Hashim et al., 1998; Pubchem, 2019). Recent studies have shown that TMP exhibits potent nootropic, antioxidative, antiinflammatory, and antimicrobial activities in the models of rats and chickens (Kao et al., 2013; Liu et al., 2018; Wu et al., 2013). The antioxidative mechanism of TMP, as well as pyrazine compounds, may be related to its redox regulation by cleaning reactive oxygen species (Zou et al., 2018). In food additive classes, TMP is a flavoring agent listed in EU Food Improvement Agents, and pyrazines are important compounds of Maillard reaction flavors (Scalone et al., 2019). Pyrazine compounds, including 2,5-dimethylpyrazine, 2-ethyl-3-methylpyrazine, and 2,3,5-trimethylpyrazine, can interact with porcine MPs at different pH values (Shen et al., 2019). Given its the antioxidative activity mentioned above, the effect of TMP on the antioxidation of meat protein is unclear. So, hypothetically, TMP can depress MPs oxidation during processing and consequently improve meat quality. The aim of this study is to investigate the TMP effect on the physicochemical and gelation property of rabbit MPs with or without oxidative stress.
Materials and Methods
TMP (2,3,5,6-TMP, purity: ≥99%) was purchased from Deruifeng commercial company (Jinan, China). Treatments included control, control+TMP (15 mg/L), oxidation and oxidation+TMP (15 mg/L). Analyzed levels of TMP in control+TMP and oxidation+TMP were 13.6 and 14.1 mg/L, respectively. The TMP concentration in the present study referred to the authors’ pilot study and the bioactive effect of TMP as a dietary supplement on animals (Liu et al., 2018).
The oxidation treatment was prepared using hydroxyl free radical oxidation system (Xiong et al., 2010). Briefly, MPs was diluted into 50 mg/mL using 20 mM phosphate buffered solution (pH 7.0), and dissolved. Then, FeCl3, ascorbic acid, and H2O2 were added into the protein solution. The oxidation system was composed of 40 mg/mL rabbit MPs, 0.01 mM FeCl3, 0.1 mM ascorbic acid, and 10 mM H2O2. The control treatment excluded the FeCl3, ascorbic acid, and H2O2.
Fresh rabbit longissimus without anadesma and fat was collected, chopped, and ground for the extraction of MPs according to the method by Park et al. (2007) and Feng et al. (2017) with minor modification. Briefly, 50 g meat sample was mixed with 200 mL buffer solution (0.1 M KCl, 2 mM MgCl2, 1 mM EDTA, 10 mM Na2HPO4), homogenized and centrifuged at 2,000×g for 15 min at 4°C. Supernatant was discarded and pellets were kept and treated repeatedly three times as mentioned above. The pellets were then washed three times with 4 volumes of 0.1 M NaCl using the same mixing and centrifugation conditions listed above except for the last suspension that was filtered through four layers of gauze to eliminate the connective tissue. The filtrate was adjusted to pH 6.2 using 0.1 M HCl, centrifuged at 2,000×g for 15 min at 4°C. The final MPs were kept in tightly capped bottles and stored in ice slurry before use (within 3 h). The protein concentrations of the myofibril pellets were measured as described by Liu et al. (2010).
The content of free thiol group was quantified using the method by Xia et al. (2009). The sample was diluted to 1 mg/mL using Tris-glycine buffer (0.086 M Tris, 0.09 M glycine, 4 mM EDTA, pH 8.0). Then, 15 μL Ellman’s reagent (5,5′-dithio-bis-[2-nitrobenzoic acid]) was added into 1.5 mL sample, agitated at room temperature for 1 h, and centrifuged at 12,000×g for 10 min. The absorbance of supernatant was detected at 412 nm wavelength (Spectronic 200, ThermoFisher Scientific, Carlsbad, CA, USA). Dityrosine content was expressed as fluorescence intensity (FI) with the detection conditions of excitation 325 nm, emission 420 nm, and slit width 10 nm by a wavelength scanning parameter (Fluoroskan™) according to the method by Davies et al. (1987).
In terms of the FI of endogenous tryptophan, MPs at 0.1 mg/mL were diluted by 15 mM piperazine-1,4-bisethanesulfonic acid buffer containing 0.6 M NaCl (pH 6.25) at room temperature (18°C). Before measurement, sample temperature was equilibrated for 30 min at room temperature to ensure recording fluorescence at the same temperature. The detection conditions included excitation at 283 nm, emission wavelength at 331 (300–400) nm, slit width at 5 nm, and electric tension at 700 V using a fluorescence spectrometer using Fluoroskan™. The cuvettes had to be removed from the excitation light between the single measurements to avoid photooxidation of the tryptophane residues; fluorescence was measured every 10 min.
Surface hydrophobicity of MPs was detected as described by Chelh et al. (2006). MPs was diluted to 5 mg/mL using 20 mM phosphate buffered solution. A total 0.2 mL bromophenol blue (BPB) solution (1 mg/mL) was added into 1 mL MPs, mixed, centrifuged at 2,000×g for 10 min at room temperature, and supernatant was collected. The surface hydrophobicity was expressed as the absorbance of the binding of BPB to protein surface hydrophobic regions in the supernatant monitored at 595 nm. The contents of TMP in the samples were determined according to the Chinese Standard of Light Industrial Manufacturing (QB/T 2748-2012).
The heat-induced gel was prepared by using MPs (40 mg/mL) bathed in water at 80°C for 30 min. The gel was weighed, bathed in water at 80°C for 30 min, cooled to room temperature, dried with filter paper, and weighed again, and cooking yield was expressed as the weight ratio of after- to pre-bathed. The inspection sample of rabbit MPs gel was put into flat and smooth slices at 5 mm in thickness. The color of MPs sample was evaluated immediately using CIE Lab system (L*, lightness; a*, redness; and b*, yellowness) with a color-difference meter instrument (NH310, Shenzhen 3nh tech. Co. Ltd., China) at illuminating geometry 8/d, light source D65, and auto white and black calibration (≤0.40L*a*b*) at startup. Three pieces were tested to minimize possible errors. Whiteness, an index for the general appearance of MPs gel, was calculated as: Whiteness=100−[(100−L*)2+a*2+b*2]1/2.
In terms of the detection of WHC, Eppendorf tubes without/with MPs gel samples (approximately 5 g) were accurately weighed before and after centrifugation at 6,000×g for 15 min at 4°C. WHC (%) of MPs gel was calculated using the formula: WHC=(M2−M)/(M1−M)×100, where, M as the weight of empty tube (g), M1 as the weight of tube containing sample before centrifugation (g), and M2 as the weight of tube containing sample after centrifugation (g).
Gel water distribution was detected using Low Field Nuclear Magnetic Resonance according to the method by Han et al. (2014) with detection conditions of 22.6 MHz proton resonance frequency and 32°C measuring temperature.
All treatments repeated 5 times starting from extraction of MPs. Each sample was detected in triplicate, and the mean was an average value. Data were analyzed using General Linear Model procedure of SAS (SAS Inst. Inc., Cary, NC, USA). The model for the factorial design included the TMP, oxidation, and the TMP×oxidation interaction and differences of variables were separated using Tukey’s Studentized Range test. The differences between treatments control+TMP vs. control, oxidation vs. control, and oxidation+TMP vs. oxidation were analyzed using independent sample t-test. Data in tables were expressed as mean and the average SEM, and FI of endogenous tryptophan were exhibited as means and standard errors. A lowercase superscript for means in tables denotes a significant level at p<0.05, and uppercase superscript at p<0.01.
Results and Discussion
As shown in Table 1, compared to the control group, MPs treated with TMP increased the content of free thiol group, and decreased the fluorescent intensity of dityrosine (p<0.05); whereas oxidation treatment decreased free thiol group, but increased hydrophobicity and dityrosine content (p<0.01). TMP treatment to oxidative MPs increased free thiol content, but decreased hydrophobicity and dityrosine (p<0.01). The effect of TMP on the MPs property was more pronounced with the exposure to oxidative stress, which caused interactions (p<0.01). These findings indicate that TMP can improve the physicochemical property of MPs under oxidative stress conditions.
The free thiol group is commonly used as an indicator for protein oxidation in biological samples. With the oxidative stress, protein free thiol groups can be oxidized into disulfide bonds, so the free thiol groups as well as total thiol groups are decreased (Feng et al., 2017; Trivedi et al., 2009). In the present study, the higher levels of free thiol group in TMP treatments indicates it can increase the oxidative stability of proteins in the meat processing. The antioxidative property of TMP or its analogues was initially reported in animals or humans. As a feed additive, TMP reduced gut or liver oxidative stress of chickens by positively interfering with opportunistic bacteria and endotoxins or SOD, T-AOC, and GSH-Px (Liu et al., 2018; Mehmood et al., 2018; Wang et al., 2016). TMP ameliorated hepatocellular mitochondrial dysfunction by decreasing inflammatory responses but increasing AQP8 protein expression in septic rats (Tao et al., 2017). The information, however, about TMP on protein thiol oxidation is unavailable. Additionally, the thiol groups can be decreased with partial thiol groups covered by aggregated proteins due to oxidative degeneration (Lund et al., 2011).
Dityrosine is a fluorescent molecule formed as a result of normal posttranslational processing. In many structural proteins, dityrosine confers resistance to proteolysis and physicochemical trauma as a stabilizing crosslink (DiMarco and Giulivi, 2007). Dityrosine has also been found in oxidative/nitrative stress under a variety of conditions and biological systems because tyrosine is sensitive to hydroxyl radical and is easily oxidized into its dimer form (Martinez and Kannan, 2018). Furthermore, oxidized tyrosine and dityrosine exposure changed systemic metabolic diseases and object recognition deficits in rodents (Ran et al., 2016; Yang et al., 2017b). In the present study, oxidative stress induced dityrosine oxidation, but TMP exhibited a protective function with/without oxidative stress.
In the present study, the finding that TMP decreased surface hydrophobicity further evidenced its potent antioxidative function on rabbit MPs. As known, protein oxidation can increase its surface hydrophobicity due to more hydrophobic amino acids exposed to the surface, leading to protein conformational change or aggregation and crosslinking reaction between proteins (Davies et al., 1987; Kramer et al., 2017). Importantly, in the present study, the effect of TMP was more pronounced on the MPs with exposure to oxidative stress, which caused significant interactions between the TMP and oxidative stress. This is consistent with the finding that electrostatic and hydrophobic interactions were found between porcine MPs and pyrazine compounds in pH varied models (Shen et al., 2019). Overall, these findings imply that it is absolutely essential to reduce the generation of oxidation protein products in food system through improving modern food processing methods.
Compared to control, TMP increased whiteness of rabbit MPs (p<0.05, Table 2), while oxidation decreased gel yield, whiteness, and WHC (p<0.01). Compared to oxidation group, TMP increased the three parameters (p<0.01). Interactions were found on gel yield and WHC (p<0.05). Oxidation caused the damages of side-chain amino acids structures and proteins conformation, the loss of some water or juices, and consequently the reduction of WHC (Kramer et al., 2017). Furthermore, disulfide bond crosslinking caused by oxidation reduces the interactions between functional groups of proteins, leading to poor water retention and texture of protein gels (Feng et al., 2017).
In the present study, TMP increased gel yield and water retention of MPs possibly in that the antioxidant binds free radical groups, retards oxidation process, protects protein side-chains and conformation, but more research about this is needed. Additionally, TMP increased whiteness of rabbit MPs gels, which may be due to the light scattering effect of proteins with less oxidation in TMP treatments than do in others. The findings in the present study indicate that TMP might interact with myofibrils and consequently influence its physicochemical properties. Further application of TMP should be in various meat and its products with more focuses on color parameters of L*, a*, and b* other than whiteness. Interestingly, pyrazine compounds affected the porcine MPs flavor releasing, and the release behavior may be due to protein-protein interactions and surface tension (Shen et al., 2019). So, TMP may affect rabbit MPs flavor releasing, which deserves further study.
The FI of endogenous tryptophan was the lowest in the oxidation group (p<0.05, Fig. 1), whereas the FI was increased by TMP addition to the oxidation group (p<0.05), further increased by TMP in the treatment without oxidation (p<0.05), but no difference existed between the two non-oxidative treatments. Loss of tryptophan fluorescence is another marker for oxidation at protein core due to disrupted spatial structure by oxidative stress, while antioxidants could be protective to protein conformation and consequent tryptophan fluorescence (Liang et al., 2018; Yang and Xiong, 2018). The similar result in the present study indicated that TMP was effective in protecting oxidative stability and structural stability of MPs. Additionally, MPs interacted with pyrazine compounds and the interaction followed a combination of static and dynamic quenching probably due to protein aggregation from the binding of MPs to 2,5-dimethylpyrazine (Shen et al., 2019).
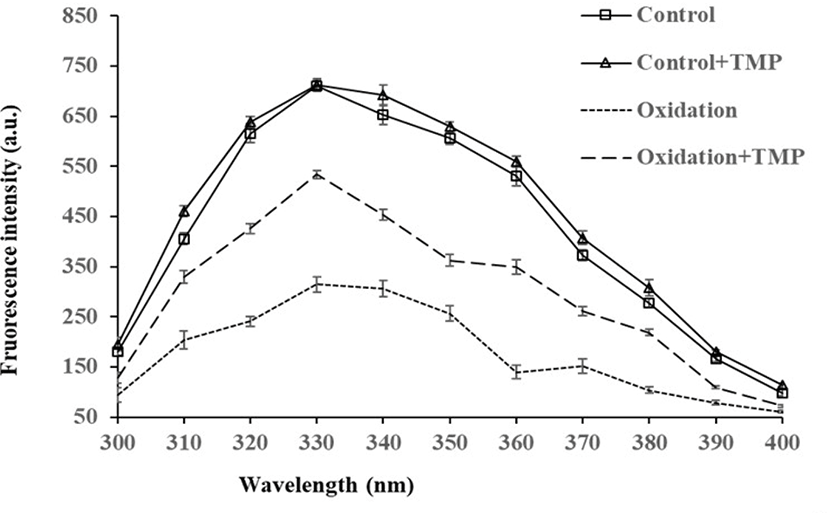
Compared to control, oxidation decreased bound water and immobilized water of MPs, but increased free water (p<0.01, Table 3), indicating that the two forms of water educed as free water, which leads to the decreased WHC of MPs gels. Without oxidation, TMP treatment increased bound water and decreased free water (p<0.01); concurrent with oxidation, TMP increased bound water and immobilized water, but decreased free water (p<0.01). Significant interactions were found between treatments TMP and oxidation (p<0.01).
The results in the present study further confirmed the protection of TMP on oxidative stability of MPs gels. With oxidative stress, proteins will accompany a serial of changes, including modified protein side-chain; unfolded structure; β-turn and random coil from myosin α-helix; and loosed spatial structure (Wang et al., 2016). All those changes can contribute to the decrease of bound water and immobilized water. Reversely, TMP treatment protected myosin spatial structure and the water retention capacity of rabbit MPs gels. Shen et al. (2019) reported that the binding of 2,5-dimethylpyrazine from the interaction of pyrazines and MPs increased the α-helix content of MPs depending on varied pH values, and this was further demonstrated by Yang et al. (2017a) using alcohols, aldehydes, ketones, and esters. Therefore, it is interesting to further study how TMP as a curing agent influences the α-helix content of MPs at a varied pH condition.
Conclusion
Oxidative stress to rabbit MPs worsened physiochemical and gelation properties. Without oxidative stress, TMP increased free thiol content, whiteness, and bound water, but decreased dityrosine content and free water; under oxidative conditions, TMP inversely influenced these parameters. The results suggest that TMP can be an antioxidant to improve the oxidative stability, water retention, and gel forming property of rabbit MPs.