Introduction
Coffee is one of the most popular beverages worldwide. The consumption of coffee has increased steadily in the last 50 years. In general, consumers report that flavor is one of the essential determinants of their choice of coffee. Coffee flavoring involves numerous chemicals, and specific flavors are generated during the coffee bean roasting process (Caporaso et al., 2018; Dmowski and Dabrowska, 2014; Sunarharum et al., 2014). The volatile compounds that impart the coffee flavor include furan, pyrazine, ketone, hydrocarbon, aldehyde, alcohol, esters, oxarole, thiopene, thiazole, and phenol (Clarke and Macrac, 1987). The flavor of coffee, however, is likely to be lost during storage due to oxidation. Changes in the coffee flavor during storage occur in three steps that include loss of volatile compounds, reactions among the coffee components, and oxidation (Sievetz, 1985). During these steps, oxygen, moisture, and temperature mainly affect the degradation processes (Sievetz and Desroier, 1980). The changes in the coffee flavor directly influence the sensory evaluation of coffee taste (Moon and Cho, 1999). Thus, maintaining the coffee flavor for as long as possible during its shelf-life is considered to be the best way to retain the quality and sensory properties of coffee beverages. In this regard, Moon and Cho (1999) proposed vacuum or nitrogen-filled packaging for coffee products. There are, however, some restrictions on applying these techniques to coffee beverages, despite the suitability of these methods for use on coffee beans.
Microencapsulation is defined as a process by which liquid, solid, or gas materials are coated with polymeric materials (Roshan et al., 2016). This method is advantageous in that it allows for sustained release of the core material, conversion of dosage form, enhancement of drug stability, reduction in gastrointestinal irritation, improved taste masking, stabilization in the presence of oxygen, and increased bioavailability of the core material (Langer, 1998; Lu et al., 2007). Microencapsulation of indomethacin, acetaminophen, aspirin, insulin, and other drugs has been studied in regard to sustained-release properties and stabilization in the presence of oxygen characteristics (Roshan et al., 2016; Liu et al., 2004; Mutaliyeva et al., 2017). Similarly, microencapsulation of vitamins, flavors, probiotics, and enzymes has been studied for use in food processing (Ahn et al., 2019; Liu et al., 2001; Shah and Ravula, 2000; Uddin et al., 2001). Microencapsulation of flavor could protect the flavor components from unwanted chemical changes and may retain the flavor during manufacture or storage (Shahidi and Han, 1993). Additionally, it can control the release of the flavors during storage (Reneccius, 1995). Currently, studies regarding the microencapsulation of coffee flavor to extend flavor retention are lacking. Therefore, the objective of this study was to determine the feasibility of microencapsulation in prolonging flavor retention of coffee beverages during storage.
Materials and Methods
Caramel flavors (KM90202 and SY20733) and a coffee-containing milk beverage that is currently commercially available were provided by Seoul F & B Co., Ltd. (Hoengseong, Korea). The beverage was composed of 30% raw milk, 28% coffee extract, 29% purified water, 6% sucrose, and 0.03% emulsifier. Maltodextrin and medium-chain triglycerides (MCT) used as coating materials were purchased from Samyang Corp. (Seoul, Korea) and Ilshin Wells Co., Ltd. (Seoul, Korea), respectively. Emulsifiers such as polyglycerol polyricinoleate (PGPR) and polyoxyethylene sorbitan monolaurate (PSML) were provided by Ilshin Wells Co., Ltd. Pepsin and pancreatin were purchased from Sigma-Aldrich Co. (St. Louis, MO, USA).
To determine the optimal amount of emulsifiers for water-in-oil (W/O) and water-in-oil-in-water (W/O/W) emulsification of caramel flavor, the ESIs were determined using a volumetric method described by Chang et al. (1994) with minor modification. The ratio of core to coating material was fixed at 1:9, and the emulsions were prepared by addition of 0.25%, 0.520%, 0.75%, and 1.00% of the primary emulsifier (PGPR) and the secondary emulsifier (PSML). The emulsions were placed in a 55°C incubator for 20 h to observe layer separation. The ESI was computed using the following equation.
To optimize the microencapsulation of caramel flavor, response surface methodology (RSM) was employed. The ratios of caramel flavor to MCT for the primary emulsification and the primary emulsion to maltodextrin for the secondary emulsification, a process that could affect the microencapsulation process, were replaced by variables X1 and X2, respectively. They are indicated as −1, 0, and +1 in the range from 1:9 to 4:6, as shown in Table 1. The faced central composite design (FCCD) approach was used to design the composition of the two variables. Thirteen experimental settings were designed with 2 factors and 3 levels by FCCD, and analyses were performed using Minitab 16 and Design expert 7. The microencapsulation yield is expressed as a dependent variable Y in the regression as follows:
Variables | Coded Xi | Coded level | ||
---|---|---|---|---|
−1 | 0 | +1 | ||
Ratio of core to primary coating material | X 1 | 1.0:9.0 | 2.5:7.5 | 4.0:6.0 |
Ratio of primary emulsion to secondary coating material | X 2 | 1.0:9.0 | 2.5:7.5 | 4.0:6.0 |
where, the dependent variable Y represents the yield of micro encapsulation, and β0, β1, β2β12, β11, and β22 are coefficients of intercept, linear, interaction and quadratic terms, respectively. This response surface model was employed to predict the result by iso-response three-dimensional (3-D) surface plots.
For the primary emulsification, the caramel flavor (KM90202 or SY20733) was diluted to 50% using distilled water (DW). This was then mixed with MCT as a primary coating material and PGPR as a primary emulsifier at a constant ratio. The mixture was homogenized using a high-speed homogenizer (Ultra-Turrex T-25, Ika Werke & Co. KG, Staufen, Germany) at 17,500 rpm to obtain the primary emulsion (W/O phase). The primary emulsion was mixed with the secondary coating material, and this mixture was homogenized at 6,500 rpm to obtain the secondary emulsion (W/O/W phase). The secondary emulsion was spray-dried and microencapsulated with a spray drier (SD-100, Tokyo Rikakikai Co., Ltd., Tokyo, Japan). The spray drying conditions included a feed flow rate of 0.5 L/h, an inlet temperature of 170°C, an airflow rate of 0.70 m3/min, and an atomizing pressure of 50 kPa.
In the present study, it was found that the caramel flavors exert an antioxidant effect. Consequently, total polyphenol content was determined to measure the microencapsulation yield, and this yield was measured from the unencapsulated portion within the W/O/W emulsion using the indirect method. To determine total polyphenol content, 0.1 mL of sample was mixed with 0.2 mL of 2 N Folin & Ciocalteau’s phenol reagent and 2 mL of DW. After 3 min incubation, 2 mL of 20% Na2CO3 was added, and the mixture was incubated in the dark for 1 h. Subsequently, the absorbance was measured at 765 nm. The microencapsulation yield was calculated using the following equation.
Simulated gastric fluid (SGF) was prepared according to the method of Luan et al. (2006). Pepsin (0.0032 g) was dissolved in 10 mL 0.03 M NaCl, and the pH was adjusted to pH 1.2 by the addition of 1 N HCl. The microcapsules were added to the SGF at a concentration of 0.2 g/mL. The mixture was incubated in a 37°C shaking water bath at 120 rpm. Samples were collected every 30 min for 2 h. The collected samples were immersed in ice for 10 min or more to terminate the enzyme reaction. The collected sample was then filtered through a 0.45-μm syringe filter, and total polyphenol content was assayed.
The simulated intestinal fluid (SIF) was prepared according to the method of Papagianni and Anastasiadou (2009) with minor modification. To produce SIF, 0.04 g pancreatin and 0.25 g deoxycholate were dissolved in 10 mL 0.1 M sodium bicarbonate. The SGF-treated samples and SIF were transferred into phosphate buffer (pH 7.4) at a concentration of 0.2 g/mL, and this mixture was incubated in a 37°C shaking water bath at 120 rpm. Sample collections and analyses were performed as described above.
Volatile compounds from normal coffee-containing milk beverage (control), caramel flavoring, or the caramel flavor microcapsules were collected and analyzed using headspace solid-phase microextraction (HS-SPME)/GC-FID at 30°C. Samples were collected 6 times over 15 days. To analyze the volatile compounds adsorbed by SPME fibers, ACME 6500 gas chromatography (Younglin Co., Korea) was used. The analysis conditions for the GC assay are listed in Table 2.
Results and Discussion
Fig. 1 illustrates the changes in ESIs after the addition of various amounts of PGPR and PSML during the primary and secondary emulsifications, respectively. For the primary emulsification, the ESI was slightly increased by 0.5% PGPR addition; however, this increase was not statistically significant (p>0.05). Upon 0.75% PGPR addition, the ESI significantly increased (p<0.05), and the ESI was 90.12% after the addition of 1.0% PGPR. These findings were in agreement with those of Ahn et al. (2013), who reported that ESI tended to increase as the amount of PGPR increased. Further, Park et al. (2006) reported that increased PGPR concentrations induced increases in ESI.
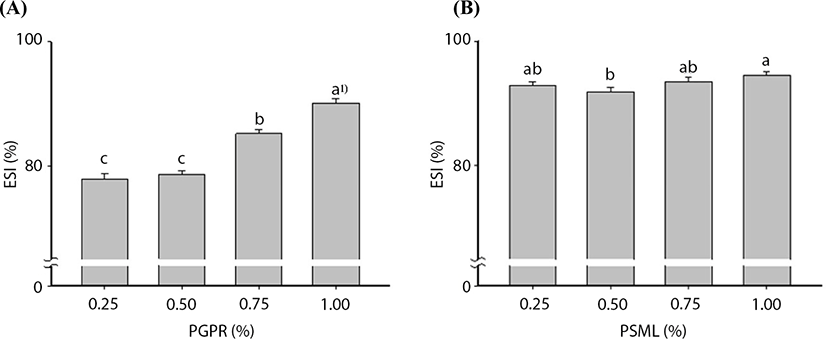
For W/O/W emulsification, the ESI slightly increased as the addition of PSML increased. This increase, however, was not statistically significant (p>0.05). According to Ficheux et al. (1998) and Pays et al. (2001), the presence of high amounts of hydrophilic emulsifiers such as PSML can destroy the oil layer and cause the release of inner aqueous core material in W/O/W double layered microencapsulation. Given this, we believed that the addition of 0.25% (w/v) PSML was reasonable for this study.
Based on FCCD, the ratios of core to coating material (X1) and the ratio of primary emulsion (W/O) to secondary coating material (X2) were set as independent variables, and microencapsulation yield (Y) was employed as a dependent variable. A total of 13 experiments were performed (Table 3), and the results are listed in Table 4. As shown in in Table 4, constant, linear, and interaction terms were highly significant (p<0.05), while quadratic terms showed no significance (p>0.05). Consequently, the quadratic term was removed, and the following regression could be obtained based on this result.
In Eq. (1), X1 (ratio of core to primary coating material), X2 (ratio of W/O to secondary coating material), and X1X2 exhibited a negative relationship to microencapsulation yield. According to Simmons (1978), a negative coefficient exhibits an antithetic effect with the dependent variable. This indicates that the microencapsulation yield increases as these parameters decrease. Fig. 2 presents a 3-D surface plot of RSM for the microencapsulation of caramel flavor based on the results of the RSM. As mentioned above in connection with the Eq. (1), as the ratios of the core to primary coating material and W/O to secondary coating material decreased, the microencapsulation yield tended to increase. Taking all conditions into account, we determined the optimized condition for caramel flavor microencapsulation (Fig. 3). When the ratio of the caramel flavor as a core material to the primary coating material MCT was 1:9 and W/O emulsion to the secondary coating material was 1:9, the microencapsulation yield of caramel flavor was approximately 93.43% and its composite desirability was approximately 82.59%.
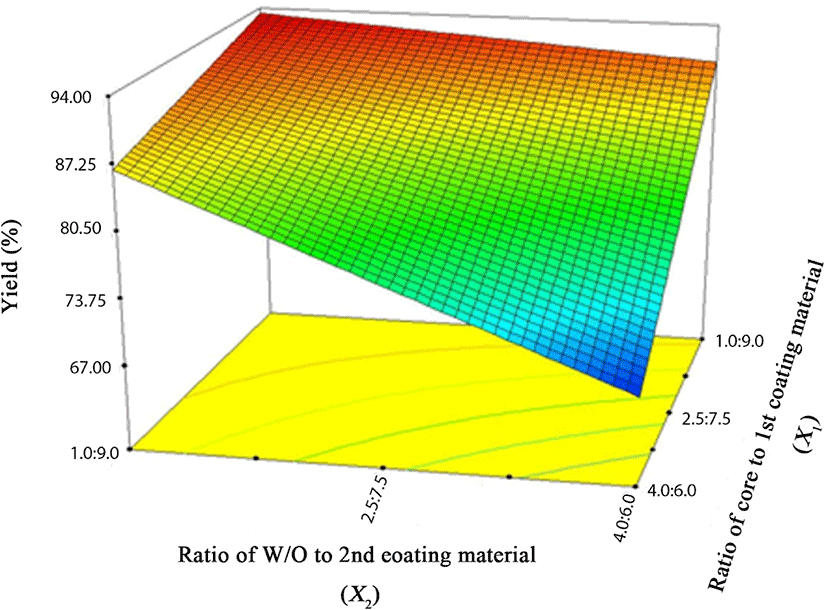
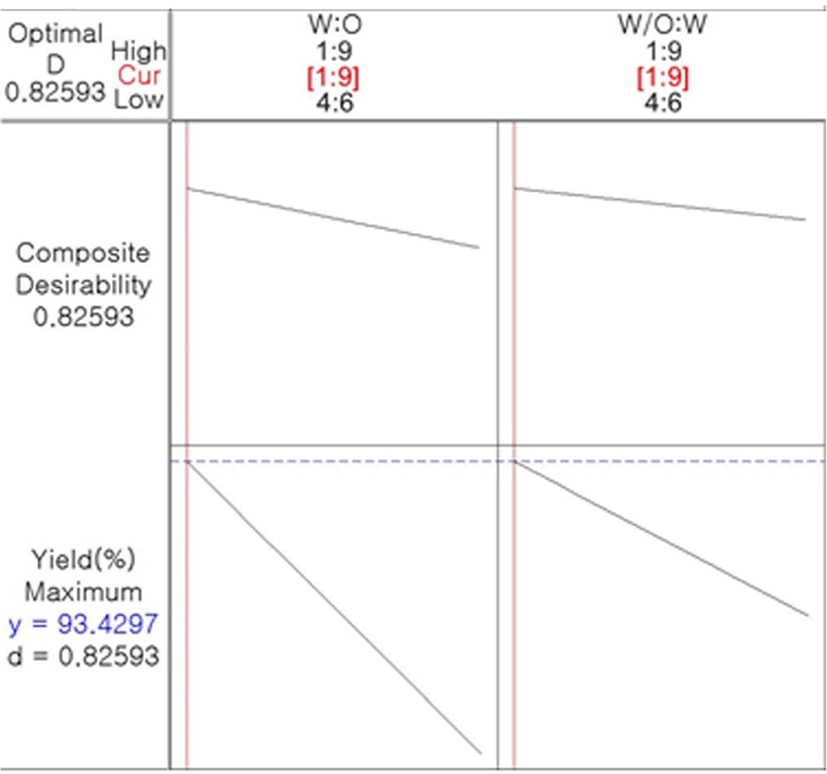
As mentioned above, an antioxidant effect was detected in the caramel flavors. Consequently, in vitro release properties were investigated, as it is necessary that these antioxidant compounds of the caramel flavors can safely pass through a gastric environment and release the compounds into the intestine. As shown in Fig. 4, the caramel flavor microcapsules released approximately 4% of their core material into SGF at 120 min. Subsequently, this material was transferred into SIF, and the release rate in the simulated intestinal environment was investigated. The release rate rapidly increased at 30 min of digestion time, and it steadily increased for 120 min in SIF. Ultimately, approximately 69.6% of the core material was released from the microcapsules. Sansone et al. (2011) reported that the release rate of the core material was approximately 40% to 90%, and this rate depended on the ratio of maltodextrin to pectin as a coating material. In the present study, we thought it likely that the release properties of caramel flavor as a core material could be controlled when the maltodextrin was mixed with other materials such as pectin, WPI, and gum Arabic. Subsequently, microencapsulation would be useful to deliver the caramel flavor compounds that possess an antioxidant effect into the intestinal tract.
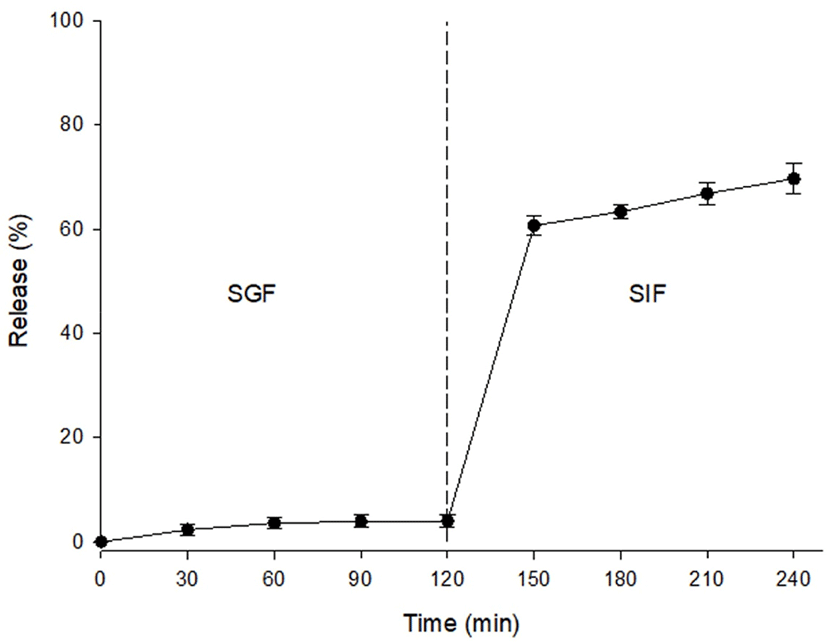
Figs. 5 and 6 illustrate the changes in volatile compounds of non-added milk beverage supplemented with coffee (NC), caramel flavoring (FC), or caramel flavor microcapsules (CC) under 30°C accelerated conditions. In the GC-chromatogram presented in Fig. 5, it is revealed that the main volatile compound of caramel flavor can be detected at 14.5 min. As shown in Fig. 5D–F, the caramel flavor was not detected upon initial and after 15 d-of storage; however, the detection of the flavor was confirmed at the 9th storage day in CC. More detailed release properties of the volatile compounds during storage are provided in Fig. 6. The FC possessed a high amount of volatile compounds at the initial time point; however, the peak area of the volatile compounds rapidly decreased during storage, and the amount of caramel flavor reached zero at the 12th storage day. Moon and Cho (1999) reported that roasted coffee flavor was exponentially degraded during storage, and this is in agreement with the result of the present study. In the case of CC, the volatile compounds were detected at low levels at the initial time point. The peak area gradually increased, and the highest peak area was observed at the 9th storage day. After this time point, the peak area gradually decreased.
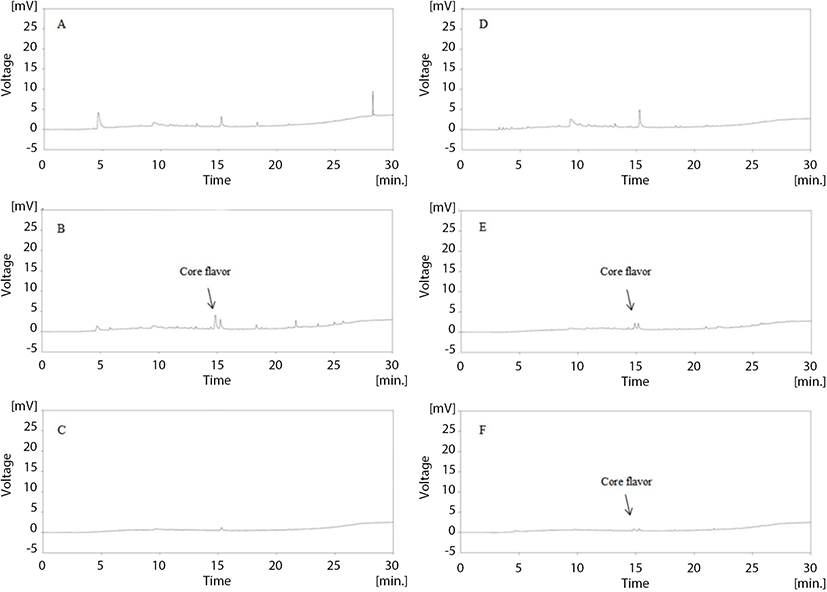
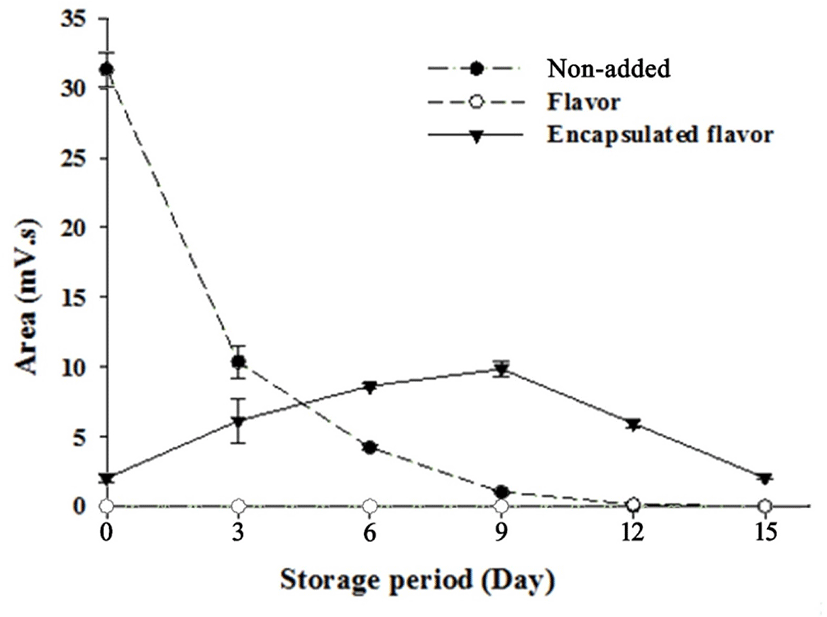
As mentioned above, Sievetz and Desroier (1980) stated that the degradation of coffee flavor is related to oxygen, temperature, and moisture. Radtke and Pringer (1981) observed that fresh coffee flavor could last approximately 2 wk at 21°C in the presence of oxygen, which is similar to the results of our study. Additionally, microencapsulation is known to preserve core material from unwanted environmental conditions (Roshan et al., 2016). Given this, it is likely that the encapsulated caramel flavor was effectively protected from head-space oxygen by microencapsulation, and this could contribute to prolonging coffee flavor.
In this study, the microencapsulated caramel flavor exhibited sustained release and was protected from oxygen, and the microcapsules that contained sample could maintain coffee flavor for a longer period than the control. Therefore, it is likely that the encapsulated caramel flavor sample could maintain its flavor for a longer period under normal refrigerated conditions.
Conclusion
This study suggested a method using microencapsulation technology to ameliorate the quality of coffee beverages by increasing the retention of the coffee flavor. The in vitro release characteristics of the capsules, which were rapidly released into the simulated intestinal environment, were confirmed. Additionally, the microencapsulated flavors, when added to beverages, exhibited properties of sustained release during storage. Based on the results from the present study, the microencapsulation of flavor and addition of these microcapsules to coffee beverages was effective for prolonging flavor retention and improving the quality of the beverages. The physicochemical properties of the capsules, however, require further study prior to their use in coffee beverages.