Introduction
Cellular senescence, a permanent and irreversible state of cell cycle arrest, show distinctive phenotypic changes in morphology and gene expression (Hayflick, 1965; Pazolli and Stewart, 2008). Following a limited number of cell divisions, primary cells undergo replicative senescence characterized by accelerated attrition of telomeres that eventually lead to the incomplete chromosomal replication (Harley et al., 1990). Unlike replicative senescence, stress-induced premature senescence is induced by diverse factors that cause cellular stress such as angiotensin II (Ang II), ultraviolet radiation, and hydrogen peroxide (Toussaint et al., 2000; Touyz and Schiffrin, 2000). Recently, Ang II was reported to trigger aging of vascular smooth muscle cells (VSMCs) by causing oxidative DNA damage which is intimately linked to the stability of atherosclerotic plaques (Herbert et al., 2008; Matthews et al., 2006). These findings are consistent with the reports that blockade of Ang II activity by polyphenols, such as resveratrol and those found in berries, inhibits vascular senescence-mediated intracellular signaling, leading to blockade of vascular age-associated diseases including atherosclerosis (Feresin et al., 2016; Kim et al., 2018; Najjar et al., 2005). Thus, aging-related vascular disorders may be prevented by controlling cellular senescence. As a potential candidate among diverse anti-senescence factors, the NAD-dependent deacetylase SIRT1 has a pivotal function in cardiovascular systems and is highly expressed (Potente et al., 2007). SIRT1 elicits beneficial effects on neointima formation, vascular remodeling, and atherosclerosis by inhibiting stress-induced cellular senescence (Gao et al., 2014; Kim et al., 2012; Li et al., 2011b). Exacerbated DNA damage and senescence are observed in VSMCs located in atherosclerotic regions, in which SIRT1 expression is reduced (Gorenne et al., 2013, Zhang et al., 2008). In addition, our previous studies showed that peroxisome proliferator-activated receptor δ-mediated induction of SIRT1 expression suppresses Ang II-triggered premature senescence of human VSMCs and endothelial cells (Kim et al., 2011; Kim et al., 2012). Thus, molecules that upregulate expression of the anti-senescence protein SIRT1 alter the pathological cardiovascular conditions caused by aging of vascular cells (Gorenne et al., 2013; Ota et al., 2008).
Duck oil is an avian oil that derived from duck skin, a by-product of duck meat processes (Shin et al., 2019). Recent report has shown that duck skin-derived oil contains a higher amount of long-chain fatty acids including oleic acid (18:1) and linoleic acid (18:2) than other animal skin fats, such as chicken, swine, bovine (Shin et al., 2019). In fact, long-chain fatty acids have been shown direct beneficial effects in the prevention and treatment of many diseases, such as diabetes, obesity, and cardiovascular disorders (Fuke and Nornberg, 2017; Massaro and De Caterina, 2002). Furthermore, duck oil showed a high unsaturated fatty acid/saturated fatty acid ratio (above 50%) compared with fats derived from swine and bovine, indicating usefulness of duck oil in food industries (Shin et al., 2019). However, the biological activity of duck oil has not been experimentally elucidated. Consequently, we investigated the effects of duck oil in the vascular aging processes. We here demonstrate that duck oil derived from duck skin inhibits premature senescence of VSMCs triggered by Ang II by upregulating SIRT1.
Materials and Methods
Duck skin was obtained from Farm Duck Co. (Jeongeup-si, Korea). A pressurized hot water extraction method was used to isolate the oil as described previously (Plaza & Turner, 2015). In detail, skin samples from eight individual duck were washed several times with distill water and connective tissues and visible fat were removed from the skin. Following these procedures, a pressure extractor was applied to extract oil from the skin by heating the skin at 115°C under 1.4 kgf/cm2. Following treatment for 3 h, the samples were dehydrated for 30 min and then filtrated with a 40-mesh bucket filter. Finally, the oil phase (supernatant) was obtained by centrifugation of the filtrate at 14,500×g for 1 h.
Duck oil-loaded nanoemulsion (DO-NE) was generated by mixing 20% (w/w) duck oil in medium chain triglyceride oil (NOW Foods, Elmhurst, IL, USA) containing the hydrophobic emulsifier soy lecithin (ESFOOD, Gunpo, Korea). Distilled water was mixed with the hydrophilic emulsifier Tween-80 (Daejung Chemical, Seoul, Korea) to generate the aqueous phase. After mixing using a magnetic bar for 2 h, the samples were sequentially homogenized (550×g, 10 min), ultrasonicated (15 min), and exposured to high pressure (10,000 psi, three times) to prepare nanoemulsions (NE). The same procedure without duck oil was performed to generate control NE.
A particle size and zeta potential analyzer was used to determine the polydispersity index (PDI), mean droplet size, and zeta potential of NEs. Specifically, a polystyrene latex cell containing 1 mL NE was analyzed using a detector angle of 90° and a wavelength of 633 nm at 25°C to determine PDI and mean droplet size. A droplet of diluted NE was placed on a copper grid and briefly dried. After negative staining with phosphotungstic acid, the grid was dried overnight and then images were acquired using a transmission electron microscope at 110 kV and at a magnification of 70 K.
Primary VSMCs were isolated from the thoracic aorta of a rat as described previously (Hwang et al., 2016). Briefly, the aorta of a male Sprague-Dawley rat was dissected longitudinally. Endothelial cells were removed and the medial layer of the aorta was chopped into small fragments and incubated up to 3 d. Fresh medium was then added, and fragmented tissues were incubated for several further days. Thereafter, migrated VSMCs were collected and passaged in Dulbecco’s modified Eagle’s medium containing antibiotics and 10% fetal bovine serum using routine cell culture procedures. VSMCs employed in this study were originally isolated in 2008. Cells at passage 4-8 were used in the present study.
The MTT assay was performed to assess cell viability as described previously (Yoo et al., 2016). Specifically, the indicated concentration of NE or DO-NE was added to VSMCs plated into 24-well plates, and cultures were incubated for 24 h. Thereafter, the cells were further incubated in culture medium containing 0.1 mg/mL MTT for 2 h. After removal of the medium, crystalized formazan was dissolved in acidified isopropanol and the optical density was measured at 570 nm.
VSMCs cultured in 60 mm dishes were pretreated with NE or DO-NE for 24 h. Thereafter, the cells were exposed to vehicle (Dimethyl sulfoxide, DMSO) or Ang II for 72 h, washed briefly with cold phosphate-buffered saline, and then stained with a solution for 24 h at 37°C as described previously (Kim et al., 2012). Fluorescence microscopy was performed to detect stained cells. The number of stained cells in four individual captured images was counted on a computer monitor using a DS-Ri2 camera equipped to Nikon Eclipse Ti2 inverted fluorescence microscope (Tokyo, Japan) by an independent observer.
SDS-polyacrylamide gel electrophoresis was performed using whole-cell lysates of VSMCs treated with the indicated reagents, and then immunoblotting was conducted using specific antibodies. Specifically, the membranes were blocked with 3% skim milk prepared in Tris-buffered saline containing 0.1% Tween-20 for 2 h at ambient temperature. Thereafter, the membranes were reacted with a specific antibody at 4°C overnight and then with a secondary antibody conjugated with peroxidase at room temperature for 2 h. Signals were detected by enhanced chemiluminescence as described previously (Hwang et al., 2016). The band intensity of blots was quantified by image J software (NIH, Bethesda, MD, USA) and normalized using loading control.
The mouse-specific SIRT1 promoter (−2,487 to −30 in pGL4) was obtained from Dr. Toren Finkel [National Institutes of Health (NIH), MD, USA]. VSMCs were co-transfected with the SIRT1 luciferase reporter plasmid (1 μg) and the SV40 β-galactosidase expression vector (0.5 μg) and incubated for 24 h. Following exposure to different concentrations of DO-NE for 72 h, the cells were lysed and promoter activity was measured. β-galactosidase activity was used to normalize variations in the transfection efficiency between samples.
Results and Discussion
The solubility and biological performance of nanoparticles are affected by the physicochemical properties of NEs (Ghasemiyeh and Mohammadi-Samani, 2018). Therefore, we analyzed the zeta potential, PDI, and droplet size of the NEs. The mean droplet size and zeta potential of the NEs ranged from 104 to 192 nm and from −30 to −41 mV, respectively, and the NEs were of a uniform size and had a spherical shape without any flocculation (Fig. 1).
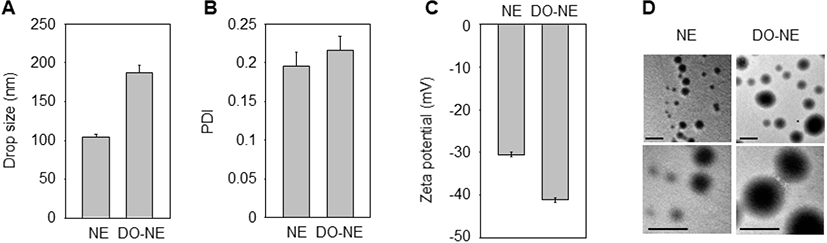
To determine the optimal concentrations of the NEs for treatment of VSMCs, the MTT assay was performed to assess cell viability. Treatment with increasing concentrations (0, 50, 100, 250, 500, and 1,000 μg/mL) of NE and DO-NE for 24 h did not significantly affect cell viability (Fig. 2). Accordingly, the NEs were used at a concentration of 1 mg/mL in subsequent experiments.
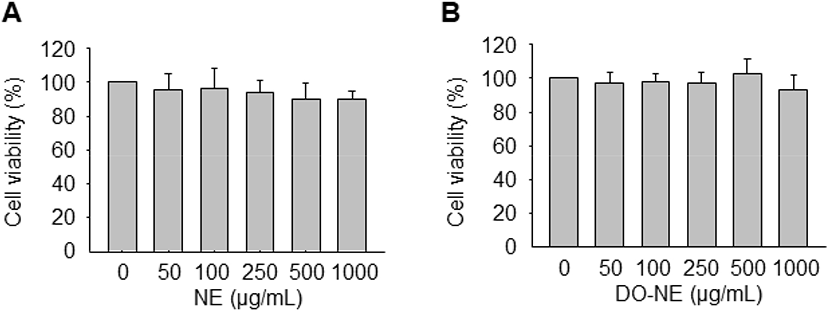
Next, we examined whether DO-NE affects Ang II-triggered senescence of VSMCs. SA β-gal activity, a senescence biomarker, in the sham group was significantly higher in VSMCs treated with Ang II (63.63±5.17, p<0.01) for 3 days than in cells exposed to DMSO (4.22±1.09). However, this increase was significantly suppressed by treatment with DO-NE (21.77±2.38, p<0.01) but not by treatment with NE (54.42±2.76; Fig. 3A–B). These findings are consistent with the previous report that oils from avian species, such as emu and duck, contain a high ratio of unsaturated fatty acids to saturated fatty acids and protect against oxidative damage in a model biological membrane system (Bennett et al., 2008). Additionally, the triglyceride concentration in the serum and liver of rats is decreased more by feeding of duck oil than by feeding of soybean oil (Koh et al., 1995). Although the molecular basis of the anti-senescence effects of duck oil in the vascular system is unknown, our results suggest that duck oil elicits beneficial effects on senescence of VSMCs stimulated by Ang II and can therefore be potentially used as a functional food.
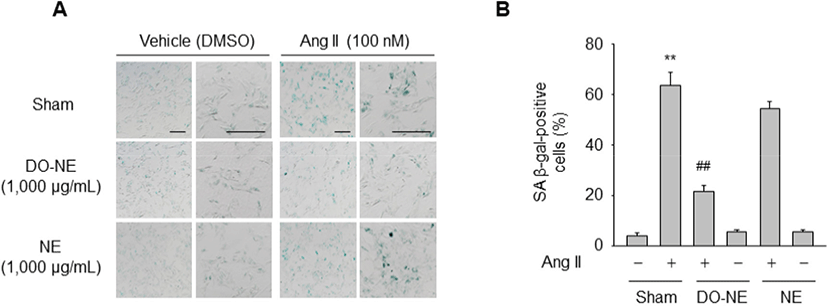
The pro-longevity gene SIRT1 is implicated in lifespan modulation in various organisms ranging from yeast to mammals (Haigis and Sinclair, 2010). SIRT1 was directly implicated in the modulation of cellular aging by deacetylating critical transcription factors such as forkhead box protein and p53, thereby repressing the transcription of Ang II type I receptor as demonstrated in VSMCs (Brunet et al., 2004; Langley et al., 2002; Miyazaki et al., 2008). We examined the effects of duck oil on SIRT1 expression in VSMCs. DO-NE, but not NE, dose- and time-dependently enhanced the expression level of SIRT1 protein, as expected (Fig. 4A–C). The level of SIRT1 protein was highest upon treatment with 1,000 μg/mL DO-NE for 72 h (2.03±0.14, p<0.01). When VSMCs were treated with 1,000 μg/mL DO-NE, SIRT1 expression was significantly increased at 48 h (1.85±0.20, p<0.05) and this persisted for up to 72 h (2.36±0.29, p<0.01).
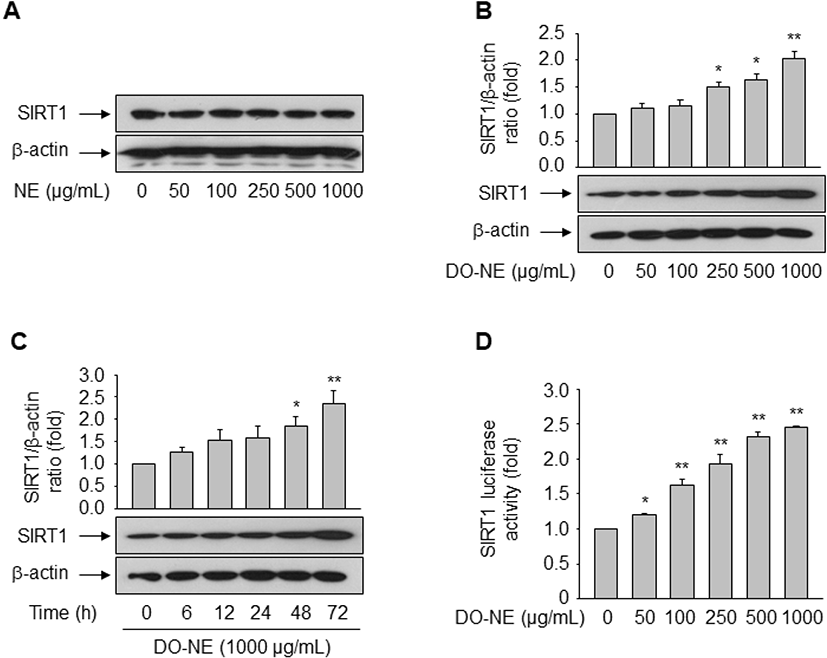
No previous report has shown that duck oil affects expression of SIRT1. Hence, we investigated whether duck oil regulates transcription of the SIRT1 gene. To this end, VSMCs were transfected with a luciferase reporter construct containing the SIRT1 promoter. As shown in Fig. 4D, DO-NE dose-dependently enhanced SIRT1 promoter activity from 50 μg/mL DO-NE (1.21±0.01, p<0.05) to 1,000 μg/mL DO-NE (2.45±0.01, p<0.01). This result is consistent with the induction of SIRT1 protein expression by DO-NE, indicating that duck oil transcriptionally regulates expression of SIRT1 in VSMCs. In fact, SIRT1 is reported to modulate diverse biological processes, including energy metabolism, stress responses, inflammation, and aging (Brunet et al., 2004; Cohen et al., 2004; Feige and Auwerx, 2008; Yeung et al., 2004). In addition to the role of caloric restriction in SIRT1 regulation (Cohen et al., 2004), diverse cellular molecules such as p53, the HIC:CtBP co-repressor complex, E2F1, cAMP response element-binding protein, breast cancer 1, and TLX are associated with modulation of SIRT1 transcription (Chen et al., 2005; Iwahara et al., 2009; Noriega et al., 2011; Wang et al., 2006; Wang et al., 2008; Yi and Luo, 2010). We previously demonstrated that peroxisome proliferator-activated receptor δ, a ligand-dependent nuclear receptor, induces SIRT1 transcription in rat VSMCs and human endothelial cells, thereby regulating cellular senescence and proliferation triggered by the atherogenic-molecule oxidized low-density lipoprotein and Ang II, respectively (Hwang et al., 2016; Kim et al., 2012). Additionally, the present study clearly demonstrates that duck oil also induces SIRT1 expression.
To examine the direct impact of duck oil-mediated induction of SIRT1 on suppression of VSMC senescence triggered by Ang II, expression of endogenous SIRT1 was monitored in VSMCs exposed to Ang II with or without DO-NE. Treatment with Ang II time-dependently suppressed the level of endogenous SIRT1 protein from 24 h (0.58±0.08, p<0.05; Fig. 5A). However, DO-NE dose-dependently reversed this effect from 100 μg/mL DO-NE (p<0.01), indicating that induction of SIRT1 protein is essential for DO-NE-mediated suppression of VSMC senescence triggered by Ang II (Fig. 5B).
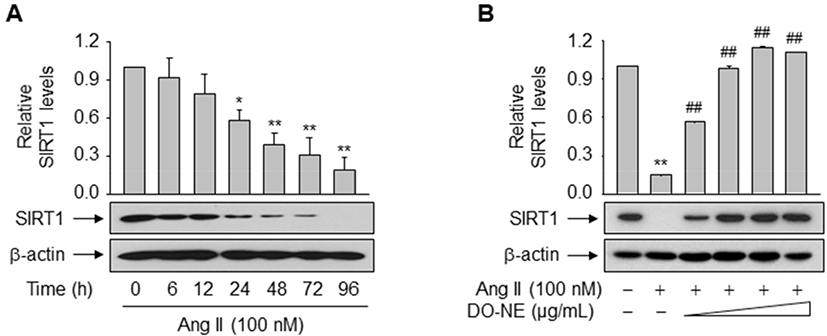
To further elucidate the importance of SIRT1 induction for inhibition of Ang II-triggered VSMC senescence by DO-NE, cells were treated with reagents that modulate SIRT1 activity. The number of SA β-gal-positive cells was lower among VSMCs exposed to Ang II and resveratrol (11.50±0.76, p<0.01) than among VSMCs treated with Ang II alone (37.10±1.95; Fig. 6). Furthermore, the inhibitory effect of DO-NE was potentiated by addition of resveratrol to DO-NE-treated VSMCs (9.03±0.09, p<0.01). By contrast, addition of sirtinol, a specific inhibitor of SIRT1, significantly counteracted the DO-NE-mediated reduction of SA β-gal-positive cells in Ang II-treated cells (28.46±1.89, p<0.01). These observations indicate that the anti-senescence activity of DO-NE in Ang II-treated VSMCs is attributable, at least in part, to DO-NE-mediated induction of SIRT1, a protein that is associated with increased lifespan in mammals (Haigis and Sinclair, 2010). Although the detailed mechanisms by which DO-NE induces expression of SIRT1 are unclear, chemical-mediated modulation of SIRT1 activity clearly indicated that DO-NE inhibits senescence of VSMCs triggered by Ang II in a SIRT1-dependent manner. Consistent with this anti-senescence effect of DO-NE, a previous study demonstrated that expression of a VSMC-specific SIRT1 transgene inhibits Ang II-induced vascular remodeling (Gao et al., 2014). Additionally, ectopic expression of SIRT1 using an adenovirus markedly suppresses hypertrophy of VSMCs triggered by Ang II (Li et al., 2011a). Furthermore, knockout of SIRT1 in VSMCs promotes Ang II-induced vascular cell senescence, thereby accelerating formation and rupture of abdominal aortic aneurysms in apolipoprotein E knockout mice (Chen et al., 2016). These findings suggest that DO-NE-mediated upregulation of SIRT1 is associated with the usefulness of duck oil as a potential functional food to prevent aging-related vascular diseases.
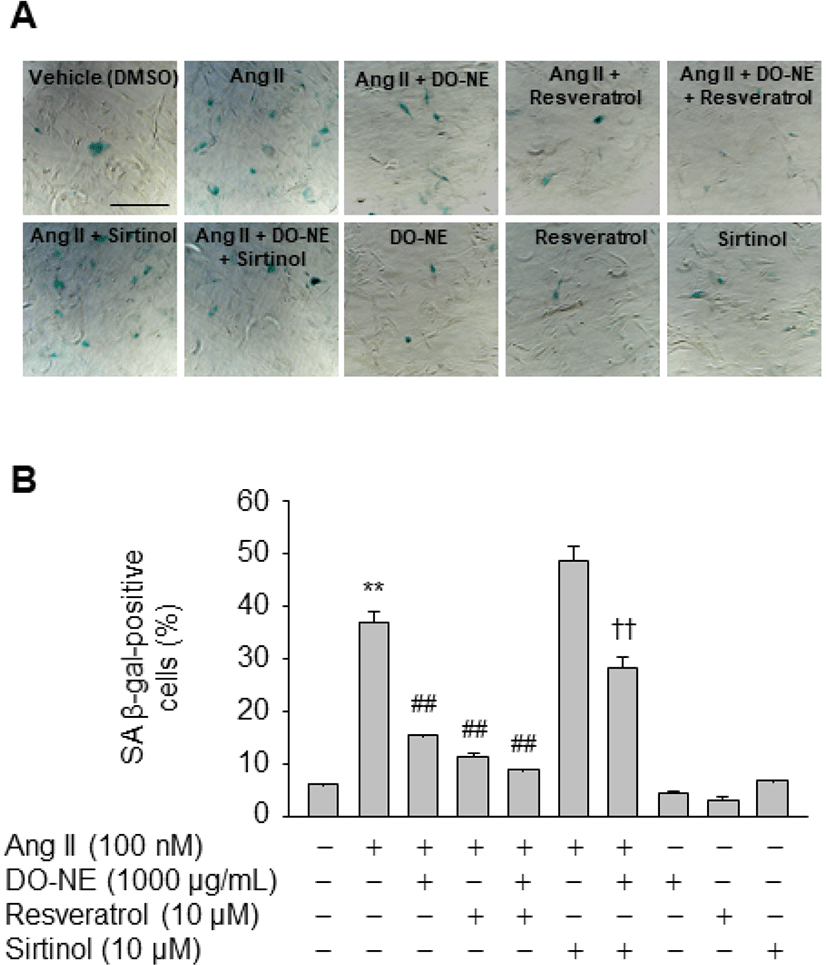
Conclusion
To obtain scientific evidence of the biological activity of duck oil, we examined the effects of this oil on vascular senescence triggered by Ang II, a vasoactive hormone. The present study demonstrates that DO-NE suppresses Ang II-stimulated senescence of VSMCs by increasing transcription of SIRT1. The present work provides the first clue that induction of SIRT1 by DO-NE suppresses vascular aging. This novel evidence provides insights into the anti-senescence effect of duck oil and the detailed mechanisms underlying SIRT1 expression. The anti-aging activity of duck oil in vascular systems supports the potential use of this oil as a functional food to improve vascular functions.