Introduction
The muscle stem cells that are responsible for the regeneration of muscle tissues include quiescent satellite cells and its progeny, such as activated satellite cells, the so-called myoblasts (Motohashi et al., 2012). Quiescent satellite cells reside beneath the basal lamina of myofibers, upon injury, they are activated into proliferating myoblasts via growth factor stimulation and differentiation forward to myocytes and myotubes. The quiescent satellite cells highly express Pax7, but not Myf5 or MyoD (Kuang et al., 2007). Stimulations, such as muscle injury, induce myogenic commitment via the activation of Myf5 and MyoD and inactivation of Pax7, which results in the activation of satellite cells into proliferating myoblasts. With the downregulation of Pax7, MyoG is subsequently expressed in myogenic precursors and completes the terminal differentiation (Jin et al., 2016). Muscle stem cells are generally cultured as proliferating myoblasts because the satellite cells cannot be maintained in vitro without losing their properties (Gilbert et al., 2010; Montarras et al., 2005). The in vitro culture of stem cells is accomplished by mimicking the in vivo cellular niche (Choi and Lee, 2019). To trigger proliferation in vitro, the isolated satellite cells are cultured with various cytokines and signaling molecules, which are secreted from the surrounding cells, such as stromal and interstitial cells, and muscle stem cells themselves (Yin et al., 2013).
The isolation procedure from muscle tissues is a type of muscle injury that triggers the activation of quiescent satellite cells into proliferating myoblasts within 24 hours via the upregulation of MyoD expression (Zammit et al., 2004). The proliferating myoblasts are most widely cultured with fibroblast growth factor 2 (FGF2)-supplemented media in vitro. FGF2 is secreted from stromal cells, such as fibroblasts, and it plays a role in the proliferation of the myoblasts in vivo (Groux-Muscatelli et al., 1990; Quinn et al., 1990). For in vitro culture, FGF2 treatment has a higher mitogenic effect on myoblasts compared to other growth factors, including insulin, insulin-like growth factors (IGFs), and leukemia inhibitory factor (LIF) (Gospodarowicz et al., 1976; Rando and Blau, 1994). IGFs and inflammatory cytokines also enhance proliferation and suppress myogenic differentiation during muscle stem cell culture. IGF-1 and IGF-2 play pivotal roles in the expansion of muscle stem cells in vivo and in vitro (Florini et al., 1984; Jennische et al., 1987). Pro-inflammatory cytokines produced from the infiltrated immune cells into the damaged area of muscle, such as interleukins, tumor necrosis factor, and interferons, support the maintenance of the stemness of muscle stem cells in vitro for an extended period (Fu et al., 2015). Several molecules that regulate the signaling pathways involved in the stemness and aging of muscle stem cells were also used, such as inhibitors of p38, Wnts, and dexamethasone (Bentzinger et al., 2013; Ding et al., 2018; Dodson et al., 1985).
Because the pig is a valuable candidate as a preclinical model of human cell therapy and an important food source, an understanding of the physiology of pig myogenic progenitors, such as skeletal muscle satellite cells and myoblasts, is required to cure muscular diseases and improve meat production. Muscle stem cells recently isolated from domestic animals, including cows and pigs, were spotlighted as candidates for the production of alternative protein resources, so-called cultured meat or lab-grown meat. Cultured meat is an artificial meat that imitates fresh meat using the in vitro production of muscle tissue, which would solve the problems derived from traditional meat production, including animal welfare and environmental pollution (Post, 2012). Various researchers used fetal bovine serum (FBS)-containing minimum essential medium (MEM) to culture pig muscle stem cells for a long time (Doumit and Merkel, 1992; Miersch et al., 2018). However, FBS-containing MEM-based culture conditions do not support the stemness of pig muscle stem cells for an extended period. Therefore, the present study optimized the in vitro culture conditions for the long-term expansion of pig muscle stem cells via the screening of various signaling molecules.
Materials and Methods
The Institutional Animal Care and Use Committee (IACUC) at Seoul National University approved the care and experimental use of pigs (approval no.: SNU-180612-2). The experiments were performed according to the standard protocol of the Institute of Laboratory Animal Resources at Seoul National University.
Pig muscle stem cells were isolated from the biceps femoris muscle of 3-d-old crossbred pigs (Landrace×Yorkshire×Duroc, LYD), which were euthanized via CO2 inhalation and exsanguination. The biceps femoris muscles were collected and washed with Dulbecco's phosphate-buffered saline (DPBS; Welgene, Gyeongsan, Korea) containing 2× antibiotic–antimycotic (AA; Gibco, Gaithersburg, USA), and excessive connective tissues and blood vessels were removed. The collected tissues were minced in a meat grinder and digested using 0.8 mg/mL Pronase (Sigma-Aldrich, St. Louis, MO, USA) for 40 min at 37°C with vortexing every 10 min. The resultant was harvested via centrifugation at 1,200×g for 15 min, and the pellets were resuspended in MEM containing 10% FBS (Gibco). For separation of undigested tissues from the digested cells containing the muscle stem cell population, the digested muscle tissues were centrifuged at 300×g for 5 min, and the supernatant was collected. The supernatant was filtered through a 100-μm cell strainer and harvested via centrifugation at 1,200×g for 15 min. The resulting cells were cultured in the basic growth media (GM) described below or cryopreserved in GM containing 10% dimethyl sulfoxide (DMSO) until used.
The isolated muscle stem cells were cultured on gelatin-coated dishes in basic GM, which consisted of MEM containing 10% (v/v) FBS, 1× glutamax, 1× AA, and 0.1 mM β-mercaptoethanol (All from Gibco) or Skeletal Muscle Cell Growth Medium-2 BulletKit™ (SkGM-2; Lonza, Basel, Switzerland) supplemented with 20 μM SB203580 (Cayman Chemical, Ann Arbor, USA) according to manufacturer’s instructions. Pig muscle stem cells were subcultured every 3 d. When the cells reached approximately 90% confluence, the cultured cells were dissociated using TrypLE™ Express (Gibco). The dissociated cells were transferred onto new gelatin-coated culture dishes at a 1:10 split ratio. The medium was changed every 24 h, and the cells were cultured under humidified conditions in an atmosphere containing 5% CO2 at 37°C.
After three days of subculture, the muscle stem cells at confluence were used for myogenic differentiation. The cells were cultured in a differentiation media consisting of MEM containing 2% (v/v) horse serum (Biowest, Nuaillé, France), 1× glutamax, 1× AA, and 0.1 mM β-mercaptoethanol for 2 d without media changes. After myofiber formation from muscle stem cells, the cells were fixed with 4% paraformaldehyde for further analysis.
Total RNA was extracted using TRIzol reagent (Invitrogen, Carlsbad, USA), and cDNA was synthesized using the High-Capacity RNA-to-cDNA Kit (Applied Biosystems, Foster City, USA). cDNA was amplified using a DyNAmo HS SYBR Green qPCR Kit (Thermo Fisher Scientific, Waltham, USA) containing 1–2 pmol of each primer set listed below in a 10 μL reaction volume: 5’-GTGCCCTCAGTGAGTTCGAT-3’ (forward) and 5’-TCCAGACGGTTCCCTTTGTC-3’ (reverse) for PAX7; 5’-CTGCCCAAGGTGGAAATCCT-3’ (forward) and 5’-GGGGGCCGCTATAATCCATC-3’ (reverse) for MYOD1; 5’-AGTTCGGGGACGAGTTTGAG-3’ (forward) and 5’-TCAAACGCCTGGTTGACCTT-3’ (reverse) for MYF5; 5’-GAGCTGTATGAGACATCCCCC-3’ (forward) and 5’-GTGGACGGGCAGGTAGTTTT-3’ (reverse) for MYOG; 5’-TGCTCCTCCCCGTTCGAC-3’ (forward) and 5’-ATGCGGCCAAATCCGTTC-3’ (reverse) for GAPDH. Amplification and detection were performed using the ABI 7300 Real-Time PCR System (Applied Biosystems) under the following conditions: one cycle at 50°C for 2 min and 95°C for 10 min, followed by 40 cycles of denaturation at 95°C for 15 s and annealing/ extension for 1 min (60°C for MYF5, MYOD1, MYOG, and GAPDH; 58°C for PAX7). The dissociation curves were analyzed, and the amplified products were loaded onto gels to confirm the specificity of the PCR products. Relative expression levels were calculated by normalizing the threshold cycle (Ct) values of each gene to the reference gene, GAPDH, using the delta-delta Ct method.
Cell samples were preincubated for 10 min at 4°C and fixed in 4% (w/v) paraformaldehyde for 30 min. After washing twice with DPBS (Welgene), the samples were treated for 15 min with 0.2% (v/v) Triton X-100 (Sigma-Aldrich) and blocked for 1 h with 10% (v/v) goat serum in DPBS to prevent nonspecific binding. Serum-treated cells were incubated overnight at 4°C with primary antibodies against the myosin heavy chain [MHC (1:200; 05-716, Sigma-Aldrich)]. After incubation with the primary antibody, the cells were treated overnight at 4°C with the appropriate Alexa Fluor-conjugated secondary antibodies. Nuclei were stained with Hoechst 33342 (Molecular Probes, Eugene, OR, USA). Images of stained cells were captured using an inverted fluorescence microscope (Eclipse TE2000-U, Nikon, Konan, Japan).
The data obtained in this study are presented as means±SEM and were analyzed using Prism 6 software (GraphPad Software, San Diego, CA, USA). The significance of differences was determined using two-way analyses of variance followed by Fisher’s least significant difference test. Differences were considered significant at p<0.05 (* p<0.05, ** p<0.01, and *** p<0.001 in Fig).
Results and Discussion
The total number of muscle stem cells per muscle mass having the same weight decreases during postnatal development (Campion et al., 1981), and the proliferation ability of the muscle stem cells is reduced with aging (Chakravarthy et al., 2000). When we compared muscle stem cells from 3-d-old and 25-d-old pigs, the cells from 3-d-old pigs were more numerous and proliferative than cells from the 25-d-old pigs (data not shown). Based on this preliminary observation, the cells were collected from biceps femoris muscles of 3-d-old LYD pigs to establish the in vitro culture conditions to maintain pig muscle stem cells. Because the muscle tissues digested by Pronase contained connective tissues, muscle fibers and quiescent satellite cells, the stem cell population was separated from tissue debris via differential centrifugation and filtration. The isolated muscle stem cells were cultured in MEM-based growth media. Various studies widely used FBS-containing MEM to culture pig muscle stem cells in vitro (Jeong et al., 2013; Miersch et al., 2018). Doumit et al., 1993, showed that pig muscle stem cells had a higher proliferation rate in MEM-based media compared to other media, including McCoy’s 5A, Ham’s F12, DMEM, and DMEM/F12. The high concentration of FBS, which contains several unknown growth factors, supports the proliferation rate and myogenic potential of the pig stem cells during in vitro culture (Doumit and Merkel, 1992). However, pig muscle stem cells gradually lose their proliferation ability and featured morphology during long-term culture over two weeks (Fig. 1A). Quantitative PCR analysis showed that the marker genes for satellite cells and myoblasts (PAX7, MYF5, and MYOD1) (Kuang et al., 2007) and myogenesis-related gene (MYOG) (Cao et al., 2006) were dramatically decreased after passaging (Fig. 1B), which indicates that FBS-containing MEM cannot support the stemness of pig muscle stem cells for an extended period in vitro.
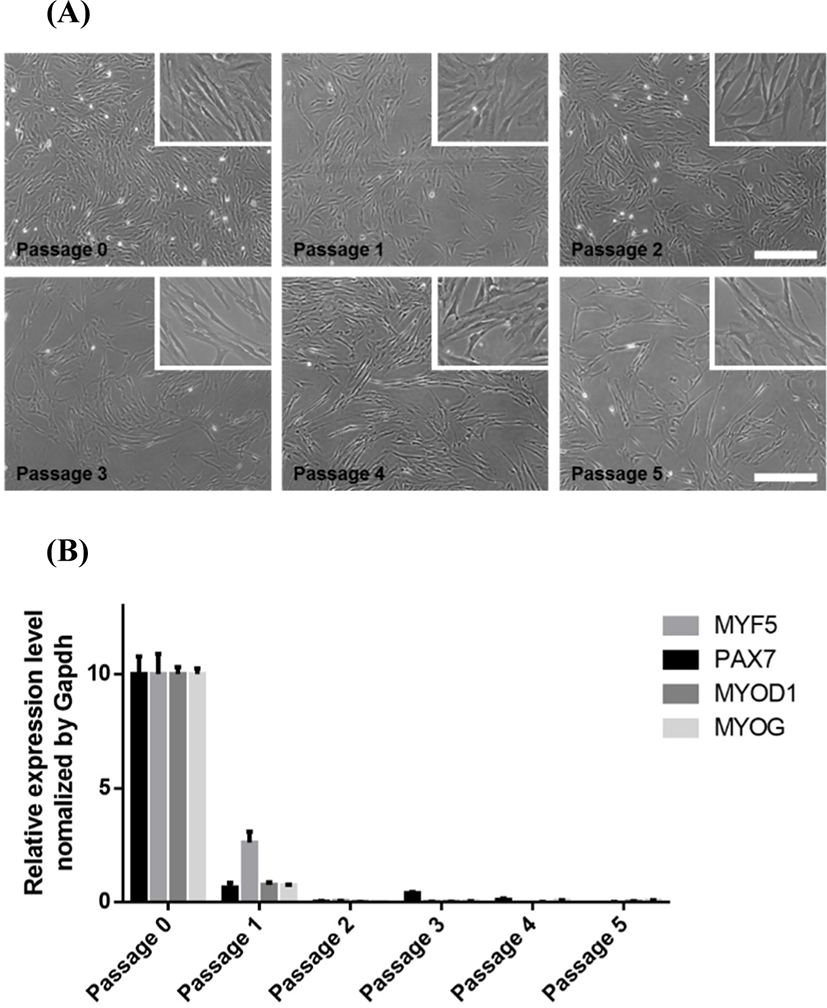
To find suitable in vitro culture conditions for pig muscle stem cells, we first tested the commercially available media SkGM-2, which includes epidermal growth factor (EGF) and dexamethasone, for human myoblasts. EGF and dexamethasone enhance the proliferation and differentiation capacity of myoblasts in vitro (Roe et al., 1995; Syverud et al., 2016). The proliferation rate of pig muscle stem cells cultured in SkGM-2 was highly increased compared to MEM-based growth media (Fig. 2A), and the cells were maintained for an extended period (Fig. 2B). Although the myogenic cell marker genes were significantly increased in the SkGM-2-treated group as measured using qPCR analysis, the genes gradually declined with passaging (Fig. 2C). We next applied SkGM-2 as a basal medium and examined the effect of the p38 inhibitor SB203580 on maintaining the undifferentiated state of pig muscle stem cells. Activation of the p38 signaling pathway inhibits proliferation and causes myogenic differentiation in muscle stem cells (Bernet et al., 2014; Troy et al., 2012). Treatment with SB203580 prevents the downregulation of PAX7 and maintains the stemness of muscle stem cells in vitro (Ding et al., 2018). Although the number of cells was slightly increased, no significant differences were observed between the SB203580 treatment and control groups in morphological features (Fig. 2D). Notably, the myogenic marker genes were significantly upregulated and constantly expressed during long-term culture in the SB203580-treated group (Fig. 2E). The cells cultured in media supplemented with SB203580 were capable of myogenic differentiation after multiple passages, as determined by the immunostaining of MHC (Fig. 3). We verified that these culture conditions also maintained muscle stem cells from other breeds, including Berkshire and Korean traditional pig (data not shown).
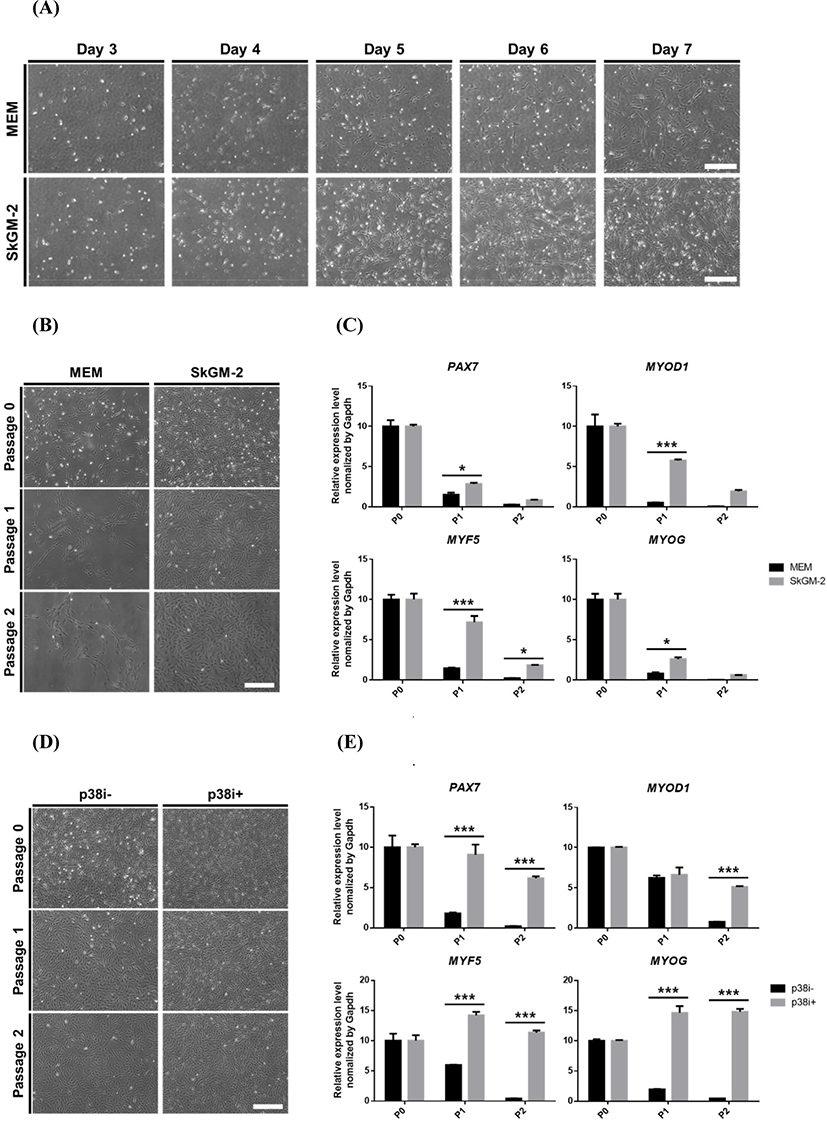
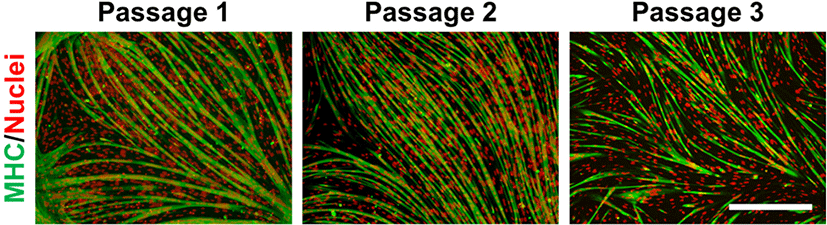
EGF is a well-known mitogen that stimulates the proliferation of pig muscle stem cells cultured in serum-free media (Doumit et al., 1993). EGF enhances the nutrient uptake and protein synthesis of ovine muscle stem cells (Roe et al., 1995). The p38 signaling pathway is involved in the differentiation and aging of muscle stem cells in vivo. Muscle injury upregulates the p38 pathway, which leads to the differentiation of quiescent satellite cells (Troy et al., 2012). The p38 pathway was upregulated in the satellite cells of aged mice, which suppressed the proliferation of satellite cells (Bernet et al., 2014). Dexamethasone is a synthetic glucocorticoid that enhanced the proliferation ability of muscle stem cells via regulation of catabolism (Guerriero and Florini, 1980). Dexamethasone also increased the mitogenic effect of growth factors, such as IGF-1 and IGF-2, on satellite cells (Dodson et al., 1985) and promoted myogenic maturation (Syverud et al., 2016). Similarly, our results demonstrated that these signaling pathways also played pivotal roles in maintaining the stemness of pig muscle stem cells. Accordingly, we found that basal culture conditions containing EGF, dexamethasone, and a p38 inhibitor were suitable for maintaining pig muscle stem cells during an expanded culture in vitro.