Introduction
In recent years, consumers have widely enjoyed fried chicken and sweet and sour pork owing to their high nutritional values, good sensory properties, long shelf lives, and cost effectiveness (Rahimi et al., 2017). Indeed, there is an increasing global demand for deep-fat-fried products due to the palatability, affordability, accessibility, and availability of fried food (Blumenthal, 1991). During the cooking process, food is in contact with hot fat or oil, which is used as the heat-transfer medium, at temperatures above the boiling point of water. Frying is a food-processing operation that involves simultaneous heat and mass transfer. For example, deep-fat-fried savory snacks generally contain 35%–40% oil (Debnath et al., 2009). The frying fat or oil is exposed to atmospheric oxygen and moisture at a high temperature (160°C–180°C) for long times during the frying process (Debnath et al., 2012). Frying oil cannot be used continuously due to the complex series of reactions that take place during frying, thereby resulting in its hydrolysis, oxidation, and polymerization (Benedito et al., 2002). The quality of fried food depends on the quality of oil; therefore, several degradation products of frying oil are harmful to human health as they destroy vitamins, inhibit enzymes, potentially cause mutations, or are gastrointestinal irritation. While low levels of oxidation cause no problems, oil that has been reused beyond its quality limit must not be further used owing to its rancidity; however, establishing uniform usage limits for oils is very difficult. Therefore, in this study, we evaluated the effect of frying time on the oxidative stability of four oils, namely soybean oil (SO), canola oil (CO), palm oil (PO), and pork lard oil (LO), which were reused up to 100 times.
Their acid values (AVs), peroxide values (POVs), thiobarbituric acid reactive substances (TBARS) values, and trans fatty acid contents were measured after each frying cycle. According to the standard regulations, POVs cannot exceed 50 and 60 in the case of fried oil and fried food, respectively. In addition, regulations require AVs not to exceed 2.5 and 5.0 in the case of fried oil and fried food, respectively; however, these regulations only apply to common food and not meat products. Therefore, to provide freshness information for meat product companies and consumers, in this study, linear regression analysis was used to determine how many times SO, LO, CO, and PO can be used to safely fry sweet and sour pork.
Materials and Methods
Sweet and sour pork (Dongwon, Seoul, Korea) was purchased from a major local supermarket. Samples of sweet and sour pork, each weighing 600 g, were prepared and stored in a freezer at −18°C until they were required for various experiments. Commercially available refined, bleached, and deodorized SO (Cheiljedang, Seoul, Korea), LO (Samyang, Seoul, Korea), CO (Cheiljedang, Seoul, Korea), and PO (Ottogi, Seoul, Korea) were used as frying oils. Frying was conducted using a laboratory deep-fat fryer (TF:20, Dong Yang Magic, Seoul, Korea). As the standard method, sweet and sour pork was deep-fat fried in a thermostatically temperature-controlled fryer filled with 2.5 L of refined frying oil. To reach the required temperature, this system was turned on 1 h prior to frying, and the sample was defrosted at 25°C.
After frying for 30 min, each sample was immediately withdrawn from the oil and gently blotted with dry tissue paper for removing the excess surface oil. Before further analysis, each sample was allowed to cool to 25°C. After 1 h, a new sample was fried using the same oil; this process was repeated (without any oil change) 100 times for 10 d (10 times per day). The AV, POV, and TBARS values, and the trans fatty acid contents of each sample were measured.
The AV of the frying oils and fried food were measured according to the AOAC official method. Ethanol:ether (1:2 v/v, 20 mL) was mixed with 1.0 g of the sample oil. AV was determined by titration with aqueous potassium hydroxide (KOH) using phenolphthalein solution as the indicator. AV (mg KOH/g) was calculated as 5.611×(a–b)×f/sample weight (g), where a is the input volume of KOH, b is the blank input volume of KOH, and f is a factor.
The POVs of food and frying oil were measured according to the official method described by the AOAC (2000). Acetic acid:chloroform (3:2, 25 mL) was mixed with 1.0 g of the sample. Saturated potassium iodide solution (70 g of KI in 50 g of water) was added, and the mixture was maintained in the dark for 10 min. After the addition of 30 mL of deionized water, the POV was determined by titration with sodium thiosulfate.
The POV (meq/kg) was calculated as (a–b)×f/sample weight (g)×10, where a is the input volume of sodium thiosulfate, b is the blank input volume of sodium thiosulfate, and f is a factor.
A 10 g sample was homogenized with 25 mL of 20% (w/v) aqueous trichloroacetic acid in 2 M phosphoric acid at room temperature. Homogenization was performed at 14,000×g for 1 min with a homogenizer (HM-150IV, Hanil, Daejeon, Korea). After homogenization, the sample was made up to 20 mL with distilled water, and the supernatant was filtered using Whatman paper (No. 1). A 5-mL aliquot of the filtrate was reacted with 5 mL of 2-TBA (0.005 M in distilled water) in a test tube and stored in the dark for 15 h. The optical density was measured at 530 nm using a spectrophotometer (Libra S22, Biochrom, Cambridge, UK). The results were expressed using the following equation and reported in milligrams of malonaldehyde (MDA) per kilogram; all determinations were performed in triplicate (Witte et al., 1970).
The trans fatty acid contents of the frying oils were measured according to the official Ministry of Food and Drug Safety (MFDS, 2020) method. Gas chromatography was performed using an Agilent model 6890 instrument fitted with a 100 m×0.25 mm×0.2 μm SP-2560 capillary column (Supelco, Bellefonte, PA, USA) and flame ionization detector (FID). The following GC-FID conditions were used: injection volume=1 μL, nitrogen carrier-gas flow rate=1.0 mL/min, and split ratio=50:1 with constant flow control. The injector and detector temperature were set to 250°C and 280°C, respectively. The oven temperature was initially maintained at 180°C for 40 min, and then increased to 230°C at 3°C/min, after which the oven temperature was maintained at 230°C for 10 min.
Frying oils for food (except animal products) have MFDS freshness regulations, which require that AVs and POVs should not exceed 2.5 and 50, respectively. In addition, for fried food, food-freshness regulations require that AVs and POVs should not exceed 5.0 and 60, respectively. Therefore, the available freshness of each frying oil/fried food sample and the maximum number of times the frying oil can be used were estimated by correlating AV and POV data and were calculated as the earliest times at which the quality criteria reached the quality limits stated in the MFDS regulations. Therefore, the time that each quality limit was reached was established as the value of “x” computed by substituting the quality limit “y” in the regression equation that relates the number of frying cycles with representative values of the appropriate factor (Utama et al., 2016).
All analyses were conducted at least three times under each set of experimental conditions. The average value and standard deviation were calculated using Microsoft Excel. Analysis of variance was performed using Statistical Packaging for the Social Sciences for Windows version 10.0. Duncan’s multiple range test (p<0.05) was used to determine differences between means.
Results and Discussion
The AV is commonly specified for fats and oils; it is defined as the weight of KOH (in mg) needed to neutralize the organic acids present in 1 g of fat and is a measure of the free fatty acids present in the fat or oil (Lee et al., 2012; Shin et al., 1990). Control experiment with unused oil provided values of 0.03–0.13, 0.03–0.16, 0.03–0.09, and 0.03–0.19 for SO, LO, CO, and PO, respectively, and no oil exhibited a significant change over the 10 d storage period. The AV of each frying oil was observed to increase significantly with increasing reuse of the oil (reuse number) (Table 1), which is consistent with other report (Yun et al., 2000). The AV was also found to depend on the degree of saturation of the oil, with the highest AV observed for lard. When sweet and sour pork is fried in SO, the AV of the frying oil after a single use was found to be 0.28 mg KOH/g; the AV of the frying oil after 100 cycles of use was 5.41 mg KOH/g (an increase of 5.13), which is above 5.0, the legally allowed maximum for frying oil for non-animal products. When sweet and sour pork was fried in LO, the AV of the frying oil after single use was 0.38 mg KOH/g, which increased to 5.63 mg KOH/g after 100 cycles of use (an increase of 5.25). These results show that frying sweet and sour pork in LO, which has a higher degree of saturation, results in a higher acid value. The AV increased from 0.26 mg KOH/g to 4.80 mg KOH/g over 100 frying cycles for CO, while PO exhibited the smallest increase (1.16 mg KOH/g to 2.61 mg KOH/g over 100 frying cycles). Significant differences were found between SO, LO, CO, and PO (p<0.05). These results are consistent with those of Lee et al. (2013), i.e., AV increases regardless of the type of frying oil. The fried food also exhibited increases in AV during 100 frying cycles, from 0.54 mg KOH/g to 5.72 mg KOH/g in SO, 0.60 mg KOH/g to 6.66 mg KOH/g in LO, 0.56 mg KOH/g to 6.26 mg KOH/g in CO, and 1.96 mg KOH/g to 3.36 mg KOH/g in PO.
The POV is a very important characteristic of lipid quality. The detection of peroxides provides initial evidence of rancidity in an unsaturated fat or oil. While other parameters have been used, the POV is the most widely used parameter; it provides a measure of the extent to which an oil sample has undergone primary oxidation. Control experiments provided POVs of 0.20–1.00, 0.20–1.90, 0.10–0.90, and 0.70–1.40 for unused SO, LO, CO, and PO, respectively, over 10 d of storage. POVs determined during 100 frying cycles are summarized in Table 2. POV was observed to increase as the frying time was increased; hence, the levels of oxidation and rancidity also increased. The POV of SO increased during 70 frying cycles, but it decreased slightly between 70 and 80 cycles and then increased again, with the highest POV of 65.83 observed after 100 cycles. The highest POV for LO (70.20) was observed after 100 frying cycles, and similarly CO and PO also exhibited their highest POVs after 100 cycles, i.e., 62.50 and 14.70, respectively. The POV did not increase regularly in the early stages, which can be attributed to an induction period that rapidly shortens with increasing oxidation temperature, leading to instantaneous peroxide decomposition and formation of secondary compounds, such as alcohols, carboxylic acids, and carbonyl compounds; consequently, a low POV is observed. Nevertheless, the POV had increased significantly at the end of 100 frying cycles. Therefore, after 100 frying cycles, the POVs of all the frying oils used in this study had increased, which is consistent with the results of other studies (Kim et al., 1990; Shin and Kim, 1985). Therefore, since the POVs follow the order: LO>SO>CO>PO, SO, CO, and LO are more likely to become rancid during frying than PO. Palm oil contains significant amounts of saturated fatty acids and palmitic acid; therefore, it is used as a solid oil at 25°C. The reason that PO exhibits the lowest POV is that it is oxidized slowly, which reduces the production of volatile carbonyl compounds through oxidation upon heating (Han et al., 1991). In contrast, vegetable fats and oils have high unsaturated fatty acid contents. Hence, they become rancid quickly, which can be attributed to the high degree of unsaturation that leads to the formation of large amounts of free radicals that produce hydroperoxides. These hydroperoxides decompose to form aldehydes and ketones that tarnish the flavor of the oil. In addition, organic acids are produced by the thermal decomposition of double bonds and the hydrolysis of esters; hence, rancidity increases strongly (Lee et al., 2004). These results are consistent with those of Lee et al. (2000) who showed that the POV of SO increased more than those of other frying oils. In addition, this mechanism is complicated and depends strongly on the type of frying oil and food. The POVs for fried food show similar results and trends as the frying oils. After frying only once, the POV of sweet and sour pork fried in SO was 6.80 meq/kg, whereas it was 7.43 meq/kg in LO, 7.49 meq/kg in CO, and 7.36 meq/kg in PO. After frying 100 times, POVs of 78.40 meq/kg, 82.60 meq/kg, 78.90 meq/kg and 16.83 meq/kg were observed for SO, LO, CO, and PO, respectively. In the case of PO, the POV after one frying cycle was measured to be 7.36 meq/kg, and it increased continuously with increasing storage and frying cycles. A POV of 16.83 meq/kg was observed after frying 100 times in PO, indicating that the formation of peroxides and decomposition occur more slowly in PO than in the other frying oils. This observation is ascribable to the high saturated fatty acid content of PO that endows it with excellent characteristics in terms of oxidation and thermal safety, as discussed above.
TBARS values of 0.077–0.283, 0.085–0.333, 0.078–0.263, and 0.055–0.299 were determined for unused SO, LO, CO, and PO, respectively, after 10 d of storage. TBARS values determined during frying are presented in Table 3. The TBARS values of the frying oils were observed to increase or decrease at various time intervals when frying sweet and sour pork. After 100 frying cycles, the TBARS values of the frying oils had increased significantly, with values of 3.142 MDA mg/kg, 3.454 MDA mg/kg, 2.187 MDA mg/kg, and 7.924 MDA determined for SO, LO, CO, and PO, respectively (Table 3). A similar result was observed for the fried food, with TBARS values of 2.461 MDA mg/kg, 3.351 MDA mg/kg, 2.738 MDA mg/kg, and 1.510 MDA mg/kg determined after frying 100-times in SO, LO, CA, and PO, respectively. However, because the changes in the TBARS values during frying were irregular and that they correlated poorly with other factors, TBARS values were not used as a shelf-live reuse prediction indicator.
Trans fatty acid (tFA) contents during frying are presented in Table 4. The trans fatty acid contents of soybean, lard, canola, and palm oils used for frying sweet and sour pork were measured. The tFA contents, as proportions of the of total fat (as g/100 g fatty acid), were found to be 0.70, 0.58, 0.69, and 0.51 in the singly used SO, LO, CO, and PO, respectively. After 100 frying cycles and 10 d, SO, LO, CO, and PO exhibited tFA contents (g/100 g) of 0.85, 1.13, 0.86, and 0.61, respectively. These results are consistent with those of Kim et al. (2006) who showed that trans fatty acid content increases during the frying process. Therefore, the tFA levels increased significantly during the reuse of frying oil, with LO exhibiting the highest tFA content after 100 frying cycles (Bochicchio et al., 2005; Indrasti et al., 2010). These results suggest that oxidative polymerization caused by the production of free fatty acids, formation of carbonyl compounds, and polymerization during high temperature heating occur during frying, i.e., the tFA content increases with the number of frying cycles and frying temperature. In addition, as the trans fatty acids in the sweet and sour pork were released at the beginning of frying, the tFA content appears to be high (Kim et al., 2006).
Frytime/day | Frying oil (g/100 g) | |||
---|---|---|---|---|
Soybean oil | Lard | Canola oil | Palm oil | |
1 t/0 d | 0.70 | 0.58 | 0.69 | 0.51 |
100 t/10 d | 0.85 | 1.13 | 0.86 | 0.61 |
To analyze the relationships between AV and POV, we subjected the data obtained in the current study to linear regression analysis (Fig. 1). An r2 value of 0.9301 was observed for SO, while values of 0.9191, 0.8014, and 0.9301 were determined for LO, CO, and PO, respectively. Clearly, the formation of oxidation products is strongly related to changes in POV and AV (r2=0.8014–0.9301). These results agree with those of Kim (2008) and Lee and Park (2010), revealing that AV and POV are highly correlated. In contrast, the relationship between the TBARS value and oxidation is less correlated than those of the other two oil-oxidation parameters (r2=0.5141–0.841). We next subjected the data in Tables 1 and 2 to linear regression analysis to determine the maximum reusability of each oil, the results of which are summarized in Tables 5 and 6. Using AV as the quality criterion, SO can be used 37 times before the quality threshold for oil is reached (r2>0.9389), whereas LO, CO, and PO can be used 32, 58, and 87 times, respectively (Table 5). Using POV as the quality criterion, SO, LO, CO, and PO can be used 69, 68, 77, and 450 times, respectively. According to the MFDS, regulations require frying oils for common foods to have AVs of less than 2.5 mg KOH/g and POVs less than 50 meq/kg; however, there are no specific regulations for oils used to fry animal-based foods and the corresponding fried foods. The analogous results for fried sweet and sour pork are shown Table 6. Using AV as the quality criterion, SO can be used to fry sweet and sour pork 82 times before the quality criterion is reached (r2=0.9), while LO, CO, and PO can be used 79, 82, and 286 times. Using POV as the quality criterion, SO, LO, CO, and PO can be used 78, 78, 81, and 672 times, respectively. Regulations permit common fried foods to have AVs below 5.0 mg KOH/g and POVs below 60 meq/kg; however, there are no specific regulations for edible oils used to fry animal-based foods such as pork, sweet and sour pork, pork cutlets, and their corresponding fried foods. Because animal-based foods contain significant amounts of protein and fat, they should be held to different standards than ordinary food. Hence, based on our results, we suggest establishing regulations for frying oils for animal-based foods and their corresponding fried foods, and our data may be useful for determining these food-quality regulations.
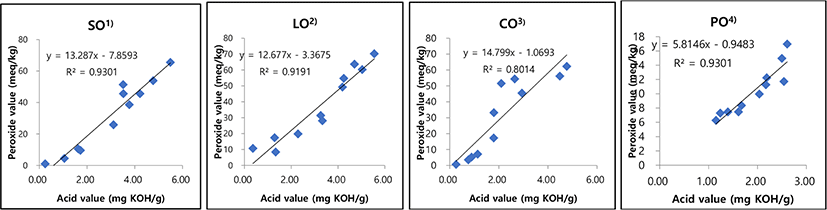