Introduction
In the modern food industry, much effort to meet consumer demand for well-being trends has been made by replacing artificial food additives with natural ingredients. This trend has now evolved into the concept of “clean label products” that are formulated with more natural ingredients and less processed (Aschemann-Witzel et al., 2019). To manufacture “clean label products”, multifunctional food additives have been considered extensively to minimize the use of artificial food additives.
Gelatin, which is obtained through the thermal hydrolysis of collagen in animal tissues, has recently been used to produce bioactive hydrolysates or peptides (Lafarga et al., 2017; Sarbon et al., 2018). Previous studies primarily used mammalian, poultry, and fish skin as gelatin sources, and several physiological benefits of gelatin hydrolysate, including antimicrobial, antioxidant, or antihypertensive activities have been found through in vitro and in vivo assays (Gómez-Guillén et al., 2011). Moreover, gelatin hydrolysates with antioxidative and/or antimicrobial activities could be used to extend the shelf stability of processed foods, resulting from the retardation of oxidative quality change and the inhibition of harmful microbial growth, respectively (Nikoo et al., 2015; Zhang et al., 2020).
In commercial restructured and/or emulsified meat products, gelatin (generally from 0.5 to 3.0 g/100 g) is used to improve water-holding capacity and textural properties (Lee and Chin, 2016). Moreover, it has been well documented that the positive functionality of gelatin in processed meat products results from mainly hydration properties and gel-forming ability of gelatin (Gómez-Guillén et al., 2011). Beyond the positive functionality, however, there is no available literature on the impact of gelatin on the oxidative stability of processed meat products during storage.
Commercial gelatin products used in the meat industry is mostly produced from pork skin and bovine hide (Karim and Bhat, 2009), but gelatin extraction materials have been diversified now into poultry and fishery sources due to the risk of infectious diseases and religious reasons (Tümerkan et al., 2019). In particular, poultry by-products such as feet and skins have been considered as promising materials for gelatin extraction (Noh et al., 2019; Park et al., 2013). However, although some previous studies have found the techno-functional advantages of duck skin gelatin in processed meat products (Kim et al., 2020), the efficiencies of pork and duck skin gelatin on the quality attributes of processed meat products have not been clearly compared.
In this preliminary study, the pork and duck skin gelatin hydrolysates were prepared through an enzymatic hydrolysis method that produced gelatin hydrolysates with antioxidant capacity (Lee et al., 2012), and the impacts of gelatin and its hydrolysate on technological properties and lipid oxidation stability of cooked sausage have been investigated.
Materials and Methods
Commercial pork skin gelatin (gel strength: 280 bloom, particle size: 5–15 mesh, Hangzhou Qunli Gelatin Chemical, Hangzhou, China) was purchased from a local market, and frozen duck skin used for gelatin extraction was also provided by a duck processing company (Farm Duck, Jeongeup, Korea). Pork ham and back fat were purchased from a local market after 48 h postmortem.
Duck skin gelatin was prepared according to a previously described extraction procedure (Kim et al., 2016; Tümerkan et al., 2019) with minor modifications. The frozen duck skin was thawed in a 4°C refrigerator for 24 h. The excessive subcutaneous fat was removed, and the duck skin was washed with tap water several times. The washed duck skin was ground using a meat grinder (MN-22S, Hankook Fugee Industries, Hwaseong, Korea) equipped with an 8-mm plate. The ground duck skin was soaked in 1.5 volumes of 0.1 M NaOH for 16 h to remove non-collagenous proteins and neutralized with tap water (approximately pH 7.0). Acidic soaking of the duck skin was performed with 2 volumes of 0.5 M acetic acid for 12 h, and the swelled duck skin was neutralized again using tap water to pH 6.5–7.0. For hot-water extraction, the duck skin was blended with distilled water (1:1 ratio, w/w) and heated in a 65°C water bath for 3 h. After heating, the sample was filtrated through cheesecloth, and the gelatin solution was set in a 4°C refrigerator for 12 h. The upper fat layer was removed manually, and the gelatin layer was freeze-dried and pulverized for producing duck skin gelatin powder.
Gelatin hydrolysates were prepared through stepwise enzymatic hydrolysis using collagenase and pepsin, as described by Lee et al. (2012) with minor modifications. Six grams of pork or duck skin gelatin powder were dissolved in 540 mL of distilled water and gently homogenized, and a low concentration of gelatin solution was used to prevent gelation and coagulation. The gelatin solution was heated at 80°C for 10 min to inactivate any enzymes contained in the gelatin powders and cooled at room temperature for 2 h. The pH of the gelatin solution was adjusted to pH 7.0 using 1 M NaOH and finally diluted to 600 mL in a volumetric flask to form 1% gelatin concentration (w/v). The first enzymatic proteolysis of gelatin was performed with collagenase (EC3.4.24.3) at a 1:100 ratio (w/w), and the enzymatic reaction was placed in a 37°C incubator for 12 h with stirring at 250 rpm. The mixture was heated at 80°C for 10 min and cooled to inactivate the collagenase. The pH of the mixture was adjusted to pH 2.0 using 6 N HCl for the second enzymatic hydrolysis, and the mixture was treated with pepsin (EC3.4.23.1) at a 1:50 ratio (w/w). The second step was conducted under the same hydrolysis condition as above, and the product was heated at 80°C for 10 min and cooled to inactivate the pepsin. The pH of gelatin hydrolysates was finally adjusted to pH 5.7 using 1 M NaOH and then centrifuged at 20,000×g for 10 min (4°C). The supernatant was freeze-dried and pulverized to obtain gelatin hydrolysate powder.
The excessive subcutaneous fat and connective tissues on the surface were removed. The pork ham and back fat were ground using a meat grinder with an 8 mm plate (MN-22S, Hankook Fugee Industries, Hwaseong, Korea). The ground pork, ground back fat, ice, and other ingredients were emulsified using a bowl cutter (Cutter C4W, Sirman, Marsango, Italy). All treatments were formulated with 60% (w/w) ground pork, 20% (w/w) ground pork back fat, and 20% (w/w) ice. Based on the total sample weight, 1.5% (w/w) NaCl, 0.3% (w/w) sodium tripolyphosphate, and 1% (w/w) gelatin or gelatin hydrolysates were added as follows: control (without gelatin), 1% (w/w) pork skin gelatin, 1% (w/w) pork skin gelatin hydrolysate, 1% (w/w) duck skin gelatin, and 1% (w/w) duck skin gelatin hydrolysate, respectively. The emulsified meat batter was stuffed into a collagen casing (diameter of 25 mm, #240, NIPPI, Tokyo, Japan), cooked in an 80°C water bath until the core temperature reached 75°C, and then, cooled in ice-water. The cooked sausages were placed at room temperature for 3 h to evaporate surface moisture. To determine lipid oxidation stability, the cooked sausages were vacuum-packaged and stored in a 4°C refrigerator for 4 wk. A total of three independent batches was prepared.
The pH of the gelatin and hydrolysate powder (1% solution, w/v) was measured three times using an electronic pH-meter (Orion Star™ A211 pH Benchtop Meter, Thermo Scientific, USA). For meat batter and cooked sausage, the homogenate, which was prepared with 3 g of sample and 27 mL of distilled water, was used for pH analysis.
The moisture content of the cooked sausage was determined using the oven air-drying method (AOAC, 2007). The protein content of gelatin powder and cooked sausage was measured using a nitrogen protein analyzer (Rapid N Cube, Elementar, Langenselbold, Germany) and calculated using nitrogen-protein conversion factors of 5.55 and 6.25, respectively (Mariotti, 2008).
The color characteristics of gelatin powder and cooked sausage were measured using a colorimeter (CR-400, Konica Minolta Sensing, Osaka, Japan) with an 8 mm aperture and 2° observer. The setting for the illuminant was a D65 source. Calibration of the instrument was conducted with a calibration tile (CIE L*: +93.01, CIE a*: –0.25, CIE b*: +3.50), according to the manufacturer’s manual. The CIE L*, a*, and b* values were taken five times on the cross section of each sample (internal color).
The protein solubility of the meat batter was measured using the method described by Warner et al. (1997). Two grams of sample were homogenized with a buffer solution (1.1 M potassium iodide in 0.1 M potassium phosphate buffer, pH 7.2) using a homogenizer (HG-15A, Daihan Sci., Seoul, Korea) at 12,000 rpm for 2 min. The homogenate was stored in a 2°C refrigerator overnight and centrifuged at 1,500×g for 20 min (4°C). The supernatant was filtered through a filter paper (Whatman no. 1), and the protein concentration of the filtrate was quantified using the Biuret method (Gornall et al., 1949). Protein solubility was expressed in mg protein soluble fraction per gram of emulsified meat batter (mg/g).
The cooking loss of cooked sausage was determined by the percent weight difference between the raw and cooked samples (Kim et al., 2015).
Texture profile analysis of cooked sausage was conducted using a texture analyzer (CT3, Brookfield Engineering Laboratories, Middleboro, MA, USA). The sausages were equilibrated to room temperature (22°C) for 3 h, and four samples (2.5 cm height) were prepared from the middle portion of each sample. A twice compression cycle test (70% compression of the original sample height) was performed with a cylinder probe (diameter in 4 cm). Sample deformation curves were obtained with a 50 kg maximum load cell, and the analysis condition was as follows: pre-test speed 1.0 mm/s, post-test speed 5.0 mm/s, and head speed 2.0 mm/s. The values for hardness (kg), springiness (ratio), cohesiveness, gumminess (kg), and chewiness (kg) were presented (Bourne, 1978).
Lipid oxidation of the cooked sausage was determined weekly using the 2-thiobarbituric acid reactive substance (TBARS) method of Buege and Aust (1978). The results were expressed as malondialdehyde mg/kg sample per kg of sample (mg MDA/kg sample).
The experimental design was a completely randomized block design with three independent batches. An analysis of variance (ANOVA) was performed on the measured variables using the one-way ANOVA procedure of the SPSS program (SPSS Inc., Chicago, IL, USA). Duncan’s multiple range test was used to determine significant differences between means (p<0.05). For lipid oxidation, a two-way ANOVA was conducted, in which treatment and storage effect as the main effects, and their interaction were found.
Results and Discussion
The protein content, pH, and color characteristics of pork and duck skin gelatin and their hydrolysates are shown in Table 1. The protein content of pork skin gelatin was 93.54 g/100 g, whereas duck skin gelatin showed a considerably lower protein content (78.71 g/100 g) (p<0.05). This result was likely that the commercial pork skin gelatin product was industrially purified through filtration and deionization processes. After stepwise enzymatic hydrolysis using collagenase and pepsin, the hydrolysates of pork and duck skin gelatins showed similar protein contents of 76.77 and 72.17 g/100 g, respectively (p>0.05). Previously, Kim et al. (2013) reported that the protein content of pork skin gelatin hydrolysate prepared through 0.3% flavourzyme treatment was 511.53 mg/g dry weight.
The pH value (pH 4.50) of pork skin gelatin was significantly lower than that of duck skin gelatin (pH 6.17); however, their hydrolysates showed similar pH values (p>0.05) since the final pH of gelatin hydrolysates were equally adjusted at the end of enzymatic hydrolysis process. According to a previous study, the final pH of gelatin is mainly affected by the acid/alkali treatment and neutralization in the gelatin extraction process (Kim et al., 2012), and the general pH after neutralization is about 5.0–7.0 (Noh et al., 2019). Thus, in this study, the pH difference between pork and duck skin gelatin could be associated with different manufacturing processes.
In terms of color characteristics, duck skin gelatin showed higher CIE L* but lower CIE b* (yellowness) than pork skin gelatin (p<0.05); there was no significant difference in redness between the two different gelatin sources. Stepwise enzymatic hydrolysis decreased lightness of both gelatin sources but increased redness (p<0.05). After enzymatic hydrolysis, the yellowness of duck skin gelatin significantly increased, but no change in yellowness was observed between pork skin gelatin and its hydrolysate. Between pork and duck skin gelatin hydrolysates, similar lightness and redness were observed, but duck skin gelatin hydrolysate showed a slightly higher yellowness than pork skin gelatin hydrolysate (p<0.05).
Previously, Chuaychan et al. (2017) suggested that an increase in temperature during the drying process could increase the yellowness of gelatin hydrolysate powder, resulting from a Maillard reaction between reducing sugars and amino acids. In this study, since the duck skin gelatin hydrolysate powder was prepared through a lyophilization process, the increased yellowness was probably due to the heating process (80°C) for the inactivation of proteases during enzymatic proteolysis. In general, commercial gelatin generally presents a white color; however, the color characteristics of gelatin are not related to functional properties such as gel-forming ability, gel strength, and emulsifying capacity (Ockerman and Hansen, 1988). Therefore, the increased yellowness of gelatin hydrolysates may have little to no impact on the functional properties of gelatin hydrolysates.
The chemical composition and color characteristics of cooked sausages prepared with gelatin or gelatin hydrolysates are shown in Table 2. The pH values of meat batter and cooked sausages were unaffected by the incorporation of gelatin or gelatin hydrolysate (p=0.174). Although there was significant difference in pH between pork and duck skin gelatin and gelatin hydrolysates (Table 1), it seemed that the inclusion level might be too small to change the pH of meat batter and cooked sausages. The addition of gelatin and gelatin hydrolysates had no significant effect on the moisture and protein content of the cooked sausage. The moisture content of the control and all treatments ranged from 61.19 to 62.41 g/100 g, and the protein content was from 16.36 to 19.89 g/100 g. As a similar result, it has been reported that the addition of 1% gelatin slightly increased the protein content of cooked sausage, whereas the moisture content was relatively decreased (Lee and Chin, 2016).
1) Gelatin hydrolysates were prepared through a stepwise enzymatic hydrolysis using collagenase and pepsin.
There was no change in the lightness and yellowness of the cooked sausages when 1% gelatin or gelatin hydrolysate were added (p>0.05). While the addition of gelatin and gelatin hydrolysate decreased the redness of the cooked sausage (p<0.05); however, the difference between the control and treatment was numerically too small. As a similar result, Lee and Chin (2016) reported that the addition of 0.5%, 1.0%, and 1.5% pork skin gelatin had no impacts on lightness, redness, and yellowness of pork sausages. Our results indicate that the color changes of gelatin hydrolysate due to enzymatic hydrolysis may have no impacts on color characteristics of cooked sausages and suggest that this phenomenon could be affected by the inclusion level of gelatin and gelatin hydrolysate.
The addition of 1% gelatin and gelatin hydrolysate caused an increasing trend in protein solubility of the meat batters (p=0.080; Table 3). This was probably related to the increased protein content with adding gelatin or gelatin hydrolysates. In terms of cooking loss, a decreasing trend was found due to the addition of 1% gelatin and gelatin hydrolysates (p=0.081). Previously, Lee and Chin (2016) reported that the addition of 1% pork skin gelatin had little effect on the water loss of cooked sausage. Recently, Noh et al. (2019) reported that the positive impacts of gelatin on the water-holding capacity of processed meat products could be associated with the functional properties rather than the interaction between myofibrillar proteins and gelatin added. Thus, the inclusion level of 1% gelatin or gelatin hydrolysate might be insufficient to form a gel matrix to entrap moisture in the meat batter.
1) Gelatin hydrolysates were prepared through a stepwise enzymatic hydrolysis using collagenase and pepsin.
The addition of 1% gelatin and gelatin hydrolysate did not affect hardness, springiness, and gumminess of cooked sausage (p>0.05; Table 3). However, the addition of duck skin gelatin hydrolysate significantly increased the cohesiveness of cooked sausage. As a result, higher chewiness was observed for gelatin or gelatin hydrolysate treatments compared to the control (p<0.05). Previously, the impacts of gelatin on textural properties of cooked sausages have been inconsistent. Lee and Chin (2016) reported that the hardness, cohesiveness, gumminess, and chewiness of emulsion sausages decreased as the gelatin level increased. In addition, they reported that gelatin addition did not affect the springiness of emulsion sausage, since only a weak interaction between muscle protein and gelatin could occur (Lee and Chin, 2016). On the other hands, Jridi et al. (2015) indicated that fish gelatin addition (0%–1.5%) increased the hardness of turkey sausage but decreased cohesiveness. However, our results show that the addition of duck skin gelatin hydrolysate could increase the cohesiveness of cooked sausage. This is probably because the peptide groups in gelatin hydrolysate, which are available for the protein-protein interaction with muscle protein, could be exposed additionally. Moreover, since there are differences in amino acid composition and molecular size of gelatin, such positive effects of gelatin hydrolysate may be also different depending on gelatin extraction source.
The effect of gelatin and gelatin hydrolysate addition on the lipid oxidation of cooked sausage during 4 wk of refrigerated storage is shown in Fig. 1. At the initial storage time (0 wk), the incorporation of duck skin gelatin or hydrolysate significantly increased the TBARS value of cooked sausages. Throughout the refrigerated storage period, the lipid oxidation of cooked sausages prepared with duck skin gelatin or hydrolysate accelerated consistently (p<0.05). As a result, the TBARS value of cooked sausage prepared with duck skin gelatin at 2 wk reached 1 mg MDA/kg sample, which is recognized as a limit of sensorial acceptance. For duck skin gelatin hydrolysate, the phenomenon of rapid lipid oxidation was alleviated slightly (0.88 mg MDA/kg at 4 wk). No changes in the TBARS value of the pork and duck skin gelatin hydrolysate treatments were observed during 4 wk of the refrigerated storage period (p>0.05). The pork skin gelatin hydrolysate treatment presented similar TBARS value to the control during overall storage period (p>0.05). According to Ch’ng et al. (2014), when 0.5% of commercial gelatin, cold water fish skin gelatin, and bovine gelatin was added to chicken sausages, rapid lipid oxidation occurred during 3 wk of refrigerated storage. Thus, fatty acids and/or other pro-oxidants contained in raw skin materials for gelatin extraction could be incorporated into gelatin powder, which might be associated with the accelerated lipid oxidation of meat products formulated with gelatin.
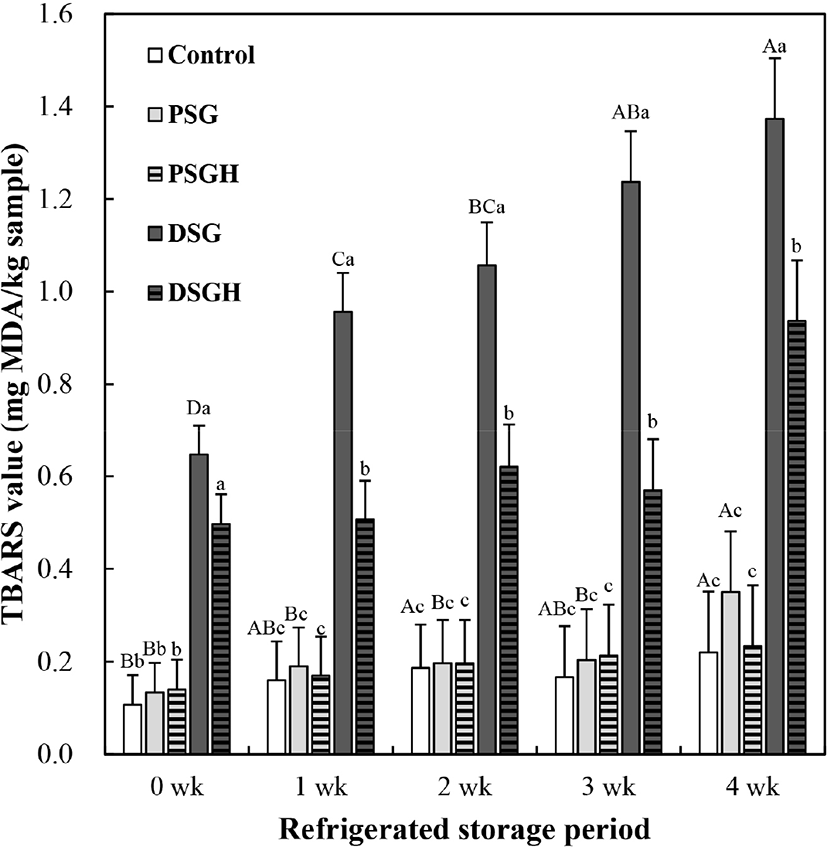
Conclusion
In conclusion, this study shows comparable effects of gelatin and gelatin hydrolysate addition on the technological properties of cooked sausages. In particular, the addition of 1% gelatin hydrolysate could increase the cohesiveness and chewiness of cooked sausages. However, duck skin gelatin considerably accelerated the lipid oxidation of cooked sausages during 4 wk of the refrigerated storage period, although duck skin gelatin hydrolysate prepared through the stepwise enzymatic hydrolysis using collagenase and pepsin could alleviate the accelerated lipid oxidation. In further studies, an extraction process that can minimize the incorporation of lipid and/or pro-oxidant compounds should be considered for developing a multifunctional gelatin hydrolysate that provides antioxidant capacity as well as technological benefits.