Introduction
Sour cream, also known as fermented cream, is a traditional dairy product that is consumed in several countries and has various applications. Sour cream is very popular in North America, Mexico, and Northern and Eastern Europe; it is commonly added to stews and other meat dishes, or is used as a topping for fish, vegetables, salads, and some cakes. It can also be employed in the baking process for cakes, cookies, biscuits, and scones (Champagne and Côté, 1987; Goddik, 2012).
In addition to its unique flavor, sour cream possesses high nutritional value because it contains milk-derived proteins and fats. Sour butter, which is made by subjecting sour cream to various processes, including churning, is popular despite its short shelf life. Sour cream is categorized as follows by its fat content and in accordance with the specifications of the United States Department of Agriculture (USDA): sour cream, fat ≥18%; light sour cream, fat ≤9%; low-fat sour cream, fat ≤6%; and non-fat sour cream, fat ≤1% (Champagne and Côté, 1987; Narvhus et al., 2019; USDA, 2000).
Sour cream has a sour but soft taste, with a tinge of sweet and savory flavors and is slightly viscous. One of the components of sour cream that exerts a strong influence on its flavor is diacetyl. Diacetyl is one of the major compounds that is generated when milk-based ingredients are subjected to lactic acid bacteria (LAB) fermentation, and which produces a decisive effect on the quality of fermented products and their acceptance by consumers (Monnet et al., 2000; Moyane and Jideani, 2013; Rincon-Delgadillo et al., 2012; Shepard et al., 2013). Aromatic compounds generated during fermentation are typically comprised of volatile organic acids and carbonyl compounds including diacetyl, which is a volatile carbonyl compound. In LAB, diacetyl is generated via the citrate fermentation pathway. Citrate, which is a precursor in this pathway, contributes to the stability of diacetyl that accumulates after fermentation (Dorau et al., 2019; Ji et al., 2015; Kaneko et al., 1990). Therefore, citrate-utilizing LAB, such as Lactobacillus, Lactococcus lactis subsp. lactis, and Leuconostoc sp., are frequently employed in the manufacture of sour cream (Bassit et al., 1995; Boumerdassi et al., 1996; Khemariya et al., 2017; Maurad and Meriem, 2008).
Increasing interest in foods that improve health and well-being life have spawned an interest in ‘probiotics’ and ‘fermentation’ worldwide (Asghar et al., 2017). Probiotics refer to living microorganisms that provide health benefits by improving the balance of gut microbes in the host. Currently, well-known probiotic bacterial strains include the genus Bifidobacterium, the genus Lactobacillus, the genus Lactococcus, the genus Enterococcus, Clostridium butyricum, and Bacillus polyfermenticus. Probiotics have been reported to produce beneficial effects, such as improving gut health, immune modulation, antibacterial and antiviral effects (Gill et al., 2000; Lim et al., 2018). Therefore, there are growing expectations regarding the nutritional value and health functional effects of sour cream that has been fermented using probiotic LAB.
In comparison with Europe, one of the greatest challenges for sour cream manufacturing technology in Korea is the failure to satisfy consumers’ demands due to insufficient low sensory profiles on account of low diacetyl concentrations. Diacetyl rapidly increases during the fermentation of cream and the fermented flavor becomes weaker as the diacetyl is converted to acetoin. There is additional loss of diacetyl during post-fermentation processes such as drying. To provide an excellent fermented flavor, it is essential to use LAB producing a high concentration of diacetyl. It is therefore important to establish fermentation conditions that allow LAB to produce diacetyl optimally.
In the present study, it was aimed to isolate LAB with probiotic activity, with excellent fermentation ability for cream, and optimal sensory properties in the manufacture of sour cream. In addition, by measuring the concentration of diacetyl produced while varying the LAB culture conditions, optimal conditions for diacetyl production were aimed to be established.
Materials and Methods
To isolate bacterial strains, unsterilized raw milk that had been milked within the last 2 days at the Lotte Foods Pasteur Factory (Hoengseong, Korea) was collected. After performing serial dilutions with sterilized saline, 0.1 mL samples of the diluted milk were obtained. The diluted milk was spread onto solid MRS medium containing 0.002% (w/w) of bromocresol purple (BCP) and 1.5% (w/w) of agar. After cultivation for 48 h in an incubator at a constant temperature of 37°C, colonies that displayed a yellow ring were isolated.
The isolated strains were inoculated onto MRS agar and activated for 24 h at 30°C, and then inoculated into 10 mL of MRS broth (Difco, Becton, NJ, USA) to produce the seed culture. The seed culture broth was inoculated at a concentration of 1% into MRS broth containing 1 g/L of citrate. The broth was incubated for 24 h at 30°C (Bassit et al., 1995; Hassan et al., 2017), and centrifuged (10,000×g, 10 min). The concentrations of α-acetolactate and diacetyl in the supernatant were measured using a gas chromatography-electron capture detector (GC-ECD, Agilent, Santa Clara, CA, USA). Lactobacillus casei ATCC393 (hereafter, referred to as LC393) obtained from the American Type Culture Collection (ATCC, Manassas, VA, USA) (Hegazi and Abo-Elnaga, 1980) was used as a control to compare diacetyl production.
The API 50 CHL kit (Biomerieux, La Balme-les-Grottes, France) was used to measure sugar utilization and rapid identification of the isolated bacterial strains. The API 50 CHL kit was inoculated with cultured colonies on MRS agar, and the variation of color (yellow) in each was measured after culturing for 24 and 48 h at 37°C, respectively. The results were used to identify bacterial strains using Biomerieux DB (https://apiweb.biomerieux.com).
In addition, genetic identification was performed by analyzing 16s rDNA. After extracting genomic DNA using a genomic DNA preparation kit (Promega, Madison, WI, USA), PCR was performed with the universal primers 27F (5’-AGA GTT TGA TCC TGG CTC AG-3’) and 1492R (5’-TAC GGY TAC CTT GTT ACG ACT T-3’) to amplify the 16s rDNA gene (Lim et al., 2018). The PCR products were purified using the QIA quick PCR kit (QIAGEN, USA), nucleotide sequencing was outsourced to Macrogen (Seoul, Korea), and the sequences were compared with a DB using BLAST at GenBank on the NCBI website (https://blast.ncbi.nlm.nih.gov/Blast.cgi).
To optimize the cultivation temperature for diacetyl production, seed culture for the isolated strain or control strain (LC393) was inoculated into 100 mL of MRS broth containing 0.1% citrate and incubated at 10°C, 20°C, 25°C, 30°C, or 37°C. To identify the optimal citrate concentration, 0%, 0.1%, 0.2%, 0.3%, 0.5%, 1%, 2%, or 3% citrate was added to the MRS broth, and the diacetyl concentration was measured after cultivation for 15 h at 20°C. To investigate the effects of metal ions, 0.01% of iron (Fe2+), magnesium (Mg2+), manganese (Mn2+), or calcium (Ca2+) was added to the culture broth. The concentrations of metal ion were treated at 0.0001%, 0.001%, 0.01%, 0.1%, or 1% for highest diacetyl production. The optimal cultivation time was investigated based on the optimal temperature and citrate concentration identified above.
In each condition, culture broth was collected after cultivation and centrifuged (12,000×g, 10 min), and GC-ECD (Agilent 7890A with Electron Capture Detector) was used to measure the diacetyl concentration. To enumerate viable cells of isolated strain, some samples were serially diluted and spread on MRS agar and incubated.
After activating the isolated strain in MRS agar, 2–3 colonies were inoculated into 30 mL of MRS broth and cultured for 24 h at 37°C. This seed culture broth was then centrifuged (8,000×g, 10 min), the supernatant was removed, and 10 mL of 0.1 M phosphate buffer (pH 6.8) was added to suspend the colonies. The same step was repeated thrice; after removing the final supernatant, 5 mL of 0.1 M phosphate buffer was added to suspend the bacterial colonies to generate the final seed culture for sour cream manufacture. The commercially whipped creams, a product from Lotte Foods, was purchased and used (Pasteur Fresh Cream, Lotte Foods, Korea), 0.2 g of citrate, and 1 mL of seed culture broth were added to 78.8 g of cream and 20 g of skim milk, and the mixture was incubated for 15 h at 20°C. After cultivation, 1 g of cream was collected and serially diluted using sterile saline, 100 μL of the appropriate dilution was collected and spread onto MRS agar for measuring the viable cell count. The diacetyl concentration was analyzed using GC-ECD. In addition, an electronic tongue (Intelligent Sensor Technology, SA402B, Japan) and GC/MS (Agilent Technologies, 5977A) were used to compare sensory and flavor components in the cream before and after fermentation. Unfermented imported commercial cream, used as a control group, was compared with the cream fermented with the isolated strain in the present study.
All data are presented as means (±SD) of at least 3 independent experiments; each experiment had 3 replicates of each sample. Data were analyzed statistically using IBM SPSS Statistics software version 25.0 (IBM, Armonk, NY, USA). The statistical difference between the mean values of test groups was analyzed by using one-way analysis of variance (ANOVA). Statistical significance was defined as p=0.05. Multiple comparisons between different groups were assessed using Duncan’s test.
Results and Discussion
A total 84 of LAB were isolated from 21 types of raw milk (data not shown). Among the isolated LABs, Lactobacillus casei LC5229 (hereafter referred to as LC5229), Lactococcus lactis LL5301 (hereafter referred to as LL5301), Lactococcus lactis LL5306 (hereafter referred to as LL5306), and Lactobacillus casei LC5316 (hereafter referred to as LC5316) demonstrated the highest levels of diacetyl production (Table 1). In particular, LL5306 showed the highest level of diacetyl production (13.20±0.54 mg/L). Therefore, LL5306 was selected as the favorable strain for the sour cream manufacturing.
Strain | Diacetyl (mg/L) |
---|---|
L. casei ATCC393 | 10.97±0.55a |
L. lactis cremoris LL5306 | 13.20±0.54b |
L. casei LC5229 | 10.70±0.35a |
L. lactis cremoris LL5301 | 8.13±0.66b |
L. casei LC5316 | 7.90±0.75b |
Monnet et al. (2000) reported that 0.5–6 mM diacetyl was produced by Lactococcus lactis subsp. lactis MR3-T7, in which nitrosoguanidine was used to induce random mutations. Although this corresponds to a concentration of 0.04–0.52 mg/L, the conditions of the medium varied and a higher concentration of diacetyl was produced when yeast extract or catalase was added. According to Guo et al. (2015), when yogurt was fermented by the Lactococcus lactis DX strain, 22.39 mg/L of diacetyl was produced and 2–4 mg/L was produced when buttermilk was fermented. Thus, the similar Lactococcus lactis strain displayed prominent differences in diacetyl production, which depended on each strain and the respective culture conditions.
Table 2 shows the results of the analysis with the API 50 CHL that was used to investigate sugar utilization by the isolated strain, LL5306. As shown in the results in Table 2, LL5306 utilized galactose, glucose, fructose, mannose, raffinose, maltose, cellobiose, lactose, sucrose, sugar alcohols such as mannitol and sorbitol, esculin, amygdalin, and salicin. However, LL5306 did not utilize glycerol, D-xylose, L-xylose, inulin, or starch.
1) The results were compared against the database from Biomerieux at https://apiweb.biomerieux.com.
The sugar utilization results from our study, when compared with the observations on the API website (www.apiweb. biomerieux.com), showed similarity to those of Lactococcus lactis ssp. lactis reference strain 1 (87.7% ID, T index 0.94); the only difference seen was the utilization of amygdalin (75%). The results were also similar to those of Lactococcus lactis ssp. lactis reference strain 2 (12.1% ID, T index 0.73), whereby utilization of D-xylose and xylitol were 1% and 20% different, respectively.
LL5306 demonstrates similarity to Lactobacillus plantarum; however the ID and T index were only 0.1% and 0.54%, respectively. Furthermore, there were considerable differences in the utilization of D-xylose, amygdalin, and trehalose, at 1%, 83%, and 1%, respectively. After using PCR to amplify the 16s rDNA gene of LL5306, sequencing of the 1,335 bp was outsourced to Macrogen (Seoul, Korea) and the sequence was used in a homology search with the NCBI BLASTN program (httrp://blast.ncbi.blm.gov). Following a comparison with the GenBank database and a homology search with the BLASTN program, a phylogenetic tree was constructed using the neighbor-joining method (Fig. 1). The results from this analysis identified the strain as Lactobacillus lactis ssp. cremoris and showed the closest homology with Lactococcus lactis subsp. cremoris strain 3941. There were also similarities to Lactococcus lactis strain, but not as much similarity as to Lactococcus lactis subsp. cremoris. Hence, the isolated strain LL5306 was named Lactococcus lactis cremoris LRCC5306 (Lotte R&D Culture Collection).
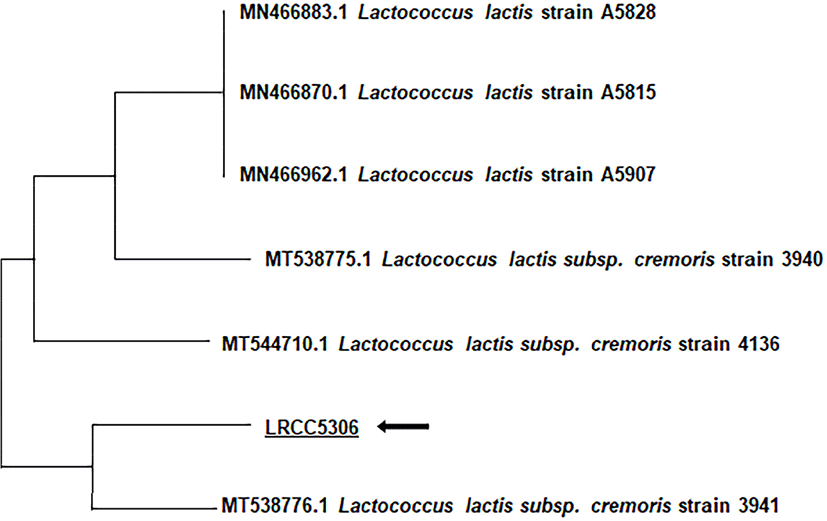
Table 3 shows the results of diacetyl production with various cultivation temperatures. As shown, the optimal culture temperature for production of diacetyl by LRCC5306 was 20°C–25°C, which is similar to previous research which showed that other Lactococcus lactis strains generally show superior secretion of metabolic products at low temperatures of ≤25°C. Diacetyl production was significantly different between 20°C and 25°C; therefore, 20°C was selected as the optimal temperature. Similar to LRCC5306, ATCC373 also showed optimal diacetyl production at 20°C, but showed lower diacetyl production than LRCC5306 at temperatures other than 37°C.
Temperature (°C) | Diacetyl (mg/L) | |
---|---|---|
LRCC5306 | ATCC393 | |
0 | 0.0 | 0.0 |
10 | 0.0 | 0.0 |
20 | 20.64±0.51d | 15.41±0.21c |
25 | 18.32±0.64c | 10.52±0.16a |
30 | 14.25±0.13b | 10.96±0.15a |
37 | 11.48±0.16a | 11.61±0.29b |
Bassit et al. (1995) investigated the optimal temperature for diacetyl production by Lactococcus lactis subsp. lactis biovar diacetilacti and reported that 0.30 mM diacetyl was produced at 18°C and 0.18 mM diacetyl at 30°C, which represents a 1.7-fold difference. The activity of diacetyl reductase, an enzyme that reduces diacetyl to acetoin, was also investigated and was found to be significantly lower at 18°C (2.31 units) than at 30°C (3.29 units). Therefore, it was assumed that lower temperatures inhibit the degradation of diacetyl to acetoin, thereby resulting in a higher concentration of diacetyl remaining in the final fermented product. In contrast, Guo et al. (2015) reported that when yogurt was fermented with Lactococcus lactis DX at 37°C, a diacetyl concentration of 22.39 mg/L was produced, which indicates that even the same strain can manifest differences in diacetyl production.
Table 4 shows the effects of added citrate concentrations on diacetyl during cultivation. As shown in the results, there was a remarkable difference in diacetyl production when citrate was added compared to the untreated samples. Compared to the diacetyl concentration of 19.85±0.38 mg/L in the absence of citrate, almost twice as much diacetyl was produced when citrate was added. However, there was no correlation between the citrate concentration and the diacetyl production. Additionally, there was a slight decrease in diacetyl concentration as the added citrate concentration increased to 2% and above. Therefore, based on the results presented in Table 4, 0.2% was selected as the optimal and economical citrate concentration, which produced the highest diacetyl concentration.
Citrate (%, w/v) | Diacetyl (mg/L) |
---|---|
0 | 19.85±0.38 |
0.1 | 40.48±0.32b |
0.2 | 43.26±0.44b |
0.3 | 42.75±1.41b |
0.5 | 43.01±0.59b |
1.0 | 42.85±1.43b |
2.0 | 34.43±1.64a |
3.0 | 35.20±1.05a |
Fig. 2 shows the diacetyl production when different metal ions were added. As shown in the graph, the highest concentration of diacetyl (66.30±2.23 mg/L) was produced in the group to which Fe2+ ions were added. Additionally, 62.64±1.76 mg/L of diacetyl was produced in the group with added Mn2+ ions; however, there was no statistically significant difference between Fe2+ and Mn2+ (p=0.20). While the addition of Mg2+ or Ca2+ ions showed that there was a trend for a slight increase in diacetyl concentration compared to the treatment with no added metal ions, the p-value was higher than 0.05, indicating that there was no statistically significant difference. Therefore, Fe2+ and Mn2+ ions were selected as the optimal metal ions for diacetyl production.
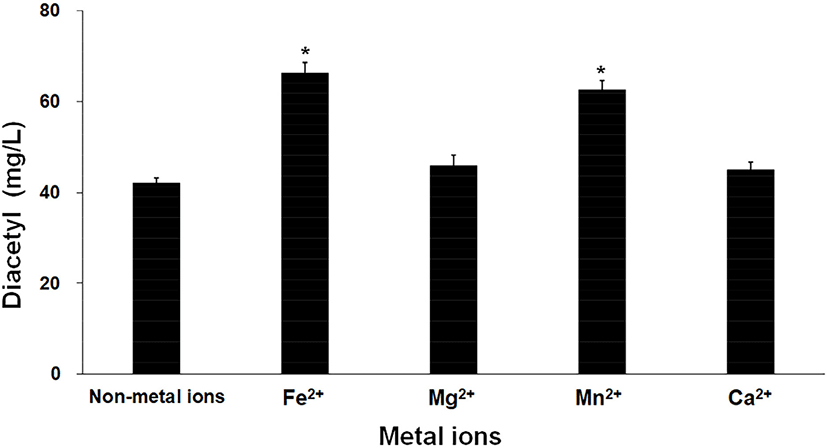
Table 5 shows the comparison of diacetyl production when different concentrations of Fe2+ or Mn2+ ions were added. Neither Fe2+ nor Mn2+ ions at a concentration of 0.0001% resulted in a significant increase in diacetyl concentration, compared to the treatment with no added metal ions; however, the addition of Fe2+ or Mn2+ ions ≥0.001% resulted in a significant increase in diacetyl. As the concentration of Fe2+ or Mn2+ ions increased further, however, there was almost no significant difference in the concentration range of 0.001% and 1.0%. Based on these results, and considering cost-effectiveness, it was determined that the optimal concentration of metal ions was 0.001%.
According to the mechanisms of diacetyl production, the enzyme involved in producing diacetyl from the precursor α-acetolactate is known to be α-acetolactate decarboxylase (Boumerdassi et al., 1996; Ji et al., 2015; Guo et al., 2015). According to Guo et al. (2015), when metal ions were added to a Lactococcus lactis DX cultivation, the relative activity of α-acetolactate decarboxylase was increased; an elevation in activity by 110%, 250%, 300%, and 320% was reported for the addition of Mn2+, Fe2+, Zn2+, and Mg2+ ions, respectively. Kaneko et al. (1990) also reported an increase in diacetyl production when Cu2+ was added to a Lactococcus lactis subsp. lactis 3022 cultivation. Therefore, taken together, the results from previous studies provide evidence that diacetyl production increases with the addition of an appropriate amount of suitable metal ions for a given bacterial strain. In the case of LRCC 5306, 0.001% of Fe2+ was selected as the optimal metal ion.
Fig. 3 shows variation in diacetyl production with cultivation time. As shown in the graph, diacetyl production by LRCC5306 was highest after 14–16 h but decreased thereafter. In particular, the diacetyl concentration of 105.04±2.06 mg/L at 15 h was statistically significant. Therefore, the optimal cultivation time for diacetyl production by LRCC5306 was determined to be 15 h.
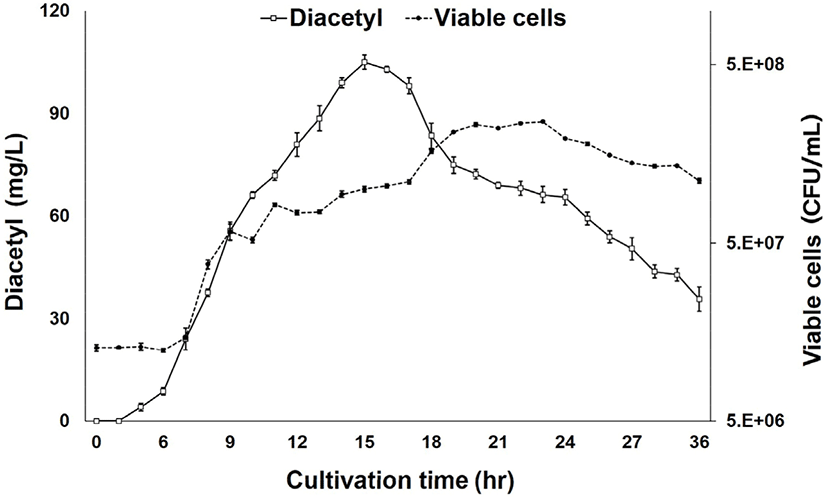
Considering the metabolic mechanisms of diacetyl-producing LAB, α-acetolactate can be produced from pyruvate, which was generated from glucose via glycolysis. The generated α-acetolactate is then converted to diacetyl (Dorau et al., 2019). However, the generated diacetyl is converted to acetoin, depending on several conditions such as the storage time and storage temperature. Therefore, while it is important to produce a high concentration of diacetyl at the end of fermentation, it is also extremely important to optimize the cultivation time that produces the highest concentration of diacetyl (Bondarchuk, 2018).
Boumerdassi et al. (1996) reported that 0.5 mM diacetyl was produced after 8–10 h of culturing Lactococcus lactis ssp. lacfis CNRZ 483 when the oxygen concentration was modulated. In addition, Gebreselassie et al. (2016) using buttermilk collected from a farm in northern Ethiopia reported that the mean diacetyl concentration after 32 h and 48 h of fermentation was 1.32 and 2.97 mg/kg, respectively, and that the highest diacetyl concentration was 7.76 mg/kg.
The results from our study of the growth of LRCC5306 with cultivation time (Fig. 3) showed that the viable cells gradually increased with the passage of time, peaked at 23 h, and slowly decreased thereafter. Thus, there was no correlation between the time of highest diacetyl production at 15 h and the highest viable cells. This indicated that diacetyl is a primarily produced metabolite in the exponential phase of the microbial growth curve. The exact underlying mechanisms need to be examined in further studies.
The viable cells in actual fermented cream, produced by inoculating LRCC5306 culture broth into commercial cream as the seed, were 1.04×108 CFU/g, and the diacetyl concentration was 106.56±1.53 mg/g. Fig. 4 shows the results of sensory properties of sour cream using the electronic tongue system. The sensory components of commercial cream before fermentation were set as the zero-base. In these data, imported commercial sour cream demonstrated a slightly more sour taste than whipped cream, but slightly lower sweetness and saltiness. It was also found that the cream fermented with LRCC5306 showed a similar sweetness and saltiness as the imported product, but a slightly lower bitterness, as well as a significantly increased sour taste.
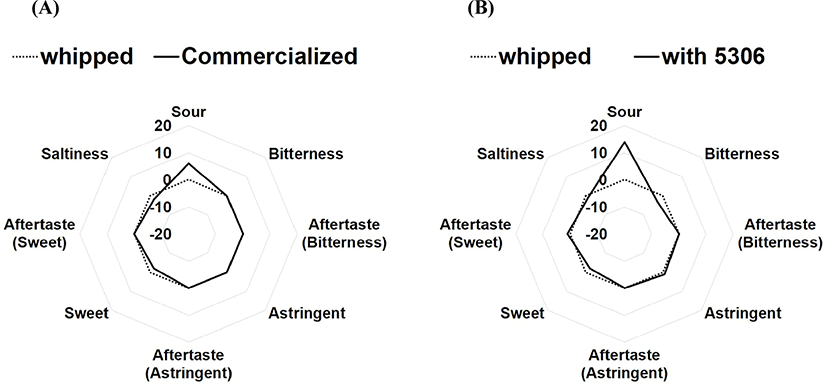
Fig. 5 shows the results of comparing flavor components between commercial whipped cream (unfermented), imported commercial sour cream, and sour cream with LRCC5306. The analyzed flavor components by GC/MS were grouped into six flavor categories. In the whipped cream, the strongest characteristic was ‘sweet’; it has very few components in other flavor categories. In particular, there were almost no buttery, acidic, or cheesy flavor components. In contrast, the imported sour cream and the sour cream with LRCC5306 both showed various changes in flavor components, with a particular increase in buttery and acidic characteristics. Cheesy, milky, and green characteristics demonstrated slight differences between the imported product and the sour cream with LRCC5306. Notably, the sour cream with LRCC5306 possessed almost no cheesy components but had somewhat stronger milky and green components.
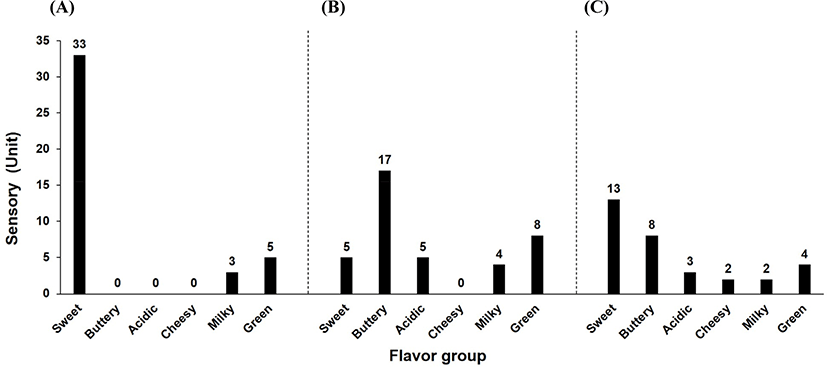
Meunier-Goddik (2004) described sour cream as a product that includes the taste of lactic acid and a balanced, pleasant, buttery-like (diacetyl) flavor. Meanwhile, Shepard et al. (2013) conducted a consumer acceptance test for 32 sour creams in the US based on the results of sensory evaluation and analysis of organic acids and volatile compounds. The flavor with the highest consumer preference was ‘butter-like’ and the active compounds in this flavor included diacetyl and acetoin.
Sour cream with LRCC5306 produced more various flavors and greatly enhanced the buttery flavor that consumers expect from sour cream, compared to the unfermented whipped cream or the imported product. Therefore, it is anticipated that sour cream produced with the LRCC5306 strain could generate a high degree of acceptability among consumers.
LAB must survive the extreme physiological conditions of the upper gastrointestinal tract to exhibit its probiotic function effectively in the intestines. Lactococcus lactis species are generally considered probiotics. We found the viability of LRCC5306 in the presence of hostile gastric and bile acids to be 81 Log % and 96 Log % (% of Log CFU/g), respectively (data not shown).
Further, efficacy studies of sour cream with LRCC5306 as a probiotic on intestinal health are pending. Such studies are important in establishing health implications of sour cream in conditions such as constipation, diarrhea. We consider clinical experiments in animals and humans to render noteworthy results in this regard.
Conclusion
Sour cream has possesses high nutritional value because it contains milk-derived proteins and fats, but it takes a lot of effort to improve its sensory. There is additional loss of diacetyl during fermentation and post-fermentation processes. Also it is very establish fermentation conditions that allow LAB to produce diacetyl optimally.
In the present study, various fermentation conditions were optimized to augment diacetyl concentration using Lactococcus lactis ssp. cremoris LRCC5306. In particular, adding the appropriate concentration of citrate and Fe2+ greatly increased diacetyl production. This effect is thought to be mediated by increased α-acetolactate decarboxylase activity. Our results showed that sour cream manufactured using LRCC5306 possessed superior sensory properties compared to commercialized sour cream. This is anticipated to provide the sensory properties that consumers expect from sour cream, with reduced bitterness and increased sourness. After fermentation with LRCC5306, the viable cell count was over 108 CFU/g. Therefore, in addition to its excellent sensory properties, it is anticipated that this sour cream will act as a source of probiotics. In future research, it will be important to investigate enzymes involved in diacetyl production during LAB fermentation and to explain their mechanisms of action. Moreover, to sustainably improve consumer acceptance, it will be necessary to conduct studies to improve the synergy and balance between flavors following fermentation.