Introduction
Salted egg is a common preserved egg product, also known as salted duck egg. It is usually pickled in brine or mixed with yellow mud, plant ash and salt and is a popular traditional pickled egg product in Indonesia. During pickling, the egg yolks gradually become solidified and hardened. Egg whites lose viscosity and become diluted (Li et al., 2022). The rate of salt penetration of the egg whites and yolks may have an effect on changes in the composition and characteristics of eggs, especially yolks, during pickling. In addition, the marinating time also plays an important role in the formation of salted eggs desired by consumers (Kaewmanee et al., 2011).
During storage, there are many factors that reduce the quality of salted eggs. The most important factor is the oxidation of oil, which not only affects the flavor of salted eggs, but also affects the nutritional value of salted eggs. The oil in salted egg mainly exists in the egg yolk; the main reason for the reduction of the shelf life of salted egg is the oxidation of oil in the egg yolk and the overall quality of the salted egg (Ahn et al., 1995; Harlina et al., 2015). Therefore, it is necessary to reduce the oxidation of oil to prolong the storage time of salted eggs. The oxidation of oil can be reduced by adding synthetic or natural additives (Hayat et al., 2010), and the liquid smoke (LS) with good antioxidant properties can be used as an additive for pickling salted duck eggs.
Many natural antioxidants are based on phenolic compounds. LS contains a lot of phenolic compounds. The constituents in LS include many different compounds such as phenols, ketones, alcohols, esters, aldehydes, acids, furan and pyran derivatives (Montazeri et al., 2013). Among them, phenolic derivatives probably represent the most important one in terms of quality, and phenolic compounds are used to optimize antimicrobial performance, antioxidant potential and sensory properties (Milly et al., 2005). The antioxidant properties of phenols can reduce chronic diseases, degenerative diseases, heart disease, eye diseases, etc. (Hasler, 2000). Adding LS to the product can delay the lipid oxidation process (Estrada-Muñoz et al., 1998). They can act as hydrogen donors, reducing agents, singlet oxygen quenchers and metal atoms and free radicals, thereby neutralizing their toxic effects (Rice-Evans et al., 1996). In particular, methoxyphenols among phenolic compounds and their derivatives have been considered as major contributors to aroma in LS. In addition, they are also the main substances responsible for the antibacterial and antioxidant effects of smoked foods (Guillén and Ibargoitia, 1998; Suñen et al., 2003).
At present, in Indonesia, people’s living standards are gradually improving, and the requirements for consumer goods are getting higher and higher, and at the same time, the processing industry of egg products is gradually becoming more advanced and modern. Therefore, innovation in the processing of salted duck eggs, increasing the shelf life of salted duck eggs and developing salted egg products with more functional properties can be achieved and need to be achieved. At present, domestic scholars have developed many new pickling techniques, which have improved the flavor and storage time of salted eggs. In recent years, developed countries have been very advanced in food processing, and LS technology is basically used for popular smoked products such as bacon. Little research has been done on egg products, especially as food additives or new processing methods for preserved egg products.
The purpose of this study was to investigate the effects of LS as an additive for pickled salted duck eggs on the antioxidant properties, volatile flavor compounds, and texture of salted duck eggs. Studying the antioxidant properties of LS and the influence of its unique flavor in salted eggs is not only beneficial to provide new ideas for the study of extending the shelf life of salted duck eggs, but also to develop new types of salted duck eggs with smoked flavors to increase consumers’ choices and developed the new markets.
Materials and Methods
Methanol, ethanol, NaOH, NaCl, potassium ferricyanide, FeCl3, 2-thiobarbituric acid (TBA), trichloroacetic acid (TCA), 1-diphenyl-2-picrylhydrazyl (DPPH) were all Pro Analysis quality chemical reagents. LS were bought from Lubna Company (Semarang, Indonesia). LS was extracted from coconut shell pyrolysis that produces LS containing 4.13% phenolic compounds, 11.3% carbonyl and 10.2% organic acids.
Fresh duck eggs were harvested within three days of laying were purchased from local producers. After thoroughly cleaning numerous duck eggs with water, place the eggs in a solution of salt that is 18% (w/w; salt solution:duck eggs=1:1, w/w), the egg samples were then treated with LS (Liquid Smoke Food Grade A, Lubna Company) at concentrations of 2.5% and 5% (v/v) and curing them for 28 days.
According to Harlina et al. (2018), oxidative rancidity of fat was assessed using a thiobarbituric acid reactive substances (TBARS) assay of malondialdehyde (MDA). Briefly, 5 g of the sample was combined with 25 mL of TCA 7.5%, homogenized, and left at room temperature for 1 hour. The solution was centrifuged (model 3,740, Kubota, Osaka, Japan) at 2,000×g for 10 min. The filtrate was then added 50 mL of purified water. 5 mL of the prepared solution and 5 mL of 0.02 M TBA were combined. A UV-vis spectrophotometer was used to test the reaction mixture’s absorbance at 532 nm after being maintained in a water bath at 100°C for 15 min. In a 50-mL volumetric flask, distilled water was added to 25 mL of 7.5% TCA, and the resulting solution (5 mL) was combined with 5 mL of 0.02 M TBA to create the blank sample. Malondialdehyde standard curve data was used to determine TBARS value, which was then represented as mg MDA/g sample.
Combining egg white and yolk with 95% ethanol at a ratio of 1:10 (w/v), the mixture was extracted for 2 h at 60°C while being continuously shaken at a speed of 170×g. The resulting slurry was centrifuged for 10 min at 10,000×g. The antioxidant activity of the supernatant was examined. Salted duck eggs treated with LS were examined in vitro for their antioxidant properties, including DPPH radical scavenging activity and reducing power assay.
Free radical scavenging activity was used to measure the antioxidant activity (DPPH method). It was made with a 0.002% concentration of DPPH. Supernatant samples were obtained at various concentrations and formed up to 2 mL quantities in each test tube. Each test tube was then filled with 2 mL of the DPPH solution, which was then left in the dark for 30 min. Each sample was examined three times. Using a UV-Visible spectrophotometer, optical density was later measured at 517 nm. As a control, ethanol combined with DPPH was employed. With a small modification, the procedure was the same as that employed by Harlina et al. (2019). The formula was:
Where: A=Optical density of control; B=Optical density of sample.
The reducing power assay was evaluated described by Harlina et al. (2018). In a summary, 2.0 mL of sample was combined with 2.0 mL of 1% (w/v) potassium ferricyanide and 2.0 mL of 0.2 mol/L sodium phosphate buffer (pH 6.6). The aforementioned combination was incubated for 20 min at 50°C. Two milliliters of 10% TCA was then added after cooling. The supernatant was recovered after centrifugation at 3,000×g for 10 min, and 2.0 mL was combined with 2.0 mL of distilled water and 0.4 mL of 0.1% (w/v) FeCl3. After 10 min of mixtures standing at room temperature, the absorbance at 700 nm was measured. As a blank, the same amount of distilled water was used.
The control and treated eggs were put in 20 mL headspace extraction bottles. CAR/PDMS Fused Silica 24 Ga (Manual Holder, 3pk; Supelco, Bellefonte, PA, USA) extracted for 2 h, desorbed in the GC-MS inlet for 5 min, and determined volatile flavor compounds. GC-MS conditions: Agilent 7890B/5977A (Agilent, Santa Clara, CA, USA) GC-MS, HP-5 (30 m ×0.25 mm×0.25 mm) column, inlet temperature 230°C, split less injection. Heating program: the initial temperature was 40°C, maintained for 6 min, increased to 135°C at a heating rate of 3°C/min, and then increased to 230°C at a heating rate of 6°C/min and held for 10 min. Mass spectrometry conditions: EI ion source, ion source temperature 200°C, electron capacity 70 ev, filament current 150 μA, scanning mass range 33–450 u.
FOX-3000 electronic nose (E-nose; Alpha MOS, Toulouse, France) was used to collect and evaluate data. It contains an HS-100 autosampler, sensor array, air generator tool, sampling instrument, and software called Alpha Soft V11. Eighteen metal oxide sensors are put in the sensor array, which is separated into three series chambers called T, P, and LY (Li et al., 2012). Take 2–3 g of the egg yolk sample into a 10 mL headspace vial, seal and equilibrate for 30 min. Using pure air as the carrier gas, the vial was put into an autosampler and equilibrated at 50°C for 10 min at a flow rate of 200 mL/min. The stirring speed was 250×g, the injection volume was 2,500 mL, and the injection time was 3 s. The injection delay time was 180 s, while the acquisition time was 50 s. Analysis was conducted using the electronic nasal maximum response point that was automatically recorded with each of the 18 sensors (Song et al., 2013). Three different evaluation samples were used.
Texture analysis can be used to determine a product’s texture. A TA.XT Plus texture analyzer was used to determine the texture of salted egg yolk. Samples of egg yolks were divided in half before texture analysis with a compressed 36 mm diameter cylindrical probe. Each sample is compressed after being sandwiched between specific stainless steel plates (using a texture analyzer at room temperature). Prior to and during the test, the texture analyzer’s speed was 2 and 5 mm/s, respectively. At an intermediate speed of 3 mm/s, the torque-deformation curves were captured. Evaluations included hardness, cohesiveness, elasticity, tackiness, chewiness, and recovery. The software Micro Stable was used to obtain these parameters (Stable Micro System, Godalming, UK). Three replicates were used on average.
Experiments were performed in triplicate. Data are presented as SD. One-way analysis of variance (ANOVA) was performed using SPSS 20.0 software (SPSS, Chicago, IL, USA). Means were compared using Duncan’s multiple range test to determine the least significant difference between methods at a significance level of 0.05.
Results
Malondialdehyde, a result of lipid peroxidation that can be represented by TBA values, can be created from polyunsaturated fatty acids with at least three double bonds (Ayala et al., 2014). The concentration of the reaction product, TBARS was calculated by reacting the peroxidation product in the sample with thiobarbituric acid. Due to the presence of TBA reactive substance resulting from the oxidation of peroxides to aldehydes and ketones during the second stage of autoxidation, the TBA value has been commonly employed to quantify the extent of lipid oxidation (Song et al., 2011). The equation derived from the standard curve was used to compute the TBA value, which was then represented as mg (MDA)/kg. Fig. 1 displays the findings of the TBA values for the control group and the LS-treated salted eggs during the duration of the 28 day curing period.
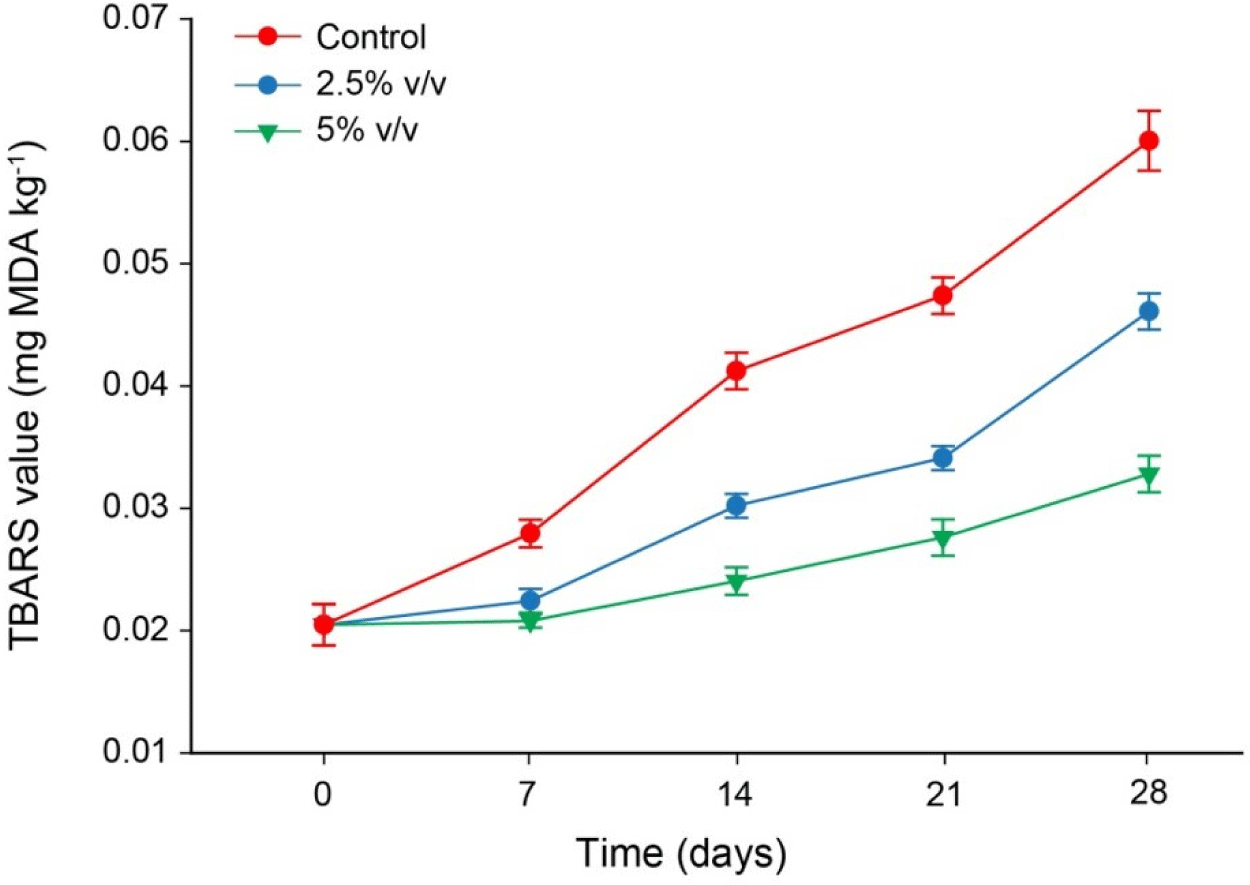
During the 28 day marinating period, TBA value of the salted egg yolk considerably increased over time (p<0.05). The steady increase in the degree of partial oxidation and dehydration of unsaturated fatty acids may be responsible for the rise in TBA value. The TBA value of the control sample was considerably different from the samples that had been treated with LS (p<0.05). The TBA value of the control sample was higher than that of the salted egg treated with LS, and the TBA value decreased with increasing LS concentration. The oxidation reaction is inhibited by the high LS concentration of phenolic compounds and their derivatives, which have good antioxidant activity and can be employed as antioxidants. According to Estrada-Muñoz et al. (1998), the instability of the generated MDA or the oxidation of various lipid populations at various intervals could both account for the rise and fall in TBA values at different storage times.
Fig. 2 displays the changes in the free radical scavenging rate of the salted eggs treated with the LS and the control group throughout the duration of the 28 day curing period. According to the findings, during the first 14 days of curing, salted duck eggs treated with LS considerably improved over time at scavenging DPPH free radicals in the yolk and protein (p<0.05). After the 14th day, the amount of the increased protein capacity to scavenge free radicals reduced with time and tended to stabilize in the salted egg yolk treated with LS. During the 28-day curing period, free radical scavenging rate of the control group did not rise significantly (p>0.05).
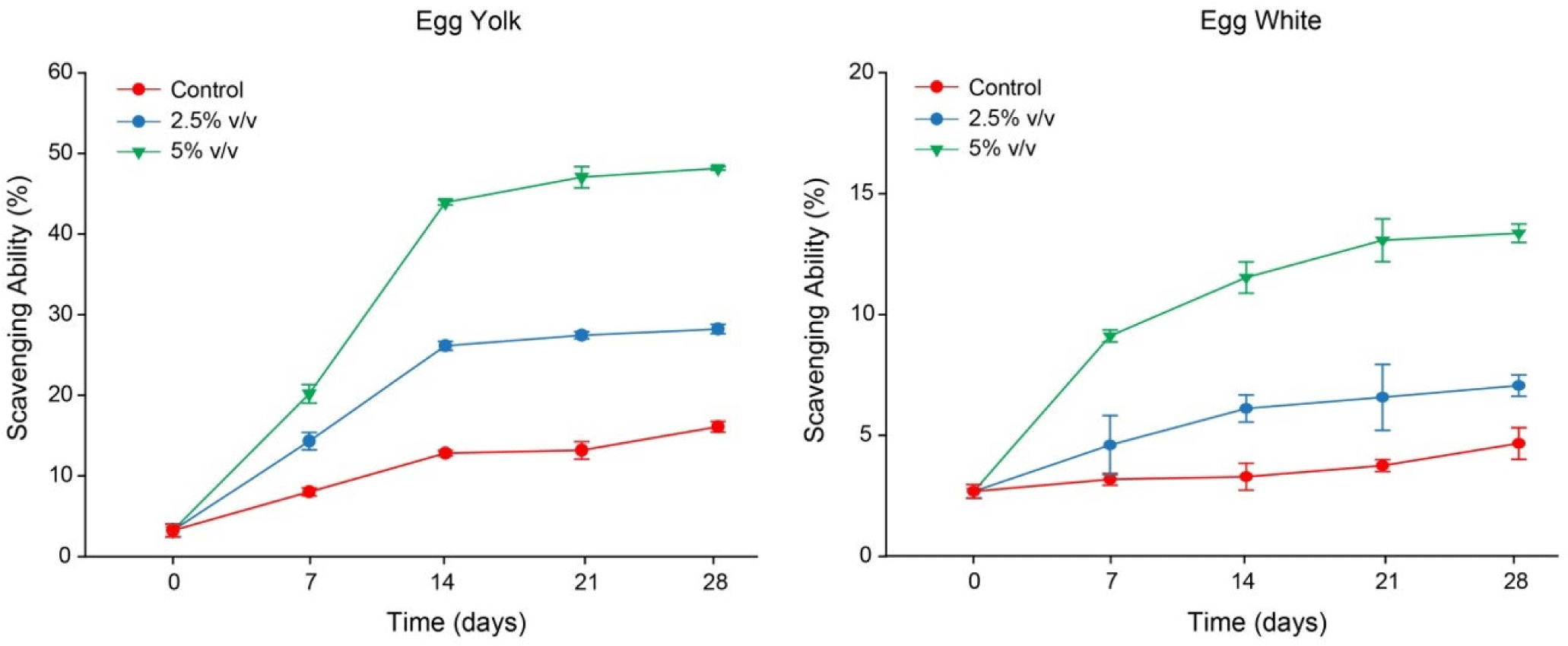
Salted eggs supplemented with LS 2.5% and 5.0%, (v/v) considerably outperformed the control sample in terms of their ability to scavenge free radicals (p<0.05), and it was found that the concentration of LS was inversely correlated with the rate of free radical scavenging. Egg yolks often scavenge free radicals at a higher rate than egg whites.
Fig. 3 illustrates changes in the reduction power of the salted eggs in the control group and the salted eggs treated with LS over the duration of the 28-day curing period. The results indicated that reduction power of salted egg yolk significantly increased over the duration of the 28-day pickling period (p< 0.05). After the first seven days (p<0.05), the reducing power of the control of salted egg white and the 2.5% (v/v) LS increased dramatically with time and then progressively stabilized. With the exception of the second week during the 28 curing cycles, the reducing power of salted egg white in 5.0% (v/v) LS increased significantly over time (p<0.05). The reducing power of salted eggs added with LS (2.5% and 5.0%, v/v) was significantly higher than that of the control samples (p<0.05). The reducing power of egg yolk is overall higher than that of egg white.
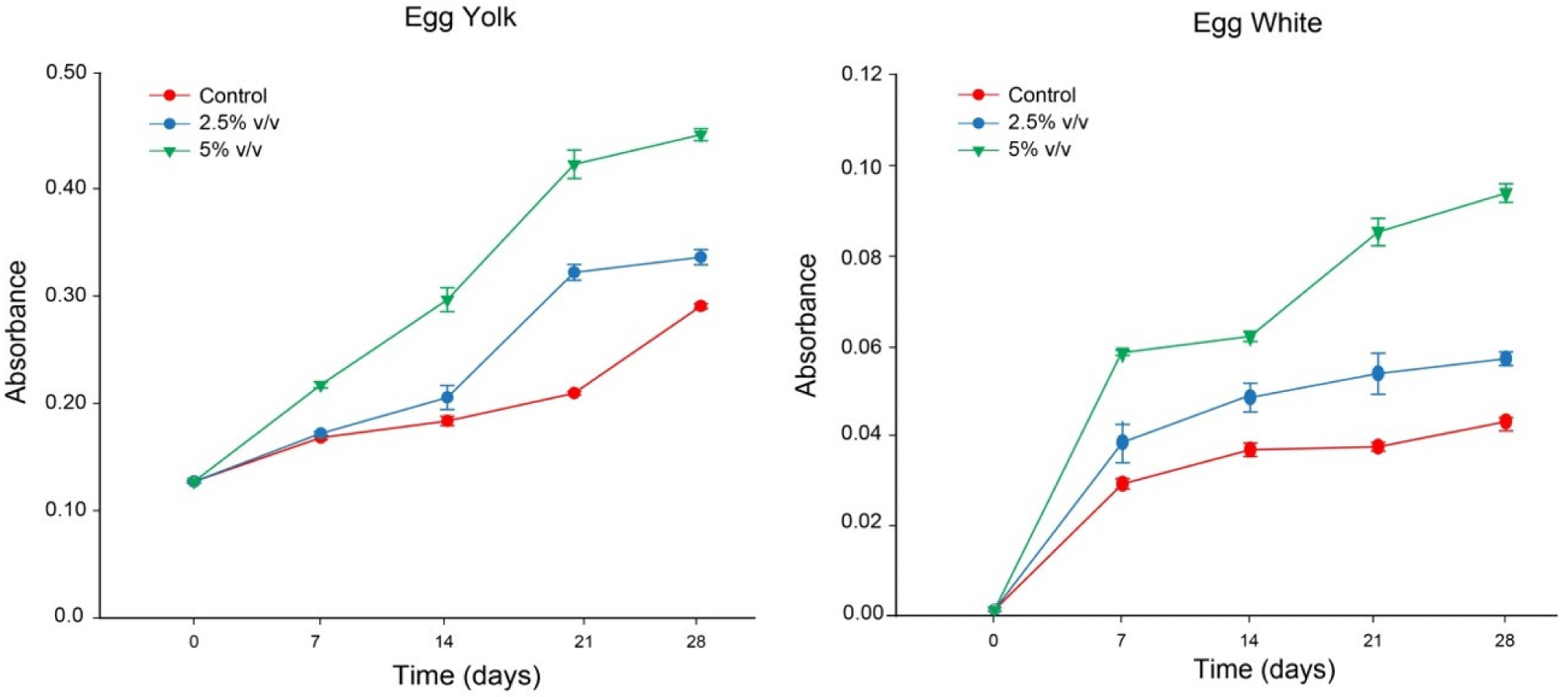
Phenolic compounds, which compose the majority of LS, act as reducing and antioxidant agents by chelating metal ions or providing hydrogen to prevent the oxidation chain reaction (Bursal and Köksal, 2011). The electrons provided by natural antioxidants are frequently calculated using the reducing force (Dorman et al., 2003). According to You et al. (2010), antioxidants cause Fe3+/FeSCN complexes to degrade into the Fe2+ form, which results in the formation of reducing power in the samples. The strongest reducing power is demonstrated by the absorbance at 700 nm (Duh, 1998; Memarpoor-Yazdi et al., 2013). Yellow color of sample reagent turns green or blue-green during the test. Antioxidants that act as a reducing agent transform ferricyanide from its complicated state to its simple ferrous form. As a result, Fe2+ content can be determined by observing Prussian blue absorption at 700 nm. The ability of a phenolic antioxidant to provide electrons is key, and its indication, the valence reduction of Fe(III), is frequently used (Dorman et al., 2003).
Lipid oxidation is the main reaction that produces volatile flavor compounds, which can improve flavor. The volatile substances found on fresh duck eggs and control salted duck eggs by GC-MS are listed in Table 1. There are 3 esters, 5 acids, 1 ketone, 3 aldehydes, 5 nitrogenous compounds, 1 ether, 2 hybrid cyclic compounds, 3 hydrocarbons, and 1 other substance among the 24 volatile compounds found in fresh duck eggs, which are classified into 9 groups. The salted duck eggs of the control group included 21 volatile components that could be categorized into 10 different groups. These included 1 ester, 5 acids, 3 alcohols, 1 ketone, 4 aldehydes, 5 nitrogenous compounds, 1 ether, 1 heterocyclic compound, 3 hydrocarbons, and 1 other substance.
The volatile flavor components found on salted duck eggs that were cured in LS 2.5% (v/v) that analyzed by GC-MS are listed in Table 2. 53 phenols, 8 esters, 3 acids, 4 alcohols, 32 ketones, 11 aldehydes, 6 nitrogen-containing compounds, 3 ethers, 7 heterocyclic compounds, 18 hydrocarbons, and 2 others are among the 147 volatile substances in LS, which are subdivided into 11 categories. A total of 63 volatile compounds, subdivided into 8 categories, were found in salted duck eggs that were cured in LS at a 2.5% (v/v) concentration. These included 23 phenols, 2 esters, 1 acid, 2 alcohols, 13 types of ketones, 4 types of aldehydes, 2 types of heterocyclic compounds, and 16 types of hydrocarbons. For total ion chromatogram of volatile flavor component from these samples can be seen in Supplementary Fig. S1.
Antibacterial, antioxidant, and organoleptic qualities are connected to phenols and their derivatives. Numerous phenolic substances, primarily phenol, guaiacol, eugenol, etc., are present in LS. When phenolic compounds are thermally degraded, they sometimes contain dihydroxybenzene derivatives, which have a high antioxidant activity (Guillén and Manzanos, 2002). Macromolecular polymers that are randomly cross-linked make up lignin. It is composed of a disorganized collection of hydroxyl groups with various linkages and methoxy-substituted phenylpropane units. Pyrolysis involves competing thermal degradation reactions that, depending on the bond energies involved, lead to various bond breakage. The considerable structural variety of lignin allows for a wide range of compounds to be produced during pyrolysis (Guillén and Ibargoitia, 1998).
Salted duck eggs marinated in 2.5% (v/v) LS contained a significant amount of phenolic chemicals. Fresh duck egg and salted duck egg without LS did not contain phenolic substances. Additionally, only a very little number of ketones were identified in fresh duck eggs and salted duck eggs without LS but there were 32 and 13 ketones in the LS and treated eggs with LS 2.5% (v/v), respectively. The primary element responsible for the color and flavor of smoked goods is ketone. Carbonyl groups found in ketones can interact with amino groups in protein molecules to produce a distinctive smoky color.
Fresh duck eggs, salted duck eggs in the control group, and salted egg yolks cured in 2.5% (v/v) LS, they all contained trace amounts of esters, alcohols, and aldehydes. Esterification is the process by which fatty acids and alcohols are converted into esters. Alcohols often make minor contributions to food taste (Barclay et al., 1997). Aldehydes are mostly produced as a result of the oxidation of fats and oils during heating (Mensink et al., 2003). Therefore, fresh duck eggs, the control group, and salted egg yolks treated with LS did not contain a significant amount of aldehydes. Salted duck eggs were cured in 2.5% (v/v) LS, and significant amounts of hydrocarbons were found in both. Alkenes dominate the liquid-cured, salted duck egg yolks.
The electronic nose (E-nose) imitates the olfactory system of mammals by employing a number of sensors that react to volatile substances (Cramp et al., 2009). The E-nose is a device that combines a gas sensor structure and an air sample tool with a personal computer. The ability of sensor array function of the E-nose device to react differently to distinct flavors sets it apart from other devices used for flavor characterization. Hundreds or even thousands of distinct volatile flavor components can be found in each flavor. The identification and measurement of numerous flavor constituents in a single flavor sample is accomplished by widely used analytical techniques like GC-MS. However, unlike the human nose, which analyzes flavors as a whole and detects them by our brains (for example, by identifying patterns of flavors in memory), the E-nose can react to a sample of total flavor compounds. This is because the human olfactory system does not require isolation of individual compounds as part of the sample analysis process (Cramp et al., 2009).
Figs. 4 and 5 show the PCA analysis and radar chart of the volatile flavor E-nose in fresh duck eggs, the control group, and the eggs treated with LS (2.5% and 5.0%, v/v), respectively. In the PCA principal component analysis graph, the abscissa represents the first principal component and the ordinate represents the second principal component, both of which show that salted duck eggs differ significantly from fresh duck eggs and those treated with LS. The radar chart demonstrates that fresh duck eggs had considerably lower relative values of T40/2, P30/2, P40/2, P30/1, PA/2, T70/2, P40/1, P10/2, and P10/1 than a sample of treated eggs. Salted eggs supplemented with LS (2.5% and 5.0%, v/v) had significantly lower PA/2, T70/2, P40/1, P10/2, P10/1, and T30/1 values than the control samples. E-nose sensor intensity for volatile compounds of these samples is shown in Supplementary Fig. S2.
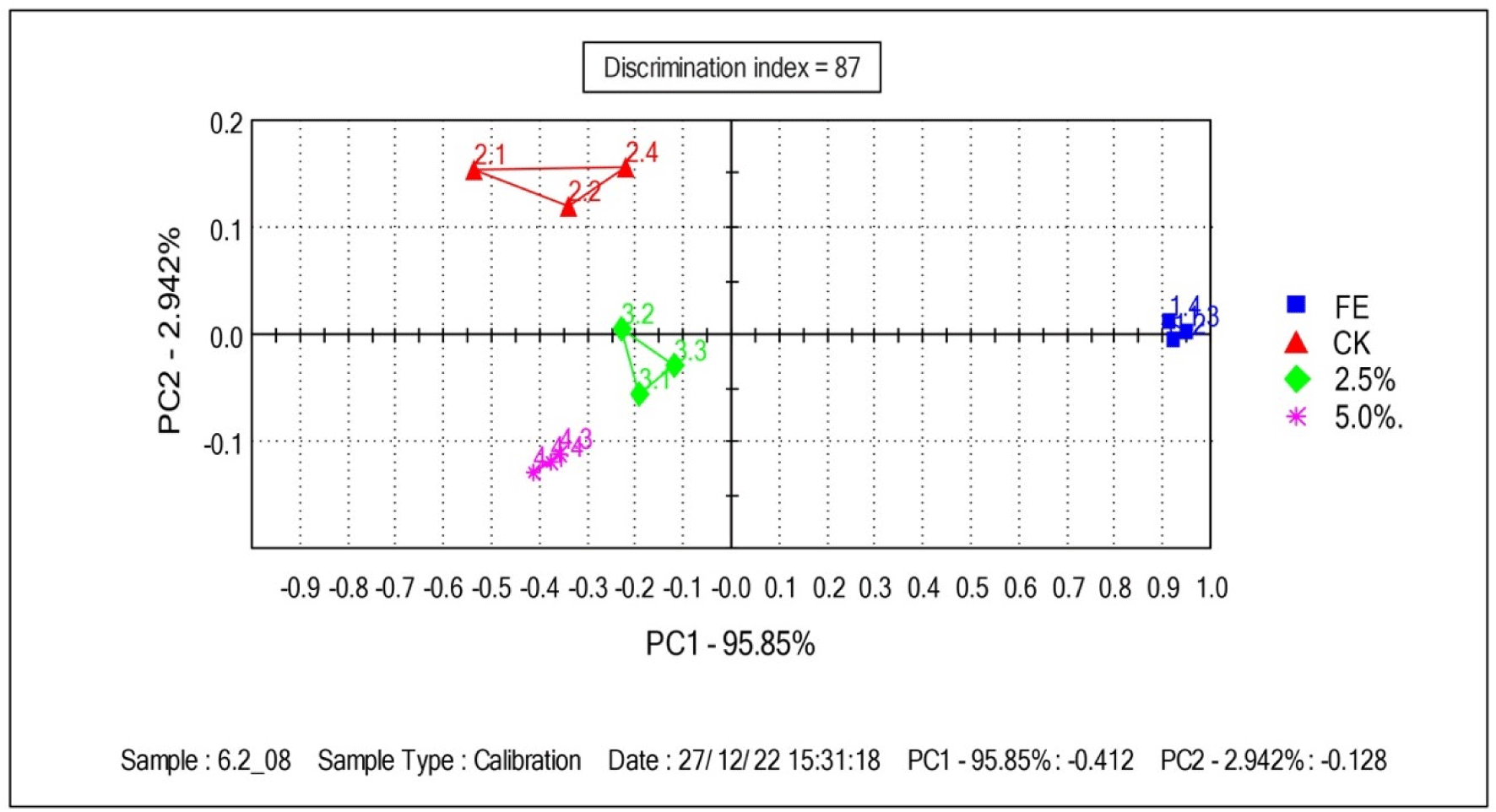
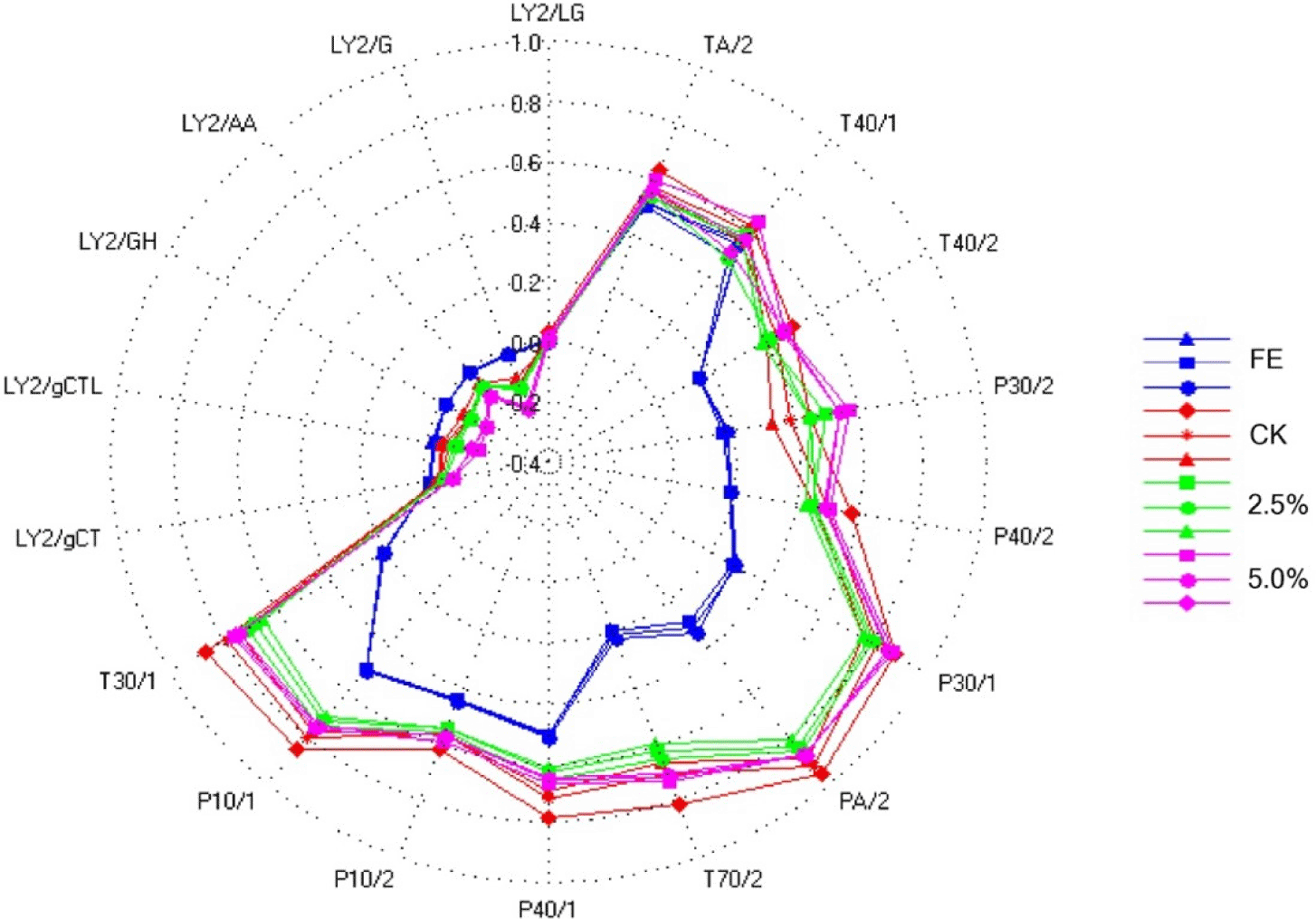
Salted egg quality is correlated with the product texture. Table 3 summarizes the texture of salted eggs enriched with LS (2.5% and 5.0%, v/v) and the control group after 28 days of curing. Significant changes in hardness may be seen between control samples and salted egg yolks treated with various concentrations of LS (p<0.05). The results showed that the structure of the salted duck egg yolk became more solidified in the eggs treated with LS, but the hardness of the egg yolk was reduced if the concentration of the LS was too high. Kaewmanee et al. (2009a) and Kaewmanee et al. (2009b) have reported that the hardness of salted duck egg yolk is associated with an increase in the hardening rate. The hardening rate of the yolk is defined as the ratio of the hard outer yolk to the weight and is used as an indicator of the completion of the pickling. The more dehydrated the marinated egg yolks are, the rougher the texture will be.
Between the control group and the LS-treated salted yolk, there was no noticeable change in terms of elasticity (p>0.05). The three groups of salted duck eggs varied significantly (p<0.05) in terms of cohesion and chewiness. In addition, the control and 2.5% (v/v) LS-treated eggs were significantly different from the 5.0% (v/v) LS-treated egg for gumminess and resilience (p<0.05), the gumminess and resilience of eggs control and 2.5% (v/v) LS were higher than those of egg treated with 5.0% (v/v) LS. It is possible that the excessively high concentration of LS causes the protein in the egg yolk to denature and alter its structure, making it flakier and lowering its viscosity and recovery. The picture of control and treated of salted duck eggs after 28 days of salting can be seen in Fig. 6.
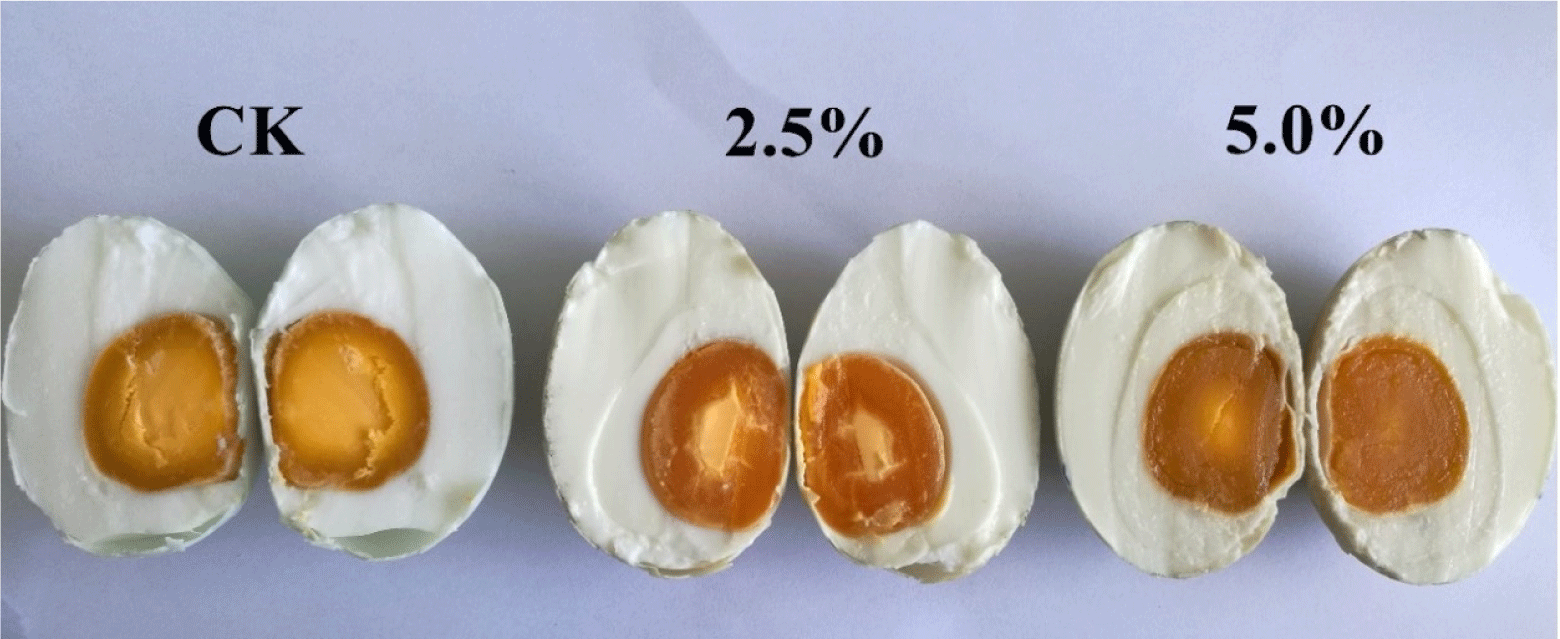
Discussion
In recent years, the use of LS as a flavor enhancer and preservative in food products has increased. Salted duck eggs, in particular, are a popular traditional food product in many cultures, and the addition of LS to this product has intrigued the interest of researchers due to its potential effect on the antioxidant activity and lipid oxidation of the eggs. LS contain a variety of compounds with antioxidant properties, including phenols and polyphenols (Montazeri et al., 2013). These compounds may contribute to the overall antioxidant capacity of salted duck eggs, preventing lipid oxidation and maintaining egg quality (Li et al., 2022). LS can improve the antioxidant properties of salted duck eggs by increasing their DPPH scavenging activity and reducing power (Soldera et al., 2008). This is most likely because LS contains phenols and polyphenols, both of which have been shown to have powerful antioxidant properties.
The fact that the hydroxyl group of the phenolic component in LS can provide stable free radicals and reduce fat oxidation caused by the interaction of free radicals and fatty acids may explain its high antioxidant activity. Phenols and their derivatives are most likely the primary class of chemicals in LS that act as antioxidants and free radical scavengers (Montazeri et al., 2013). Polyphenolic substances such as flavonoids and phenols have also been studied and found to have a variety of biological effects, including antioxidant activity (Kumar and Pandey, 2013; Shahidi and Ambigaipalan, 2015; Tungmunnithum et al., 2018).
According to Barclay et al. (1997), the antioxidant properties of phenolics and their derivatives are derived from lignin (4-propylguaiacol, eugenol, isoeugenol, and 4-allyl-2,6-dimethoxyphenol). These substances, according to reports, have greater antioxidant potential than commercially available 2,6-di-tert-butyl-4-methylphenol (BHT). The primary goal of anti-oxidation is to delay oxidation by avoiding and limiting free radical production. Antioxidants can be formed from phenolic compounds and their derivatives. As a result, using LS as a good source of phenolic chemicals can benefit functional foods. Antioxidant properties can also be used to inhibit oxidative food reactions (Albishi et al., 2013).
The addition of LS to salted duck eggs can alter their volatile flavor profile significantly. LS can improve the overall flavor of the eggs while also adding a smoky, woody aroma. This is most likely because the LS contain volatile flavor compounds such as phenols, aldehydes, and ketones (Guillén and Ibargoitia, 1998; Suñen et al., 2003). It can make the eggs more tender and juicy by improving their texture. Because the presence of volatile flavor compounds in the LS is likely, this can improve the sensory properties of the eggs and increase their overall appeal.
Conclusion
Comprehensive analysis of the experimental findings revealed that the LS did have a significant impact on the antioxidant properties of salted duck eggs, which may have the potential to enhance the antioxidant capacity of these eggs to some extent and alleviate the oxidation of the lipids in the egg yolk. In addition, GC-MS detected several phenolic compounds in both the LS and the salted duck eggs treated with LS groups. These compounds may be the primary factors contributing to the eggs treated with LS having increased antioxidant activity. Additionally, LS can alter the physical characteristics of the salted duck eggs, such as hardness, cohesiveness, chewiness, oil exudation, color, etc., for the egg yolks. Therefore, LS may be beneficial to increase the sensory quality of salted duck eggs.
SUPPLEMENTARY MATERIAL
Supplementary materials are only available online from: https://doi.org/10.5851/kosfa.2023.e10.