Introduction
Meat and meat products are generally consumed after a cooking process. Cooking processes improve digestibility, reduce exposure to microorganisms and give meat and meat products unique sensory and nutritional properties (Cordeiro et al., 2020; Nuray and Oz, 2019; Sobral et al., 2018). However, cooking processes can cause carcinogenic compounds such as polycyclic aromatic hydrocarbons (PAHs; Bouvard et al., 2015; Sun et al., 2019).
PAHs are a type of hydrocarbon molecule containing two or more benzene rings. Naphthalene, acenaphthylene, acenaphthene, fluorene, phenanthrene, and anthracene are categorised as light PAHs consisting of two to three rings. Fluoranthene, pyrene, benzo[a]anthracene, chrysene, benzo[b]fluoranthene, benzo[k]fluoranthene, benzo[a]pyrene, indeno[1,2,3-cd]pyrene, dibenzo [a,h]anthracene, and benzo[g,h,i]perylene are categorised as heavy PAHs because they consist of four or more rings (Hamidi et al., 2016). Light PAHs have very low toxicity because they are more volatile, while heavy PAHs have higher toxicity because they are more stable (Ewa and Danuta, 2017).
The incomplete combustion or pyrolysis of organic material leads to the formation of PAHs (Lee et al., 2019; Sun et al., 2019). Currently, more than 10,000 types of PAHs are known, of which about 660 different PAH compounds have been identified so far (Hwang et al., 2019; Masuda et al., 2019). The US Environmental Protection Agency has listed 16 PAH compounds [light PAHs (6)+heavy PAHs (10) as mentioned above] commonly found in samples that exhibit high toxicity in humans (Zelinkova and Wenzl, 2015). One of the main ways to come into contact with PAHs is through eating food, especially grilled and smoked meat products (Domingo and Nadal, 2015).
PAHs in grilled and/or barbecued meat and meat products can be formed in three ways. One way is pyrolysis of organic material directly on the surface of the food. Another way is smoke contamination from the heat source. Another way is contact of dripping fat with charcoals that produce smoke, including PAHs. The most important factors affecting level of PAHs during grilling are the distance from the heat source, the fat level of the raw product and the grilling time (Cordeiro et al., 2020; Rose et al., 2015; Viegas et al., 2012). In addition, the different chemical characteristics of the various charcoals could affect the formation of PAHs during charcoal grilling (Kim et al., 2021).
One of the most popular cooking methods for meat and meat products is grilling, which gives them a unique aroma and flavour (Oz and Kaya, 2019; Viegas et al., 2012). Many types of charcoal, such as wood charcoal and/or charcoal from organic waste material (e.g., coconut shell charcoal, hazelnut shell charcoal), are used as a heat source in grilling (Kim et al., 2021). Oak wood charcoal is produced at about 600°C–650°C (Pehlivan and Kahraman, 2011) and is generally used for cooking on grills. Another charcoal that is often used for grilling in Türkiye is orange wood (Citrus sinensis) charcoal, which is easy to ignite. The Valonia oak (Quercus ithaburensis subsp. macrolepis) is a deciduous tree endemic to the eastern Mediterranean region, growing naturally in western and southern Anatolia (Türkiye), Greece and southern Albania (Pantera et al., 2008). It was reported that the wood of the Valonia oak was used extensively for the production of charcoal (Giannakopoulou, 2002). Marabu is known as Dichrostachys cinerea and originates from Africa, but was transplanted to Cuba because of its attractive flowers. Cuba produces charcoal from the marabu wood, which is used for grilling and generates considerable income (Idowu and Fletcher, 2020). Extruded charcoal is made by pressing organic waste with kindling such as barium nitrate (Sepetdjian et al., 2010). The chemicals in the extruded charcoals could cause hazardous substances when burned for cooking (Kim et al., 2021). Coconut waste accumulates everywhere in the form of shells, husks and coconut fibres from the coconut milk and oil processing industries (Ahmad et al., 2020). Coconut shells are a good source of charcoal due to their high lignin content (Yerima and Grema, 2018). Hazelnut shells are a potentially important source of energy for Türkiye. The use of agricultural residues to produce charcoal is an alternative process to the other energy sources (Demirbaş, 1999).
There are limited studies in the literature investigating the effects of various types of charcoal on the formation of PAH in meat products (Kim et al., 2021; Oz, 2021; Viegas et al., 2012). Viegas et al. (2012) investigated the effects of wood and coconut charcoal on PAHs in samples of beef and salmon. Kim et al. (2021) found the impacts of three different charcoals on the occurrence of PAH in grilled beef, pork and chicken meat samples. Oz (2021) determined the effects of two charcoals (briquette and wood charcoal) and fat content on the PAH content of five grilled fish species. However, as far as we know, no study has yet examined the effects of many charcoals such as orange wood charcoal, marabu wood charcoal, hazelnut shell charcoal, and Valonia oak wood charcoal used in grilling on the formation of PAHs in meat and meat products. Therefore, due to the lack of a comprehensive study on the effects of various charcoals on the occurrence of PAH compounds in meat products, the current study aimed to determine the effects of various charcoals on the formation of PAH16 in grilled beef steaks and patties. In addition, the effects of fresh and processed meat products on the occurrence of PAHs during grilling were also determined. Another goal of this study was to suggest a suitable charcoal for grilling meat products to limit exposure to PAHs.
Materials and Methods
All chemicals and solvents used in the current study were suitable for high-performance liquid chromatography and were of analytical grade. The standards for 16 PAHs (naphthalene, acenaphthylene, acenaphthylene, fluorene, phenanthrene, anthracene, fluoranthene, pyrene, benzo[a]anthracene, chrysene, benzo[b]fluoranthene, benzo[k]fluoranthene, benzo[a]pyrene, indeno[1,2,3-cd]pyrene, dibenzo[a,h]anthracene and benzo[ghi]perylene) were purchased from Supelco (Oakville, ON, Canada).
The beef steaks used in this study were obtained from the Longissimus dorsi of beef carcasses. The muscle was purchased from a butcher in Konya, Türkiye. For the preparation of the beef patty samples, the beef (Biceps femoris) and beef fat were obtained from a butcher in Konya, Türkiye. The salt (Salina, Ankara, Türkiye) used in the production of beef patty samples was purchased from a market in Konya.
Seven different types of charcoal were obtained: charcoal from oak wood (C1; Konya, Türkiye), charcoal from orange wood (C2; Konya, Türkiye), charcoal from Valonia oak wood (C3; Isparta, Türkiye), charcoal from marabou wood (C4; Cuba), extruded charcoal (from beech wood, starch and barium nitrate; C5; Russia), charcoal from coconut shells (C6; Indonesia), and charcoal from hazelnut shells (C7; Giresun, Türkiye). The details of the charcoals used are given in Table 1. The photographs of the different charcoals used in this study are shown in Fig. 1.
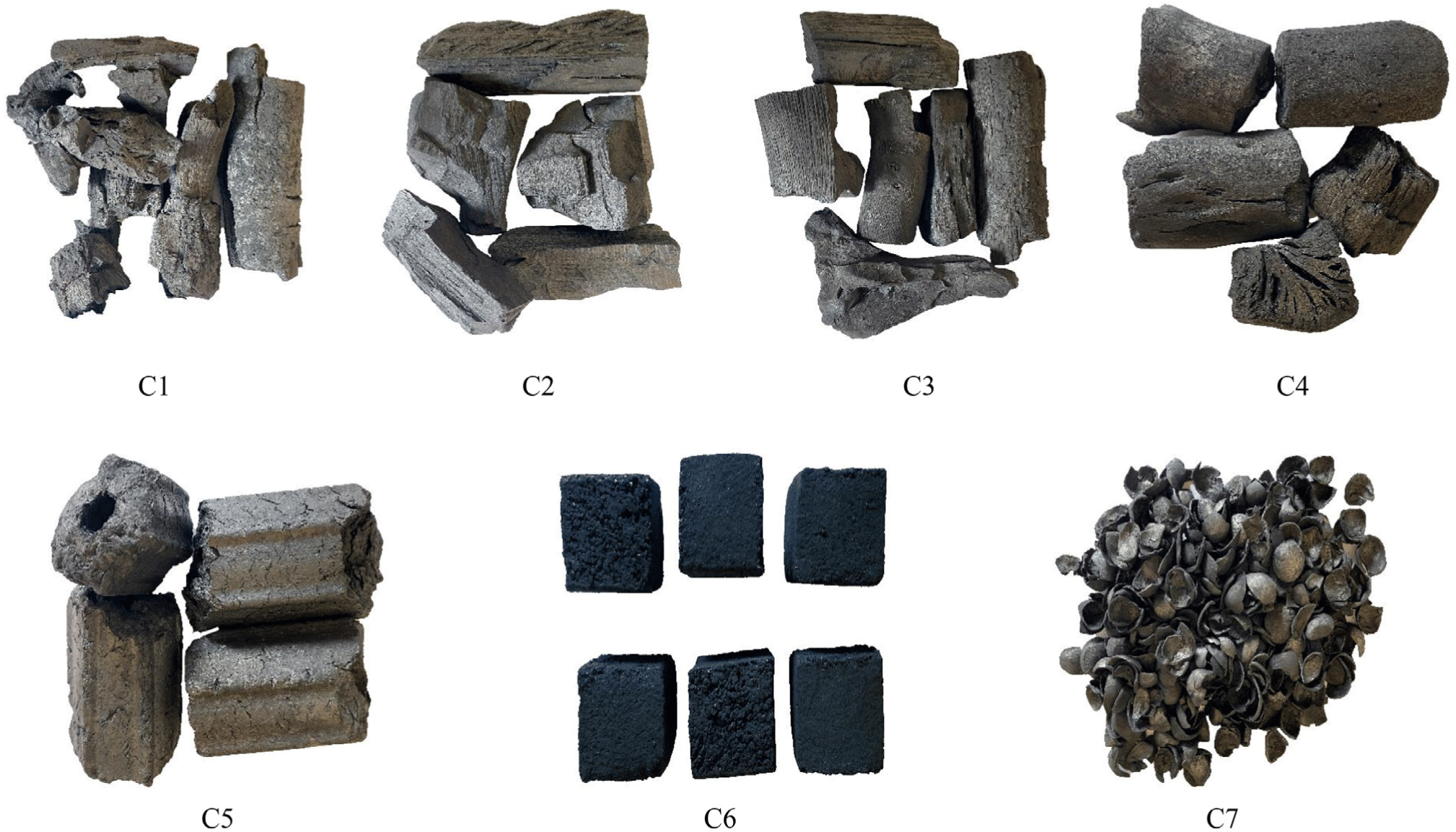
The Longissimus dorsi was cut into steaks of approximate dimensions 4 cm width×13 cm length×0.75 cm thickness, corresponding to a weight of 66.0±3.5 g. A total of 42 beef steaks were obtained.
The beef (B. femoris) and beef fat were minced twice using a 3-mm plate grinder. The amount of beef fat used was adjusted to 14% fat in the minced meat. The other ingredients (1.0% salt and 4.0% water) were added and then mixed for 5 min. This patty dough was stored for 3 hours at 4°C and formed into patties in a petri dish (40 g per patty) to obtain an average size (55 mm diameter and 10 mm thickness). A total of 42 beef patties were prepared. The prepared beef steaks and beef patties were randomly divided into seven groups for grilling with different charcoals.
A grill (25 cm width, 40 cm length, and 9 cm height; Konya, Türkiye) was used to cook the samples. About 0.75 kg of each charcoal (C1, C2, C3, C4, C5, C6, and C7) was spread on the grill for balanced heating. After the charcoals had turned to embers, the samples were grilled. The distance between the samples and the heat source was 7 cm. The samples were turned once during grilling halfway through the total cooking time. The internal temperature reached at least 74°C in all groups. The internal temperature of the samples was measured with a thermometer (Digitale Bratengabel, TCM, Hamburg, Germany). The grilling times with C1, C2, C3, C4, C5, C6, and C7 were 6 min for the beef patties and 7 min for the beef steaks until well cooked. The samples were not treated with oil before or after grilling. Samples cooled to room temperature were ground with a blender before analysis.
The water, total fat, total protein, and total ash content of beef steak and beef patty samples were determined according to AOAC (2000). The water content was determined by oven drying at 105°C. The total fat content of the samples was quantified with a Soxhlet extraction and petroleum ether was used for extraction. The total protein content of the samples was determined using the Kjeldahl method, in which the determination of the total amount of nitrogen (N) is followed by a conversion to the protein content using the conversion factor (6.25). The total ash content of the samples was determined after combustion in a furnace at 550°C. The water, total fat, total protein, and total ash content of the samples was expressed as a percentage (%).
Extraction and purification procedures were carried out based on the modified method reported by Kılıç Büyükkurt et al. (2020). A homogenised sample of 25 g was weighed into a flask. For saponification, 12 g of potassium hydroxide and 100 mL of ethanol were added to the flask. Then 5 mL of the internal standard anthracene-D10 (20.5 ppm) and 1 mL PAH mix (500 ng/kg) were added. The mixture was kept at 40°C for 2 hours under a reflux condenser. After filtration of the solution, it was cooled to room temperature. It was then transferred to a separating funnel where 100 mL of water and 100 mL of cyclohexane were added. The ethanol/water phase was discarded. The cyclohexane phase was rinsed with the mixture of 50 mL N, N-Dimethylformamide and water (9:1). Then 50 mL NaCl solution (1%) was added and rinsed again. After the phase separation was formed in the solution, the cyclohexane phase (5 mL) was transferred into the quick, easy, cheap, effective, rugged, and safe (QuEChERS) dispersive solid phase extraction (dSPE) tubes with magnesium sulphate and primary secondary amine as sorbent, and the purification procedure was started. The tubes (QuEChERS dSPE) were shaken manually for 1 min and then it was centrifuged (at 2,750×g for 5 min). After centrifugation, the upper phase (1 mL) was transferred to vials.
For the determination of PAHs in the samples, the method developed by Sik et al. (2016) for the analysis of GC-MS was used. Samples prepared in vials were injected into the GC system (Trace GC Ultra, Thermo Scientific, Waltham, MA, USA) equipped with an ISQ single-quadrupole mass spectrometer (Thermo Scientific). PAHs were separated with a Thermo Scientific HP-5MS column (15 m×0.25 mm×0.25 μm). The carrier gas (helium) had a constant flow rate of 1 mL/min. The oven temperature was first set to 50°C and held for 0.1 min. Then the temperature was increased to 180°C at 20°C/min and held for 1 min. Then the temperature was raised to 250°C at 20°C/min and held for 1 min, and finally it was raised to 300°C at 20°C/min, which was held for 3.5 min. The temperatures of the injector and detector were set to 280°C. The injection volume was 1 μL and the samples were injected in splitless mode.
The PAH concentration in the samples was calculated using a calibration curve method with different concentrations of standards (Taverniers et al., 2004). The PAH analytical method was validated with respect to the limits of detection (LOD), the limits of quantification (LOQ), the linear equation, the coefficient of the regression line (r2) and the recovery rate. The LODs, LOQs, linear equations, regression line coefficient (r2) and recoveries of PAH standards are given in Table 2. Five replicates of the spiked samples were prepared for the determination of recovery rates. The contents of the PAH compounds in the samples were given as μg/kg wet weight of sample.
Two independent replicates were conducted using a completely randomised factorial design with seven treatments (C1, C2, C3, C4, C5, C6, and C7) and two meat types (beef steak and beef patty). For the statistical analysis of the levels of PAHs, an analysis of variance was performed with the general linear model using Minitab 16.0 with one-way assignment. The different charcoal and meat types were analysed as a fixed factor, while the replicate was considered as a random factor. Significant differences between groups were further analysed using Tukey’s Multiple Range Test at 5% significance level.
After the Kaiser-Meyer-Olkin (KMO) value was determined using SPSS version 29 (IBM, Armonk, NY, USA), a principal component analysis (PCA) was performed to show and clarify the relationship between the charcoal types, meat products and analytical parameters (PAHs) using JMP software (JMP Pro 16.0, JMP, Cary, NC, USA).
Results and Discussion
The water, total protein, total fat, and total ash contents of the beef steak and beef patty samples are given in Table 3. The beef steak had the highest water and total protein content (p<0.05), while the beef patty had the highest total fat content (p<0.05). This is due to the fact that the beef fat was added to the formulation in the production of beef patty. The difference between the total ash content of beef steak and beef patty was insignificant (p>0.05). Nutrients in meat products such as fats and proteins are cracked under high-temperature conditions of 200°C and above to generate light rings with low molecular weight and intermediate free radicals with high reactivity. The two are then cyclised, leading to the formation of PAHs (Singh et al., 2020). On the other hand, PAHs have also been reported to be produced by the smoke formed when the fats in meat products drip onto the heat source (Alomirah et al., 2011).
Meat types | Water (%) | Total protein (%) | Total fat (%) | Total ash (%) |
---|---|---|---|---|
Beef steak | 64.09±0.09A | 19.93±0.08A | 13.48±0.16B | 2.20±0.02A |
Beef patty | 62.15±0.22B | 17.57±0.17B | 17.82±0.42A | 2.27±0.03A |
Thermal processing is the most important factor influencing the formation of PAHs in meat and meat products. Conventional meat processing methods that promote PAHs include heating, frying, toasting, grilling, baking, smoking, drying, and roasting (Iwegbue et al., 2019). Grilling involves placing an intermediate product over a heat source, usually charcoal, and exposing it to a high temperature (Park et al., 2017). The PAH content of grilled meat and meat products depends on several processing parameters, such as the construction of the grill, the type of heat source, the temperature and the type of meat (Duedahl-Olesen and Ionas, 2022).
PAHs in beef steaks grilled with different charcoals are given Table 4. Naphthalene, benzo[a]anthracene, chrysene, benzo[b]fluoranthene, benzo[k]fluoranthene, benzo[a]pyrene, indeno[1,2,3-cd]pyrene, and dibenzo[a,h]anthracene were not detected in all beef steak samples. Different charcoals did not affect the fluoranthene and benzo[ghi]perylene levels of beef steaks (p>0.05). Acenaphthylene (4.08 μg/kg) was only determined in beef steaks grilled with C2 (orange wood charcoal) and anthracene (2.25 μg/kg) only in beef steaks grilled with C7 (hazelnut shell charcoal; p<0.05). Beef steaks grilled with hazelnut shell charcoal (C7; 5.15 μg/kg) had a higher acenaphthene content than samples grilled with C3 (3.57 μg/kg) and C6 (3.65 μg/kg; p<0.05). C1 and C7 had the highest content of phenanthrene in beef steaks (p<0.05). The sum of PAH4 was not determined in any of the grilled beef steaks due to absence of benzo[a]anthracene, chrysene, benzo[b]fluoranthene and benzo[a]pyrene. The total concentration of the 16 PAHs (PAH16) in beef steaks grilled using oak wood charcoal (C1; 35.75 μg/kg) and hazelnut shell charcoal (C7; 36.39 μg/kg) was higher than that of C3 (23.80 μg/kg) and C6 (24.48 μg/kg; p<0.05). It has been reported that the type and shape of the heat source when grilling with charcoal has an influence on the PAH content in grilled foods (Oz and Yuzer, 2016). The seven different charcoals used in our study were obtained from different sources (e.g., wood, organic material) and had different manufacturing processes. Especially in the production of charcoal, the carbonization temperature has an important influence on the volatile components of the charcoal. When the carbonization temperature is high, the volatile content of the charcoal is low. If the carbonization temperature is low, the amount of volatile compounds in the charcoal is high. High levels of the volatile compounds can produce various PAHs during grilling (Prathomtong et al., 2016). This could be related to the fact that the C1 (oak wood charcoal) and C7 (hazelnut shell charcoal) in our study caused a higher PAH content in the beef steaks. Güler and Aydın Temel (2023) reported that hazelnut shell charcoal had higher volatile matter than wood waste charcoal.
Type of meat product | PAH compounds (μg/kg wet weight) | Different charcoals | ||||||
---|---|---|---|---|---|---|---|---|
C1 | C2 | C3 | C4 | C5 | C6 | C7 | ||
Beef steak samples | Na | NDAa | NDAa | NDAa | NDAa | NDAb | NDAa | NDAa |
Ac | NDBb | 4.08±0.80Aa | NDBb | NDBb | NDBb | NDBb | NDBb | |
Ace | 5.00 ±0.16ABb | 4.34 ±0.25ABb | 3.57 ±0.30Bb | 3.66 ±0.31ABb | 3.77 ±0.19ABb | 3.65 ±0.33Bb | 5.15 ±0.63AB[6] | |
F | 2.30 ±0.31ABb | 2.72 ±0.29AB[6] | 1.38 ±0.10Bb | 1.65 ±0.17ABb | 1.45 ±0.08Bb | 1.52 ±0.11Bb | 2.29 ±0.28ABb | |
Pa | 19.99 ±1.02AB[6] | 10.72 ±0.73Cb | 11.91 ±0.82Cb | 12.43 ±0.84Cb | 13.29 ±1.04BCb | 12.02 ±0.71Cb | 18.46 ±1.47ABb | |
A | NDBb | NDBb | NDBb | NDBb | NDBb | NDBb | 2.25 ±0.25Ab | |
Fl | 3.17 ±0.68ABb | 1.83 ±0.22ABb | 2.18 ±0.53ABb | 2.78 ±0.31ABb | 2.67 ±0.17ABb | 2.30 ±0.40ABb | 2.87 ±0.37ABb | |
P | 2.43 ±0.23ABb | 1.73 ±0.13Bb | 2.00 ±0.03ABb | 2.76 ±0.21ABb | 2.02 ±0.15ABb | 1.94 ±0.17ABb | 2.53 ±0.15ABb | |
BaA | NDAa | NDAa | NDAa | NDAa | NDABb | NDAa | NDAa | |
Ch | NDAa | NDAa | NDAa | NDAa | NDABb | NDAa | NDAa | |
BbF | NDAa | NDABb | NDAa | NDAa | NDABb | NDAa | NDAa | |
BkF | NDAa | NDAa | NDAa | NDAa | NDABb | NDAa | NDAa | |
BaP | NDAa | NDAa | NDAa | NDAa | NDABb | NDAa | NDAa | |
IP | NDAa | NDABb | NDAa | NDAa | NDABb | NDAa | NDAa | |
DhA | NDAa | NDAa | NDAa | NDAa | NDAa | NDAa | NDAa | |
BgP | 2.87 ±0.10Aa | 2.76 ±0.01ABb | 2.77 ±0.01Aa | 3.04 ±0.19Aa | 2.75 ±0.01ABb | 3.06 ±0.07Aa | 2.86 ±0.05Aa | |
PAH4 | NDAa | NDABb | NDAa | NDAa | NDABb | NDAa | NDAa | |
PAH16 | 35.75 ±2.49ABb | 27.50 ±2.21ABb | 23.80 ±1.73Bb | 26.31 ±1.95ABb | 25.93 ±1.56ABb | 24.48 ±0.81Bb | 36.39 ±3.00ABb | |
Beef patty samples | Na | NDBa | NDBa | NDBa | NDBa | 6.36 ±0.35Aa | NDBa | NDBa |
Ac | 2.14 ±0.17Ba | 4.86 ±1.47Ba | 3.35 ±0.85Ba | 2.81 ±0.35Ba | 14.48 ±3.20Aa | 2.40 ±0.16Ba | 2.81 ±0.41Ba | |
Ace | 6.46 ±0.22CDa | 7.04 ±0.39CDa | 8.37 ±0.53BCa | 5.75 ±0.58Da | 10.57 ±0.09Aa | 7.96 ±0.38BCa | 9.92 ±0.19ABa | |
F | 4.29 ±0.42Ba | 4.52 ±0.43Ba | 4.75 ±0.01Ba | 5.47 ±0.01Ba | 11.49 ±1.31Aa | 5.33 ±0.21Ba | 7.68 ±1.29ABa | |
Pa | 31.44 ±5.68Ba | 34.76 ±5.56Ba | 36.40 ±0.79Ba | 29.57 ±4.64Ba | 77.58 ±7.39Aa | 32.80 ±0.96Ba | 42.15 ±7.63Ba | |
A | 4.03 ±0.41Ba | 5.10 ±1.01Ba | 4.66 ±0.02Ba | 4.29 ±0.20Ba | 11.90 ±1.17Aa | 4.01 ±0.01Ba | 6.07 ±0.97Ba | |
Fl | 5.73 ±1.08Ba | 8.15 ±1.79Ba | 6.48 ±0.01Ba | 5 13 ±1.09Ba | 20.42 ±2.61Aa | 5.69 ±0.45Ba | 5.61 ±0.84Ba | |
P | 5.47 ±0.46Ba | 9.56 ±2.07Ba | 6.82 ±0.09Ba | 5.64 ±0.95Ba | 22.19 ±5.08Aa | 7.13 ±0.95Ba | 7.62 ±1.16Ba | |
BaA | NDBa | NDBa | NDBa | NDBa | 6.60 ±1.12Aa | NDBa | NDBa | |
Ch | NDBa | NDBa | NDBa | NDBa | 5.40 ±0.39Aa | NDBa | NDBa | |
BbF | NDCa | 3.83 ±0.54Ba | NDCa | NDCa | 5.79 ±1.63Aa | NDCa | NDCa | |
BkF | NDBa | NDBa | NDBa | NDBa | 5.59 ±0.67Aa | NDBa | NDBa | |
BaP | NDBa | 3.89 ±0.39ABa | NDBa | NDBa | 5.17 ±1.10Aa | NDBa | NDBa | |
IP | NDCa | 4.62 ±0.52Ba | NDCa | NDCa | 6.35 ±0.64Aa | NDCa | NDCa | |
DhA | NDAa | NDAa | NDAa | NDAa | NDAa | NDAa | NDAa | |
BgP | 2.99 ±0.11Ba | 3.86 ±0.72ABa | 2.91 ±0.11Ba | 3.11 ±0.03Ba | 6.54 ±1.28Aa | 3.06 ±0.06Ba | 2.87 ±0.03Ba | |
PAH4 | NDCa | 7.72 ±0.93Ba | NDCa | NDCa | 22.95 ±3.25Aa | NDCa | NDCa | |
PAH16 | 62.89 ±6.34Ba | 90.18 ±15.12Ba | 74.74 ±0.31Ba | 61.75 ±7.07Ba | 216.40 ±28.85Aa | 69.38 ±2.45Ba | 85.74 ±9.56Ba |
C1: Oak wood charcoal, C2: Orange wood charcoal, C3: Valonia oak wood charcoal, C4: Marabu wood charcoal, C5: Extruded charcoal from beech wood, C6: Coconut shell.
A–D Different superscript capital letters within the different charcoals in the same type of meat products indicate significant differences (p<0.05).
a,b Different superscript lowercase letters within the type of meat products in the same type of charcoal indicate significant differences (p<0.05).
PAH, polycyclic aromatic hydrocarbon; Na, naphthalene; ND, not detected; Ac, acenaphthylene; Ace, acenaphthylene; F, fluorene; Pa, phenanthrene; A, anthracene; Fl, fluoranthene; P, pyrene; BaA, benzo[a]anthracene; Ch, chrysene; BbF, benzo[b]fluoranthene; BkF, benzo[k]fluoranthene; BaP, benzo[a]pyrene; IP, indeno[1,2,3-cd]pyrene; DhA, dibenzo[a,h]anthracene; BgP, benzo[ghi]perylene; PAH4, BaA+Ch+BbF+BaP; PAH16, total 16 PAHs.
The amounts of PAH components formed in the beef patties grilled with different coals are listed in Table 4. Of the 16 PAH compounds, only dibenzo[a,h]anthracene was not detected in any of the beef patty samples. Naphthalene (6.36 μg/kg), benzo[a]anthracene (6.60 μg/kg), chrysene (5.40 μg/kg), and benzo[k]fluoranthene (5.59 μg/kg) were only determined in beef patties grilled with extruded charcoal (C5; p<0.05). The beef patties grilled with charcoals C5 (10.57 μg/kg) and C7 (9.92 μg/kg) had the highest acenaphthene content (p<0.05). With regard to acenaphthylene, phenanthrene, anthracene, fluoranthene, pyrene, benzo[b]fluoranthene, benzo[a]pyrene, and indeno[1,2,3-cd]pyrene, the beef patties grilled with extruded charcoal (C5) had higher levels than the other beef patty groups (p<0.05). Therefore, the highest amounts of PAH4 (22.95 μg/kg) and PAH16 (216.40 μg/kg) were determined in the beef patty samples grilled with C5 (p<0.05). The differences in the sum of PAH16 levels for groups other than C5 were insignificant (p>0.05). It has been reported that extruded charcoal is carbonized at a lower temperature (600°C–800°C) and contains ignition chemicals such as barium nitrate (Prathomtong et al., 2016). Therefore, this could be the reason why our PAH16 content was highest in the beef patties grilled with extruded charcoal. Similar results were observed in grilled samples of pork belly, beef loin and chicken thighs with extruded charcoal, which contained more PAH compounds than those from black and white charcoal (Kim et al., 2021).
The effects of the type of meat products on the PAH content in the samples of grilled beef steak and beef patties are shown in Table 4. The sum of PAH4 was not detected in any of the beef steaks, whereas it was determined in the beef patties grilled with C2 (7.72 μg/kg) and C5 (22.95 μg/kg; p<0.05). The PAH16 concentrations of the beef patty samples in each charcoal group were significantly higher compared to beef steaks (p<0.05). It is assumed that the higher fat content (Table 3) of beef patties is the reason for the higher PAH content of beef patties compared to beef steaks. Saito et al. (2014) and Wang et al. (2018) reported that differences in PAH levels formed when grilling meat products over charcoal could be due to the amount of fat contained in the meat product. This is because the fat in the food drips onto the heat source during grilling and burns, producing smoke. The PAHs are deposited on the surface of the meats by the smoke (Kim et al., 2021; Saito et al., 2014; Wang et al., 2018). Similarly, Kim et al. (2021) determined that pork belly, which had a higher fat content compared to beef loin and chicken thigh, was the group with the highest 16 PAH level as a result of grilling with extruded charcoal. Viegas et al. (2012) also attributed the higher PAH contents of the salmon samples grilled with coconut and wood charcoal to the higher fat content of the salmon samples. In addition, it has been noted that a high water content in meat and meat products can reduce the formation of PAH by inhibiting the temperature rise during cooking (Onopiuk et al., 2021). The fact that the water content of beef steaks in the present study is higher than that of beef patties (Table 3) supports the lower PAH content of beef steaks compared to beef patties. On the other hand, in this study C1 and C7 resulted in higher PAH content in beef steaks (p<0.05), while C5 resulted in the highest PAH content in grilled beef patties (p<0.05). This contradictory situation may be attributed to the type of the meat product and, in particular, to the complex formed by the dripping of the fat contained in the meat onto the charcoal. It has been pointed out that charcoal can produce light PAHs when meat products are grilled with charcoal. During grilling, the melted fat of the meat product dripping onto the charcoal can produce heavy PAHs (García-Lomillo et al., 2017). In our study, it was determined that more heavy PAHs were formed in the beef patty samples grilled with C5 than in the other groups of beef patties (Table 4) and beef steaks grilled with seven different charcoals (Table 4).
The KMO is a statistic that measures the adequacy of samples. It has been reported that a KMO value of more than 0.5 can be used for factor analysis, while a KMO value of more than 0.8 is very appropriate for factor analysis (Li et al., 2020). In the present study, the KMO value was calculated as 0.828, and the significance level was determined as p<0.001.
The PCA results are given in Table 5 and the PCA plots of the different charcoals (C1, C2, C3, C4, C5, C6, and C7) and meat products (B and M) are shown in Fig. 2. The first principal component (PC1) and the second principal component (PC2) were 86.04% and 8.81% respectively. The fluoranthene (0.991), pyrene (0.983), PAH16 (0.980), benzo[ghi]perylene (0.976) and acenaphthylene (0.968) were the most contributed factors for PC1. PC2 was identified with acenaphthene (0.656; Table 5). The beef steak samples (C1-B, C2-B, C3-B, C4-B, C5-B, C6-B, and C7-B) were located in the negative area of PC1 and PC2. The samples of C2-M, C5-M, and C7-M were located in the positive are of PC1 and C1-M, C3-M, C4-M, and C6-M were in the negative area of PC1. Additionally, C1-M, C3-M, C4-M, C6-M, and C7-M were in the positive area of PC2, while C2-M and C5-M were in the negative area of PC2 (Fig. 2). As shown in Fig. 2, the C5-M group contains most of the 16 PAH compounds.
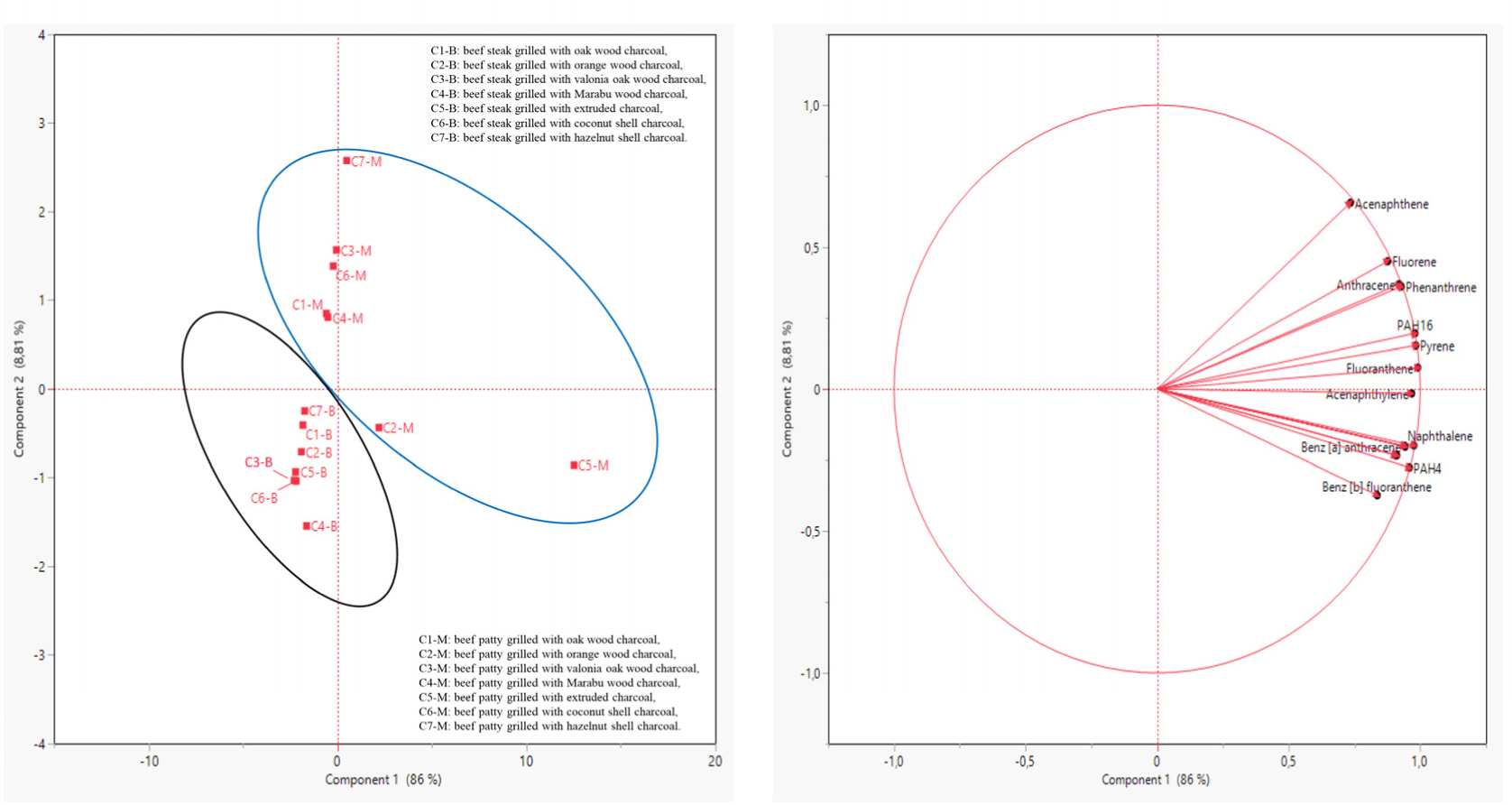
Conclusion
The present study investigated the effects of seven different charcoals on the formation of PAHs in grilled beef steaks and beef patties. The levels of the PAHs were influenced by the type of charcoal and type of meat product. Oak wood charcoal (C1) and hazelnut shell charcoal (C7) caused a higher PAH16 content in the beef steaks. The highest levels of PAH4 and PAH16 were determined in the beef patty samples grilled with extruded charcoal (C5). The PAH16 concentrations of the beef patty samples in each charcoal group were very high compared to beef steaks, which can be attributed to the higher fat content of the beef patties. It could be suggested to choose meat products with a low-fat content and to avoid the use of extruded charcoal and hazelnut shell charcoal to reduce the formation of PAHs during grilling.