Introduction
Food additives are intentionally added to foods during manufacturing and processing to preserve their flavor or improve their taste, appearance, or other qualities (Yu et al., 2020). Unsaturated fatty acids and high protein concentrations in foods, particularly meat products, are exposed to light during storage, which oxidizes lipids and proteins (Yong et al., 2021). To prevent this, various food additives are used in sausage manufacturing (Lee et al., 2020; Yong et al., 2020). Synthetic additives are used effectively in meat products owing to their low cost, high stability, and high efficiency (Alirezalu et al., 2017). Chemical preservatives and antioxidants, including butylated hydroxyanisole and butylated hydroxytoluene (BHT), reduce lipid oxidation and enhance antibacterial activity, extending the product’s shelf life (Lee and Paik, 2016). However, some chemical preservatives are carcinogenic and teratogenic; therefore, their use is restricted in each country.
Sorbate has received global regulatory approval for use as an antibacterial preservative in food, animal feed, pharmaceuticals, and cosmetics (Stopforth et al., 2005). Sorbate is effective against many bacteria, molds, and yeasts and has primarily been used as an antifungal agent in food (Robach and Sofos, 1982). It is a weak acid (pKa=4.76) in its undissociated form and shows maximum antibacterial activity at low pH. Therefore, it is consistently effective as an antimicrobial agent in foods with pH<5.0–5.5 (Robach and Sofos, 1982). Potassium sorbate or sorbic acid (SOR) slows the growth and toxin production of the spoilage microorganisms Clostridium in products such as cooked and cured red meat and poultry sausages (Robach and Sofos, 1982). It also extends the shelf life of several meat products, including bacon, and retards the growth of other pathogenic and spoilage microorganisms (Liewen and Marth, 1985).
Despite sorbates being registered as GRAS (Generally Recognized as Safe), consumers express concerns about their use because sorbates fall into the category of chemical preservatives. Therefore, the development of natural preservatives which can replace chemical preservatives is required to address consumer and market demands for clean-label foods. The shelf life of meat products is primarily determined by microbial spoilage and lipid peroxidation (Lee et al., 2013). In general, fruits and vegetables contain various phytonutrients with antioxidant properties (Ehlenfeldt and Prior, 2001). Researchers continue to utilize fruit and vegetable extracts since the antioxidant properties effectively minimize or prevent lipid oxidation in foods, delaying the formation of toxic oxidation products, and extending their shelf life (Aziz and Karboune, 2018).
Grapefruit seed extract (GFS) has been reported to exhibit highly effective antibacterial activity when applied directly to food (Reagor et al., 2002). It can prevent the growth of foodborne pathogens present in various fruit, vegetable, meat and fish products, and this effect is thought to be caused by quaternary ammonium compounds (Kim et al., 2021). Natural antioxidants, including grapeseed and green tea extracts, used to improve the quality of various meat products, have been evaluated on raw beef patties and cooked pork meatballs and successfully retard microbial growth in meat products (Bañón et al., 2007). Among the components of berry extract, phenolic acids inhibit the growth of gram-negative bacteria. In particular, ellagitannin, a common ingredient in cloudberry, raspberry, and strawberry extracts, has a strong inhibitory effect on Salmonella (Puupponen-Pimiä et al., 2001). Porcine and bovine ground meat stored in the raw and cooked state, show reduced lipid oxidation in the presence of oregano and sage essential oils (EO; 3% w/w) during storage at 4°C for 12 d. Oregano EO consists of 20 ingredients, including thymol, p-cymene, γ-terpinene and carvacrol, and sage EO consists of 37 visible ingredients, including eucalyptol, camphor and α-pinene (Fasseas et al., 2008). Rosemary extract (main active ingredient is carnosic acid) was evaluated in various meat products, including chicken Frankfurt sausages, turkey products, and cooked ground beef. All samples showed lower thiobarbituric acid reactive substances (TBARS), a lipid oxidation values, indicating better oxidative stability than those of the control samples (Ahn et al., 2007; Rižnar et al., 2006; Yu et al., 2002). In summary, these studies provide sufficient evidence for the use of natural antimicrobials as alternatives to chemical antimicrobials.
Psidium guajava is widely distributed in subtropical climates, and guava leaves possess higher antioxidant activity than that of its fruits (Lestari et al., 2022). Several functional ingredients such as terpenoid flavonoids, tannins, and quercetin are present in P. guajava leaves (Biswas et al., 2013). Ecklonia cava contains various compounds, including carotenoids, fucoidans, and phlorotannins, and has various physiological functions, including antioxidant, anticancer, and antihypertensive properties (Wijesinghe and Jeon, 2012). Paeonia japonica (Makino) Miyabe & Takeda is used as a medicinal plant and is valuable as a functional food, owing to its antioxidant and antibacterial activities. Although many natural extracts have been studied primarily for their physiological functions, research on their antibacterial effects remains insufficient.
Therefore, the purpose of this study is to determine the optimal mixing ratio of extracts (P. guajava, E. cava, and P. japonica (Makino) Miyabe & Takeda), investigate the antibacterial activity against pathogenic bacteria, and prepare a natural extract mixture that can be universally used against various types of pathogenic bacteria. And then, we aimed to determine its potential as a potential natural preservative for meat products by applying the natural extract mixture to sausages and investigating its effect on microbial growth and quality of the products during refrigerated storage.
Materials and Methods
The plants used in this study were P. guajava (Yeongcheon, Korea), E. cava (Yeongcheon, Korea), P. japonica (Makino) Miyabe & Takeda (Jecheon, Korea), which were procured from the domestic market. Each sample was pulverized using a grinder (Cgoldenwall, Zhejiang, China), mixed with 50% (v/v) ethanol at a ratio of 1:10, and stirred (120 rpm) at room temperature for 24 h. Supernatants of the extracts were separated using centrifugation (1,519×g, 5 min), and impurities were removed using Whatman filter paper. Finally, the solvent was removed under reduced pressure using a rotary evaporator (Eyela N-3000, Shanghai Eyela, Shanghai, China), lyophilized, and stored in a quick freezer at –70°C. The stored extract was prepared at a concentration of 160 mg/mL by dissolving in tryptic soy broth (TSB, Sparks, Philadelphia, PA, USA) containing 20% dimethyl sulfoxide.
Antibacterial activity was determined against three bacterial species, Listeria monocytogenes (gram-positive), Salmonella spp. (gram-negative), and Escherichia coli (gram-negative), which are associated with foodborne illnesses in meat products. L. monocytogenes strains (NCCP 10920, NCCP 10943, ATCC 13932, ATCC 51774, and ATCC BAA 839) were activated in 10 mL TSB containing 0.6% yeast extract (TSBYE) and incubated at 30°C for 24 h. Salmonella spp. (Enteritidis NCCP 14645, Typhimurium NCCP 12219, Typhimurium NCCP 16207, Montevideo NCCP 10140, Kentucky NCCP 11686) and E. coli (NCCP 13717, NCCP 13718, NCCP 13719, NCCP 13720, and NCCP 13721) were activated in 10 mL TSB and incubated at 37°C for 24 h.
Aliquots of bacterial cultures were sub-cultured in the same medium under the same conditions. The cultures were then centrifuged (1,912×g, 15 min) and washed twice with 0.85% sterile saline (Cleancer; JW Pharmaceutical, Dangjin, Korea). A mixture of the same strains was used as inoculum for the experiments.
The minimum inhibitory concentrations (MIC), defined as the lowest concentration of natural extracts and mixtures with no visible growth, were determined using the serial dilution method. Here, samples were 2-fold serially diluted and 90 μL aliquots of each sample was placed in individual wells of a 96-well microplate using TSB for E. coli and Salmonella spp. or TSBYE for L. monocytogenes. Then, the bacterial samples were inoculated in each well at a concentration of 6–7 Log colony forming units (CFU)/mL and incubated for 24 h at 37°C (TSB) or 30°C (TSBYE). Microbial growth was evaluated by measuring the turbidity of each well at 600 nm using a microplate reader (BioTek, Winooski, VT, USA).
The minimum bactericidal concentrations (MBC) of the plant extracts and mixtures were determined based on bacterial growth by streaking the samples on agar plates. After analyzing the MIC of the 96-well microplates, the contents of all microplates were streaked on TSA or TSAYE and incubated at 37°C or 30°C, respectively, for 24 h. The lowest concentration in the plate with no growth was considered as the MBC. In both MIC and MBC experiments, SOR and GFS were used as controls.
Design Expert software 7.0 (Stat-Ease, Minneapolis, MN, USA) was used to determine the optimum ratio of natural extracts, and D-optimal design was used as the mixture design. The independent variables selected were E. cava (A), P. guajava (B), and P. japonica (Makino) Miyabe & Takeda (C), and MIC and MBC were set as the dependent variables. The total concentrations of P. guajava, E. cava, and P. japonica (Makino) Miyabe & Takeda were set to 160 mg/mL, and the minimum and maximum ranges were set through preliminary experiments as follows: 2.34 mg/mL<E. cava<150 mg/mL, 2.34 mg/mL<P. guajava<150 mg/mL, 4.68 mg/mL<P. japonica (Makino) Miyabe & Takeda<150 mg/mL. The mixing ratios of the materials for each setting range are listed in Table 1. Six repetition points were observed among the fourteen experimental points.
The optimization method is suitable for increasing the process efficiency and does not require an increase in cost. In this study, the material mixing ratio was optimized through numerical optimization of the standard model and model optimization of the mixing components (Park and Kim, 2017). The goals of the experimental items were minimized and in range, and the optimization was determined using a multiple-response method called desirability.
The effects of inhibitory concentrations of the natural extract mixtures on the growth of E. coli, Salmonella spp., and L. monocytogenes within 2 d were evaluated. Concentrations of the natural extract mixtures used were 0.2% (F0.2), 0.5% (F0.5), and 1% (F1.0); TSB (CON) was used as a negative control, and SOR and GFS were used as positive controls. Fifty microliters of the solutions (at different concentrations) were divided into bottles to which, 500 μL of the bacterial suspension was added (maximum bacterial concentration of 6–7 Log CFU/mL) and incubated at 20°C. The dilutions of interest were made on 0, 1, and 2-day and cultured in TSA or TSAYE at 37°C or 30°C, respectively, for 24 h. Following this, colony count and bacteria number (Log CFU/mL) were determined.
Fresh pork ham muscles and pork back fat were ground using a chopper (3 mm plate). The sausages were formulated as described by Lee et al. (2021), using ground pork ham (50%), pork back fat (25%), and ice water (25%). Ground pork was homogenized using a silent cutter; salt (1.5%) and phosphate (0.3%) were then added. Sausage batter was prepared by adding fat (25%) and ice (25%); The natural extracts were then added and combined using a silent cutter. Following this, the batter was filled with a collagen casing (approximate diameter: 25 mm). The control contained no preservatives, while T1 and T2 contained 0.2% SOR and 0.2% GFS, respectively. The natural extracts were treated as T3 (0.2% natural extract), T4 (0.2% natural extract+0.01% ascorbic acid), T5 (0.5% natural extract), and T6 (0.5% natural extract+0.01% ascorbic acid). Cooking was performed at 85°C for 30 min in a smoke chamber (MAXi3501 Chamber, Kerres, Postfach, Germany). Each portion of the sausage was vacuum packed as described previously and used for storage analysis after 0–28 d (Woo et al., 2023).
The pH values of the sausages containing the natural extract mixtures were determined in a homogenate prepared with sausage sample (5 g) and DW (20 mL) using a pH meter (Mettler-Toledo, Schwerzenbach, Switzerland).
CIE (Commission internationale de l’Eclairage) L*a*b* color analysis was conducted on sausages containing a mixture of natural extracts. The CIE L*, CIE a*, and CIE b* values of the sausages containing the natural extract mixtures were determined using a CR-410 colorimeter (Minolta, Tokyo, Japan) calibrated with a white plate (Illuminate C Observer 2°).
Lipid oxidation in sausages containing the natural extract mixtures was determined using the TBARS method, described by Tarladgis et al. (1960). Sausages (10 g) were blended with distilled water (50 mL) and 0.3% BHT (200 μL) at 10,000 rpm for 60 s. Distilled water (47.5 mL), 4 N HCl (2.5 mL), and an antifoam agent (1 mL) were added to the flask. The distilled mixture (5 mL) and 0.02 M 2-thiobarbituric acid (5 mL) were added to a test tube. The sample solutions were mixed and heated in a water bath at 100°C for 30 min. Absorbance was measured at 538 nm using a UV/Vis spectrophotometer. TBARS value corresponding to the malonaldehyde content was calculated using the formula of a previous research (Tarladgis et al., 1960), and it expressed as mg per kg of meat.
Microbiological analysis was conducted at 1, 14, 21, and 28 d during storage at 4°C. The samples were suspended in sterile saline (0.85%) and homogenized in a stomacher (MiniMix® 100, Interscience, Saint-Nom-la-Bretèche, France) for 1 min. Aliquots of the homogenates were serially diluted, and 1 mL of each dilution was dispensed into a 3M Petrifilm Plate (3M, St. Paul, MN, USA) for total plate counts (TPC), coliform, and E. coli. The plates for TPC were incubated for 24–48 h at 37°C. Coliform and E. coli plates were incubated for 24 h at 37°C. Colonies were counted, and the results were expressed as Log CFU/g of the sample.
A total of 69 adults were selected from the Korea Food Research Institute (KFRI, Wanju, Korea). The panelists were aged 20–50 years (37 women and 32 men). Before the evaluation, the samples were boiled at 100°C for 5 min and cooled at room temperature. They were cut into 2 cm-thick slices and placed in plastic cups covered with plastic lids. The samples were coded using 3-digit random numbers and presented according to the Williams–Latin square design. Spring water and unsalted crackers were provided to clean the mouth between different samples. The panelists evaluated the overall sausage samples using a 9-point hedonic scale (from 1 point=“extremely dislike” to 9 point=“extremely like”) and other sensory properties using the RATA (Rate-All-That-Apply) method. Assessors were asked to select all the terms that described the samples and then rate the intensity of the selected terms on (3-point scale). The intensity was evaluated on a 3-point scale with guiding value labels (i.e. 1=“low”, 2=“medium”, 3=“high”). This study was approved by the Institutional Review Board of KFRI (KFRI-2023-05-002-001).
The quantified results are shown as means±SD. One-way and two-way analysis of variance were performed for statistical analyses using the IBM SPSS statistical software (SPSS Ver. 20.0, IBM, Armonk, NY, USA). The significance of variations among the mean values was assessed using Duncan’s multiple range test, with a confidence level of p<0.05. An independent sample t-test (p<0.05) was performed to determine significant differences in the sensory preference scores. A principal component analysis (PCA) biplot was constructed using the SIMCA 17 software (Umetrics, Umea, Sweden).
Results and Discussion
Table 1 lists the mixing ratios of the natural extract blends prepared at the 14 test points. The antibacterial effects of the mixed extracts were confirmed by measuring the MIC and MBC values for the three types of bacteria (E. coli, Salmonella spp., and L. monocytogenes; Table 2). The MIC of the 14 mixed extracts ranged from 2.5–20.0 mg/mL, while the MBC values ranged from 2.5–40.0 mg/mL. The main components of P. guajava, E. cava, and P. japonica (Makino) Miyabe & Takeda extracts are flavonoids, phenols, steroids, tannins, phlorotannins, and eckol. These extracts exhibit antibacterial properties against EM, Salmonella spp. (gram-negative bacteria), Saccharomyces cerevisiae (yeast strain), and Aspergillus niger (fungal strain; Choi et al., 2010; Choi et al., 2014; Das and Goswami, 2019). Mixing the extracts may alter their active ingredients and concentrations, which can affect antibacterial activity (Ouedrhiri et al., 2016). The mixtures of 14 natural extracts showed antibacterial effects against E. coli at both MIC and MBC of 2.5–10.0 mg/mL. For Salmonella spp., the MIC and MBC were 2.5–10 mg/mL and 2.5–20 mg/mL, respectively. For L. monocytogenes, the MIC and MBC were 5–20 mg/mL and 10–40 mg/mL, respectively, showing relatively lower antibacterial activity than those of E. coli and Salmonella spp. The SOR showed higher MBC values than those of the mixed extracts, with MIC and MBC values ranging from 2.5–5.0 mg/mL and 10.0–160.0 mg/mL, respectively. In contrast, grapefruit seeds exhibited antibacterial effects at 0.16–0.31 mg/mL for, both, the MIC and MBC. Grapefruit seeds are known natural antimicrobial agents with strong antibacterial and antifungal properties (Kim et al., 2021).
Fig. 1 shows ternary diagrams (3D images and contour plots) illustrating the interactions between each independent variable used for analyzing antibacterial activity against the three pathogens (E. coli, Salmonella spp., and L. monocytogenes). In the contour plot and 3D image, dark blue areas represent low MIC and MBC values (high antibacterial efficacy), while green to red areas represent medium to high MIC and MBC values (low antibacterial efficacy). The natural extract mixtures prepared according to the mixture design combinations showed stronger antibacterial effects against E. coli and Salmonella spp. (gram-negative bacteria) than that against compared to Gram-positive bacteria, L. monocytogenes. The type of bacteria exhibiting an antibacterial effect may vary depending on the type of compound included in the natural extract mixture and the reaction expression method (Chakraborty et al., 2007; Mau et al., 2001). According to Table 2 and Fig. 1, the antibacterial effect against pathogens in the central mixture design was found to weaken as the ratio of white peony increased. Consequently, a blend containing 49% E. cava, 48% P. guajava, and 3% P. japonica (Makino) Miyabe & Takeda exhibited the lowest MIC (2.5–5.0 mg/mL) and MBC (5.0–10 mg/mL) against all tested pathogens, thus representing the highest antibacterial activity against the tested pathogens.
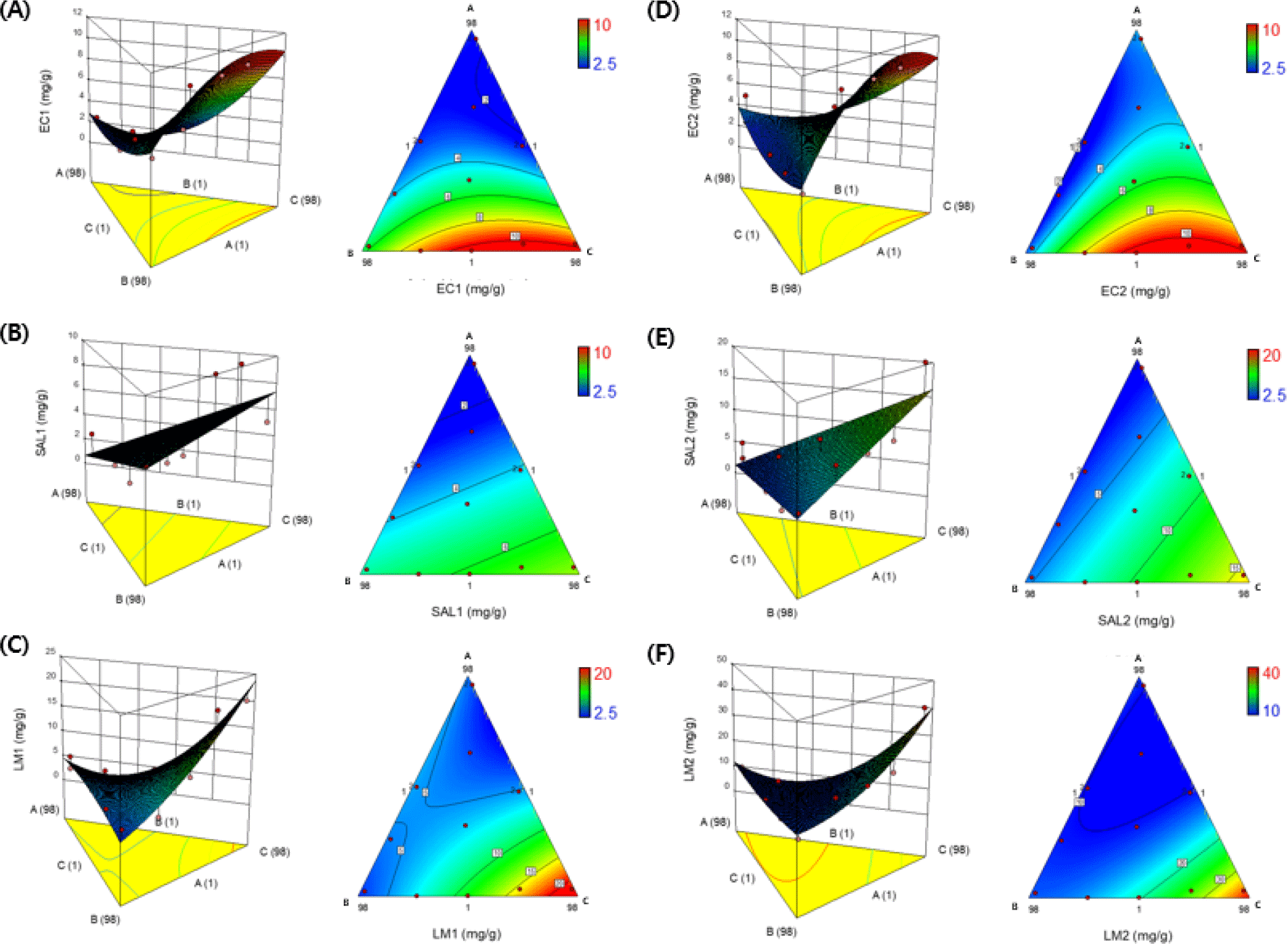
Various compounds can be mixed either by mixing EO and plant extracts and blending several types of plant extracts (Mau et al., 2001; Poimenidou et al., 2016). This approach has received significant attention because of its potential use as a natural additive in various foods. The combination of plant extracts and the main components of EO (-pinene, camphene, myrcene, -terpinene, p-cymene) can improve antibacterial and antifungal activity compared to individual hydrocarbons (Hossain et al., 2016; Nikkhah et al., 2017). Moreover, p-cymene, found in, both, plant extracts and EO, exhibits a high affinity for cell membranes, making it a membrane-exchanging impurity that assists carvacrol in penetrating cells, thereby increasing antibacterial efficacy (Poimenidou et al., 2016). Mixtures of Calendula officinalis extracts demonstrate excellent antibacterial effects against various bacteria owing to the complementary antibacterial activity of each extract fraction when used in combination (Mau et al., 2001). Therefore, blending various plant extracts is a natural and powerful method of enhancing their antibacterial properties when used as food additives.
In this study, a D-optimal mixture design optimization tool was used to predict the optimal mixing ratio of natural extracts to achieve high antibacterial activity. The formulation 1 (F1) mixing ratio was E. cava 58.40%, P. guajava 39.68%, and P. japonica (Makino) Miyabe & Takeda 1.92%. Table 3 shows the antibacterial test results of the composite extracts prepared under the aforementioned conditions for the three pathogens. F1 exhibited antibacterial activity against E. coli at 2.5 mg/mL for both MIC and MBC and against Salmonella spp. at 1.25 and 2.5 mg/mL for MIC and MBC, respectively. In the case of L. monocytogenes, the MIC concentration was 2.5 mg/mL, and the MBC concentration was 5.0 mg/mL, indicating antibacterial activity. Typically, a desirability value of 0.8–1.0 is considered favorable for product quality, and F1 scored 0.97.
Table 4 illustrates antibacterial activity by analyzing growth curves of the pathogens at different concentrations of F1 (0.2%–M0.2, 0.5%–M0.5, and 1.0%–M1.0). This method was used to determine the optimum concentrations for subsequent sausage production. The initial concentrations of the three tested bacteria were 3–4 Log CFU/mL. All concentrations of the mixed extract gradually reduced the growth of all the three types of bacteria over 2 d. In particular, F1 concentrations of 0.5% and 1% completely inhibited E. coli and L. monocytogenes after 2 d. Thus, different concentrations of the natural extract mixture presumably controlled bacterial growth. Additionally, growth of all the pathogens in the grapefruit seed group was inhibited after 1 d. In contrast, the control and SOR-treated groups showed increased bacterial growth after 2 d.
Developing natural extract mixtures using a central mixture design approach is an important technique for creating natural preservatives to replace chemical preservatives in the food industry. This helps minimize damage to the sensory properties of foods while effectively controlling pathogens through the potential synergy between extracts. Combinations of antimicrobial compounds can reduce undesirable organoleptic properties of foods by using lower effective concentrations in foods (Tiwari et al., 2009).
pH and color are important quality-indicating properties of meat products, such as sausages (Lee et al., 2021). The changes in pH and color of sausages in this study are presented in Table 5. The highest pH value at week 0 of treatment was observed for SOR treatment (T1), followed by that of the control (p<0.05). The grape seed and natural extract treatments had the lowest pH values (p<0.05), and significant differences between these treatments were not observed (p>0.05). These findings could be attributed to the presence of phenolic compounds and organic acids in the natural extracts (Lee et al., 2021; Woo et al., 2023). The typical pH values of natural compounds vary the pH of meat products (Choi et al., 2022; Kim et al., 2019; Lee et al., 2021; Woo et al., 2023). Changes in pH during storage are important in the manufacture of meat products. In emulsified sausages, a stable pH during storage is important because oxidation and microbial growth in meat products are affected by pH (Song et al., 2017). Although microbial growth was majorly inhibited by heating (Shin et al., 2017), microbial changes were observed in the control group at weeks 3 and 4 (Table 6). In addition, pH of the sausages declined during storage due to the oxidation of lipids and proteins. Decay metabolites (volatile basic nitrogen) produced by microbes (gram-negative bacteria) during storage also affect the pH of sausages (Hwang et al., 2017); the TBARS value of the control was the highest (p<0.05). Therefore, these results may have affected the pH value of the control, and sausages containing natural extracts may have more stable quality characteristics than those of the control.
The typical color of the added natural extract has a critical impact on the color value of meat products (Kim et al., 2019). The color values of the emulsified sausages are listed in Table 5. Treatments with natural extracts had lower CIE L* and CIE b* values and higher CIE a* values than those of T1 and T2 (p<0.05). After 1 wk, the CIE L* value increased, but decreased after 2 wk. This result may be due to the exudate from the sausages at week 1. The light-scattering effects of moisture on the surface increased the CIE L* value of the sausages. During the storage period, sausages lose excessive moisture, causing them to dry and reduce their CIE L* (Shin et al., 2017; Song et al., 2017). They also tend to show a decrease in CIE L*, CIE a*, and CIE b* as the color pigments are oxidized and denatured over time (Lee et al., 2021; Shin et al., 2017; Woo et al., 2023).
The antioxidant effects of the natural extracts on pork sausage were evaluated by analyzing TBARS during the 4 wk of storage (Fig. 2). On oxidation, lipids form aldehydes as secondary oxidants, one of which, malondialdehyde (MDA) reacts with thiobarbituric acid. Thus, the TBARS value was used as a parameter of lipid oxidation. During the manufacturing and thermal treatment of the sausages, the TBARS values of the control increased owing to thermal stress. Compared with the control, sausages with synthetic or natural preservatives showed significantly lower TBARS values at 0 wk of storage (p<0.05). Natural extracts were more effective in preventing lipid oxidation than those using SOR and GFSs, regardless of the amount of ascorbic acid added. During the storage period, the control, T1, and T2 groups showed an increase in TBARS values until 3 wk, followed by a decrease at 4 wk. Elevated TBARS values due to lipid oxidation are associated with the rancid flavor of meat (Lai et al., 1995). The decrease in TBARS values after 4 wk can be attributed to the degradation of MDA by further oxidation, which generates other alcohols and acid products (Azizkhani and Tooryan, 2015). The addition of natural extracts to pork sausages prevented an increase in TBARS values to less than 0.4 mg/kg during 4 wk. The natural extract mixture composed of E. cava, P. guajava, and P. japonica (Makino) Miyabe & Takeda showed excellent antioxidant activity with high polyphenol and flavonoid content (Camarena-Tello et al., 2018; Kim et al., 2016; Senevirathne et al., 2006). In E. cava, numerous phlorotannins were contained, and especially 6,6′-bieckol, one of the phlorotannins, had prominent antioxidant activity with high yield (Li et al., 2009). In addition, according to a previous research, ethanol extract of P. guajava leaf showed remarkable antioxidant effect equivalent to 4.91 mM/mg of trolox (Tachakittirungrod et al., 2007). Also in another study, ethanol extract of P. japonica showed high polyphenol content (125.1 mg/g) and flavonoid content (136.1 mg/g; Kim et al., 2016). Plant phenolic compounds are known food antioxidants; however, their optimum concentration is important for effective antioxidant activity (Balasundram et al., 2006). Therefore, compared to SOR and grapeseed extracts, the optimized natural extract and its concentration in our study were appropriate for use as an antioxidant for lipids in emulsion-type pork sausages.
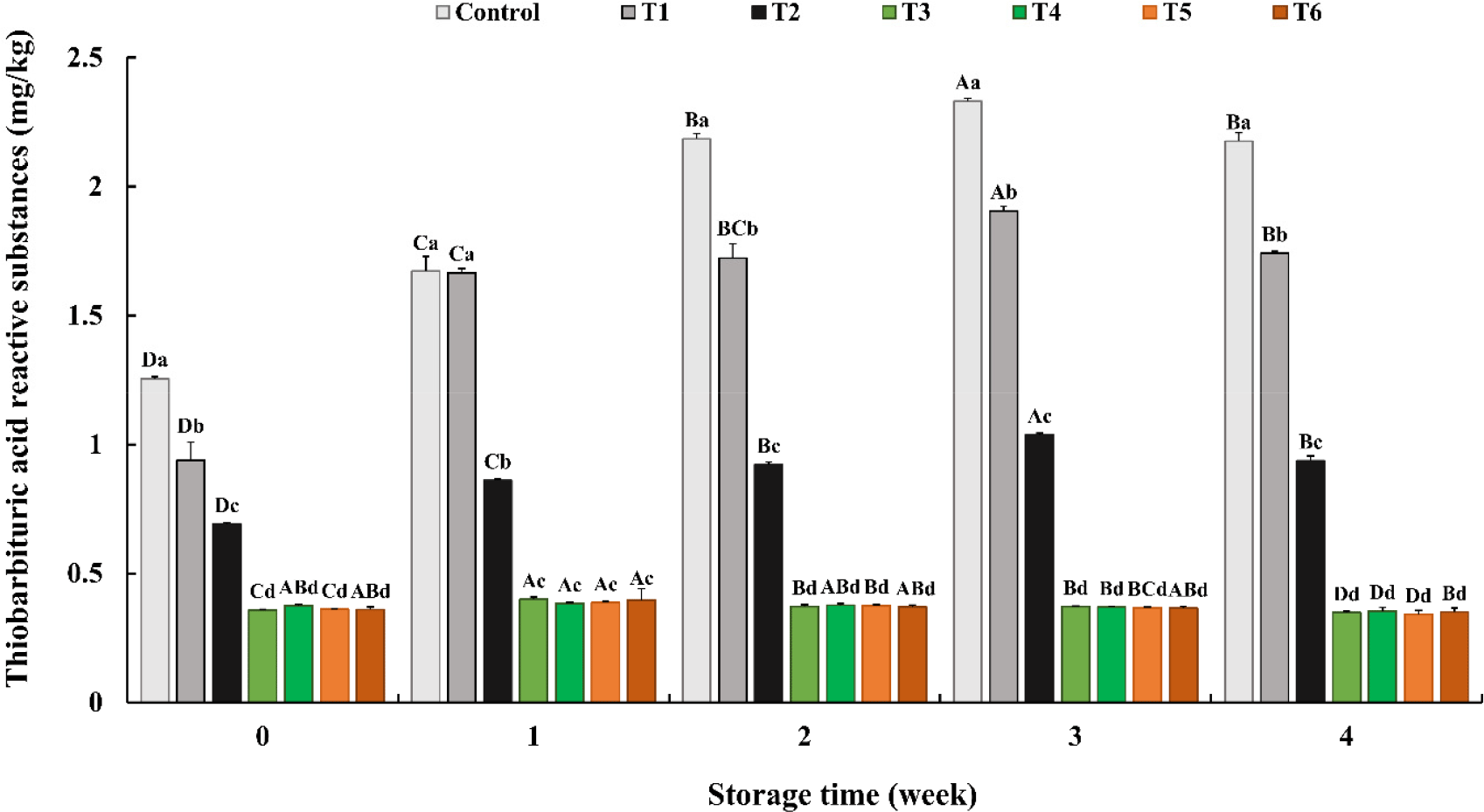
The TPC of sausages containing preservatives during 28 d of storage was shown in Table 6. Total microbes were not detected during the storage period in the treatments with preservatives added, including sausage in natural preservative extract. The control was not detected until the 14 d of storage. It was increased to 2.91 Log CFU/g after 28 d. This result is in line with those reported by Qiu and Chin (2022), who found that the addition of lotus rhizome root powder with antibacterial activity reduces the total bacterial counts in sausages compared with those of the control during storage. Also, Coliform and E. coli, were not detected during the storage period in all treatments. The particular species of bacteria that contaminate the meat will determine the spoilage profile of muscle foods stored under environmental conditions (Tajik et al., 2014). Mesophilic bacteria are the most important spoilage microorganisms that deteriorate meat and meat products during storage (Alirezalu et al., 2019). The natural preservatives used in this study were mixed extracts of E. cava, P. guajava, and P. japonica (Makino) Miyabe & Takeda. It has been reported that guava leaf extract shows high antibacterial activity against gram-positive bacteria and that the flavonoid compounds with antibacterial activity (Arima and Danno, 2002; Jo et al., 2009). E. cava and Paeoniae radix extracts were also reported for antibacterial activity (Ahn, 1998; Chang et al., 1996; Lim et al., 2008; Park and Cho, 2010). Fu et al. (2007) reported a combination of different plant-originated compounds could have additive, synergistic or antagonistic effects depending on the type of microorganism, and the results were in the same vein as our study. Therefore, these results suggest that the natural extracts could have applications as a natural preservative for sausage.
Table 7 shows the average sensory scores and SD of the sausage samples. Fig. 3 summarizes the results of the sensory evaluation. The changes in overall acceptability are shown in Fig. 3A. After a storage of 4 wk, the mean score for overall acceptability significantly decreased (p<0.05) in the control group (no preservatives). Thus, the preservatives in sausages not only controlled the growth of bacteria but also affected flavor. Compared to the other groups, the control group showed higher TBARS values, which represent the degree of lipid oxidation which is highly associated with the quality of sausages. Initially, the overall acceptability value was the highest in T3 and remained the highest among the samples after 4 wk. Natural extracts (T3) imparted an acceptable appearance and flavor to sausages. In contrast, T2 had the lowest overall acceptability value among the samples. This may be because natural preservatives, including natural extracts and grapefruit seeds, affect the flavor of sausages. It was reported that chicken breast with grapefruit extract had a higher score of overall acceptability than the untreated samples (Kang et al., 2017). However, in this study, the addition of GFS to pork sausage had negatively affected overall acceptability. On the other hand, a previous study (Velasco-Arango et al., 2021) showed that a higher mass fraction of guava extract, which is an ingredient of the natural extract in T3, led to a decrease in the acceptance of the sausage. It might be explained that the sensory results may vary depending on the amount of natural extract added and the target to which they are added.
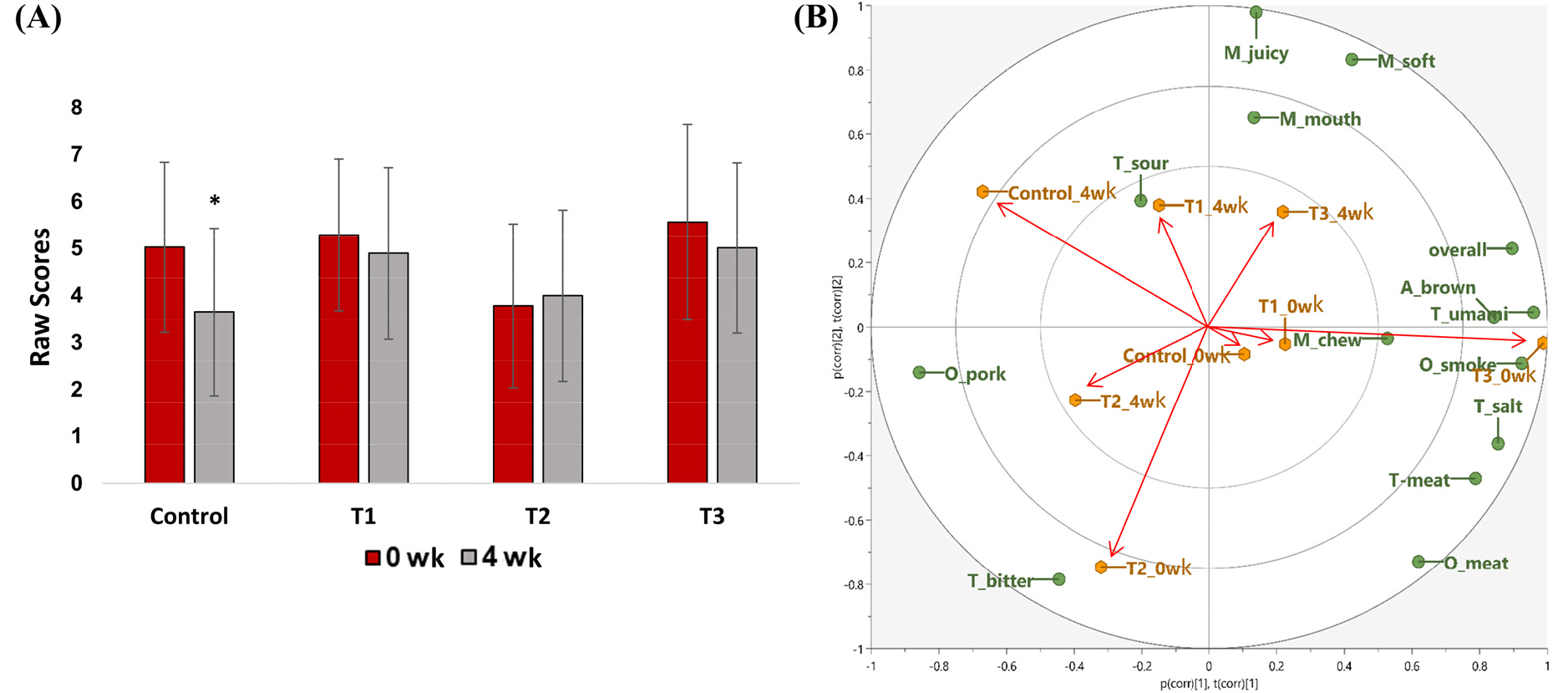
The PCA biplot visualizes the sensory attributes and shows the separate groups of sausages with different storage times and types of preservatives (Fig. 3B). The biplot explains 73% of the total variation, including PC1 (44% of the variance) and PC2 (29%). R2 was used to evaluate the goodness-of-fit of the PCA model. A higher R2 value indicates higher explanatory power of the regression model (Yang et al., 2018). In this study, the PCA model performed well (R2X=0.73) in discriminating between samples. In the PCA score plot, T2 and T3 showed different flavor profiles compared to those of the control and T1. Some sensory attributes, such as brownness, umami, and smoky odor, were strongly associated with T3, whereas T2 was associated with bitter taste. After storage for 4 wk, the sensory characteristics of the samples weakened, and other sensory features were up- or downregulated. For example, some sensory properties, such as meaty odor, saltiness, and meaty taste, decreased in all samples after 4 wk. Sourness affected the flavor of T1 after 4 wk of storage. The sourness score decreased with storage in T1 but increased in the other samples. Pork odor and bitterness, which are located in the dimension opposite to overall acceptability, could negatively affect overall acceptability. In this study, the sensory properties of T2, which were located on the opposite dimension of overall acceptability, may have a negative effect on the quality of pork sausage, while those of T3 were related to a positive effect on the quality of pork sausage. Additionally, studies have shown that adding ascorbic acid to natural extracts increases the antibacterial effect (Gedikoğlu et al., 2022). However, no significant effect of ascorbic acid was observed in our study. These results suggest that the natural extract used in this study can served as a promising alternative preservative for sausages.
Conclusion
In this study, the optimal mixing ratio of three carefully selected natural extracts (E. cava, P. guajava, P. japonica (Makino) Miyabe & Takeda) was established to obtain an extraction mixture with antibacterial and antifungal activity against various microorganisms. Storage tests were conducted by applying mixed extracts of various concentrations to sausages, and ascorbic acid was added to confirm the synergistic effect on the storage stability of sausages. Naturally derived E. cava, P. guajava, and P. japonica (Makino) Miyabe & Takeda mixtures showed preservative effects similar to those using GFS. For the same amount of preservative, the natural extract group had a higher overall acceptability than that of the GFS treatment group in the sensory evaluation. Therefore, the mixture of E. cava, P. guajava, and P. japonica (Makino) Miyabe & Takeda prepared in this study can be used as a potential natural preservative in meat products.