Introduction
Currently, Korea is facing rapid changes in population structure and lifestyle, and the food industry is greatly affected by such changes in social structure (Cha and Rha, 2021). With the increase in per capita income, consumers are investing in more delicious and immune-boosting meals. In particular, the demand for meat, including beef, which is considered an immune-boosting food rich in protein, zinc, iron, and vitamin D, is steadily increasing (Kim and Na, 2001; Whitnall and Pitts, 2019). Many studies on livestock have already been conducted in advanced countries to scientifically investigate the functionality of meats such as beef, chicken, and pork in response to consumers’ demands; however, research on the same remains insufficient in Korea. In the future, various breeds and imported beef are expected to flow into Korea through various channels such as free trade agreements. Therefore, preparing the necessary domestic countermeasures accordingly is essential.
Hanwoo (Bos taurus coreanae) is the representative Korean native cattle. In general, Hanwoo is divided into five types: brown (Hanwoo, major), tiger color (Chickso, Bos namadicus), black (Heukwoo, Bos namadicus Falconer), Jeju black (Jeju Heukwoo, Bos primigenius), and white (Baekwoo; Jo et al., 2012; Kim et al., 2020; Utama et al., 2018).
Hanwoo gave many advantages to farming and transportation with the following strengths: not prone to disease, docile temperament, strong legs and hooves, withstanding rough breeding management, and good fertility (Lee et al., 2014). However, Hanwoo is currently being bred for meat and is raised in intensive farming to obtain beef. In addition, Korean meat quality grading system mainly evaluates marbling, and it can be said that Korean consumers’ tendency to value the amount and taste of fat rather than lean meat has created today’s Korean beef (Alam et al., 2013; Joo et al., 2017). Korean studies on the quality of Korean beef have revealed that Korean beef has superior muscle fat accumulation, unsaturated fatty acid composition, and sensorial properties compared to imported beef (Hwang, 2004; Park and Yoo, 1994). In addition, many studies have reported the excellent health-improving properties (antioxidant ability, anti-hypertension, anti-inflammatory, and immune-boosting effects) of Korean beef (Seol et al., 2018).
Chickso is one of the native breeds of Korean native cattle that has been bred for a long time besides with Hanwoo. It has dark brown and black vertical stripes on a yellowish-brown background, resembling a tiger pattern. Chickso has lower marbling than Hanwoo, but it has a higher oleic acid content than regular Hanwoo, which gives the meat a soft and unique taste (Lee and Joo, 2022; Utama et al., 2018). In particular, Chickso has a reddish color and strong meat flavor compared to Hanwoo, thus research and commercialization of aging, processing, cutting, and cooking methods are necessary. Furthermore, although a few comparative studies on the quality of foreign and native breeds have been conducted, no study has reported the functional properties of livestock products derived from traditional Korean cattle except for Hanwoo. Therefore, it is considered necessary to study the meat quality and functional characteristics of Chickso to establish it as a new breed different from Hanwoo and to lay the foundation for industrialization. Therefore, this study aimed to compare the characteristics of peptides derived from Chickso and Hanwoo, which are traditional domestic breeds, and Wagyu, which is a representative overseas breed.
Materials and Methods
α-Amylase from hog pancreas (CAS No. 9000-90-2), porcine bile extract (CAS No. 8008-63-7), lipase from porcine pancreas (CAS No. 9001-62-1), mucin from porcine stomach Type II (CAS No. 84082-64-4), pepsin from porcine gastric mucosa (CAS No. 9001-75-6), crystalline uric acid (≥99%; CAS No. 69-93-2), 2,2’-azino-bis(3-ethylbenzothiazoline-6-sulfonic acid) (ABTS) diammonium salt (CAS No. 30931-67-0), 2,2-diphenyl-1-picrylhudrazyl (DPPH; CAS No. 1898-66-4), 3-(2-pyridyl)-5,6-diphenyl-1,2,4-triazine-p,p′-disulfonic acid monosodium salt hydrate (ferrozine), iron (II) chloride (CAS No. 7758-94-3), and iron (III) chloride (CAS No. 7705-08-0) were purchased from Sigma-Aldrich (St. Louis, MA, USA). EDTA-2Na dihydrate (CAS No. 6381-92-6), trichloroacetic acid (TCA; CAS No. 76-03-9), sodium phosphate dibasic anhydrous (CAS No. 7558-79-4), and sodium phosphate monobasic anhydrous (CAS No. 7558-80-7) were purchased from Daejung Chemicals (Siheung, Korea). Potassium hexacyanoferrate (III) (CAS No. 13746-66-2) was purchased from Kanto Chemical (Tokyo, Japan) and 2,6-di-tert-butyl-4-methylphenol (99.0%) was purchased from Samchun Pure Chemical (Seoul, Korea).
All animal experiments were approved by the Experimental Animal Ethics Committee of Chung-Ang University (Approval number: 2021-00038) and conducted in accordance with the rules of animal ethics. The institute of cancer research (ICR) mouse used in the experiment were eight-week-old females weighing 25–30 g purchased from Orient Bio. During the adaptation period, the mice were fed with water and pellet ad libitum. During the experiment, the mice were fed with defined amounts of mixed feed crumble and samples. DY-B-0061 bedding (shaving type) was purchased from Duyeol Biotech. The breeding environment was maintained at 22±2°C temperature, 60±5% relative humidity, and 12-hour light:dark cycle (07:00–19:00).
Fig. 1 shows the process followed for preparing peptide extracts from the loin and shank of Chickso (Bos namadicus Falconer), Hanwoo (Bos taurus coreanae), and Wagyu (Bos taurus) cattle. The Chickso loin and shank cuts used in the experiment were purchased from Woosung Ranch (1328, Chuseong-ro, Damyang-eup, Damyang-gun, Jeollanam-do) and Goseong Chickso (312-23, Sinan-ri, Ganseong-eup, Goseong-gun, Gangwon-do) in Korea. The Hanwoo loin and shank cuts were purchased from Famous Livestock (688-7, Nae-ri, Daedeok-myeon, Anseong-si, Gyeonggi-do) and Manse Livestock in Korea (15, Deokbongseowon-ro, Gongdo-eup, Anseong-si, Gyeonggi-do) in Korea. The Wagyu loin and shank cuts were purchased from Meatbox Global (22, Teheran-ro 34-gil, Gangnam-gu, Seoul) in Korea. Each sample (500 g) was completely ground using a stand mixer (SHMF-H3500TG, Hanil Electric, Bucheon, Korea). Subsequently, 10 L of distilled water (DW) was added and the samples were washed 20 times for about 30 min each time to remove as much blood and fat as much as possible. Next, the samples were homogenized using a total of 4 L of 0.04 M phosphate buffer (PBS; pH 7.4) and then centrifuged at 1,977×g for 15 min at 4°C to obtain myofibrillar proteins. Thereafter, they were hydrolyzed with alkaline-AK enzyme (protein hydrolysate obtained by fermenting soybean meal with Bacillus methylotrophicus) at pH 11.0 for 2 h at 60°C. The protein hydrolysates were heated at 80°C for 15 min to deactivate the enzyme action and dried in a 55°C dry oven for 24 h. The dried protein hydrolysates were diluted in DW at a concentration of 25 mg/mL. Subsequently, peptides were fractionated to a size of 10 kDa or less through ultrafiltration using an Amicon filter tube (Amicon Ultra, Darmstadt, Germany) at 2,306×g for 15 min and then freeze-dried at –70°C.
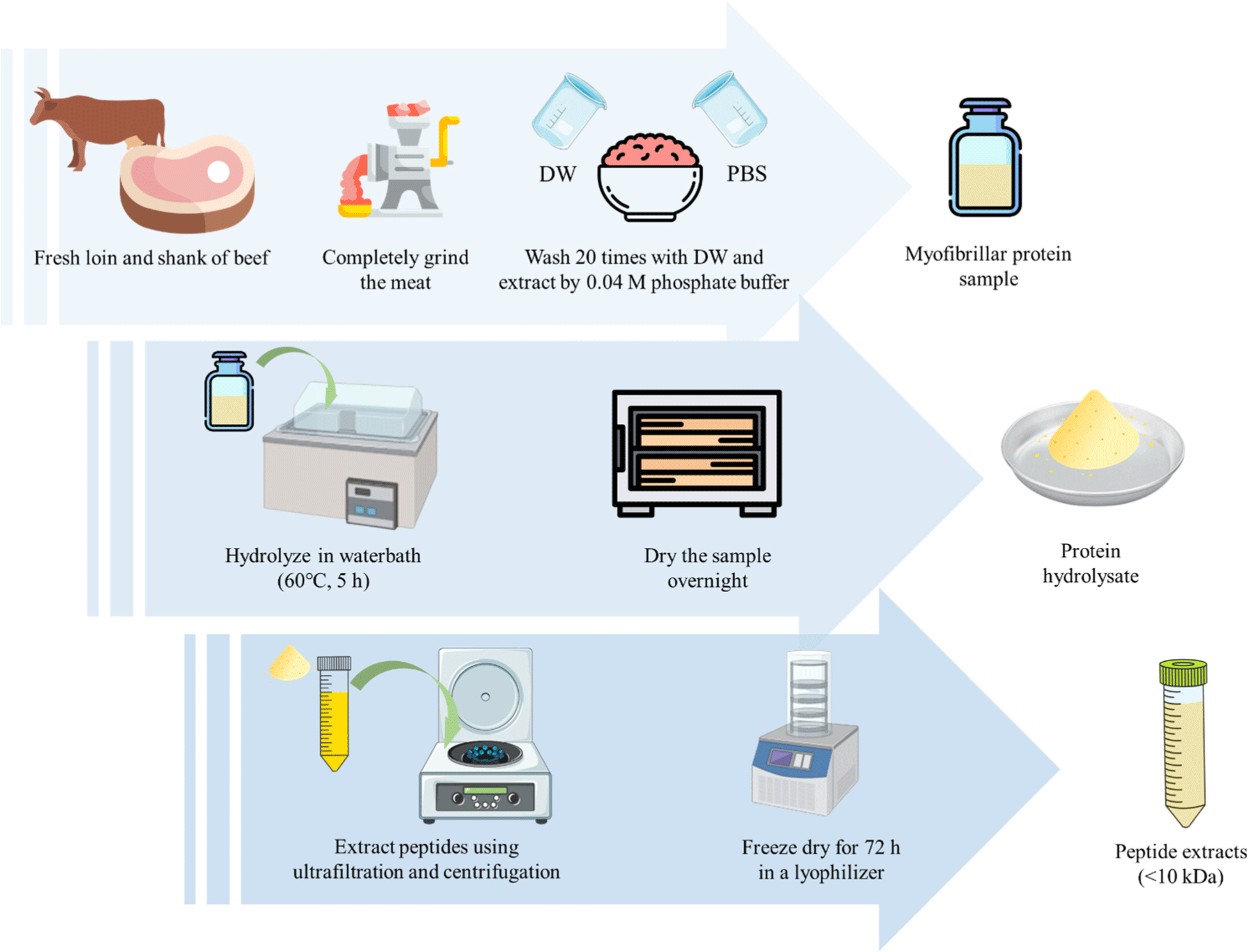
The preparation conditions of synthetic digestive juices used in the human in vitro digestion model are described in Table 1 (Hur et al., 2011). Samples (120 mg) of loin and shank peptide extracts from Chickso, Hanwoo, and Wagyu were transferred into 50 mL tubes. Saliva (5 mL) was added and the mixture was shaken at 150 rpm for 5 min at 37°C in a shaking water bath to carry out the oral digestion step. Subsequently, 10 mL of gastric juice was added to the tube and reacted in the shaking water bath at 150 rpm for 2 h at 37°C to conduct the gastrointestinal digestion step. After the reaction, 10 mL of small intestinal juice and 5 mL of bile were added into the tube and reacted at 150 rpm for 2 h at 37°C to perform the small intestinal digestion step. After all the digestive steps were completed, 25 mL and 15 mL of DW were added to the tubes of the oral and gastrointestinal digestive steps, respectively. The final volume of the samples was 35 mL. In all in vitro digestion experiments, DW was added instead of digestive enzymes to obtain pre-digestion control samples under the same conditions. After the digestion experiment, all samples were centrifuged at 13,000×g for 20 min at 4°C and then the supernatants were extracted and used as samples.
A gel permeation chromatography system was used to analyze the molecular weight distribution before and after in vitro digestion of Chickso, Hanwoo, and Wagyu loin and shank peptide extracts. Specifically, an ACQUITY APC System (Waters, Milford, MA, USA) with an Xbridge Protein BEH SEC column (Xbridge Protein, San Jose, CA, USA) was used for the analysis. All samples were filtered with a 0.2 μm syringe filter before use. Deionized water (A) and MeOH (B) were used as mobile phase solvents; the composition was 90% A and 10% B and the flow rate was 0.7 mL/min.
A Hitachi L-8900 amino acid analyzer (Hitachi High-Technologies, Tokyo, Japan) was used to analyze changes in the composition of the free amino acids before and after in vitro digestion of Chickso, Hanwoo, and Wagyu loin and shank peptide extracts. After diluting each sample to an appropriate concentration, 1 mL of 5% TCA solution was added to 1 mL of the sample, vortexed, and then centrifuged at 13,572×g for 20 min at 20°C. Thereafter, 4 mL of n-hexane was added to 2 mL of the supernatant, agitated for 10 min, and then centrifuged at 1,977×g for 20 min at 20°C. Subsequently, the lower layer solution was obtained and the centrifugation step was repeated twice to completely remove the n-hexane. Then, the samples were filtered using a 0.2 μm syringe filter and used for analysis.
ABTS, DPPH, iron-chelating, and reducing power assays were used to analyze the antioxidant activity. ABTS radical scavenging activity was analyzed using Solution 1, prepared by mixing 0.038 mg of ABTS with 10 mL of DW according to the method described by Arts et al. (2004), and Solution 2, prepared by mixing 33 mg of potassium persulfate with 50 mL of DW. The ABTS solution was prepared by mixing 10 mL each of Solutions 1 and 2, covered with aluminum foil, and overnight incubated at room temperature to continue the activation of the solution. Twenty-microliter of samples were divided into a 96-well plate, followed by the addition of 180 μL working solution of ABTS reagent in MeOH. The well plate was wrapped in foil and the samples were allowed to react for 10 min. Then, the absorbance of the samples was measured at a wavelength of 734 nm using a microplate reader (Spectramax 190, Molecular Device, San Jose, CA, USA).
The DPPH radical scavenging activity was measured using the method described by Brand-Williams et al. (1995). DPPH (2 mg) and MeOH (25 mL) were mixed to prepare a DPPH solution, covered with aluminum foil, and refrigerated. The samples (0.1 mL) were added to a well plate and 0.1 mL of the DPPH solution was mixed with the samples. Subsequently, the well plate was wrapped in foil and reacted at room temperature for 30 min. Then, the absorbance of the samples was measured at a wavelength of 517 nm using a microplate reader (Spectramax 190, Molecular Device).
The iron-chelating assay was performed according to the method described by Decker and Welch (1990). EDTA-2Na dihydrate was used as the standard solution, and DW the solvent in which the sample was dissolved was set as the control. After adding 200 μL of each prepared sample into individual 1.5 mL tubes, 200 μL of 2 mM iron (II) chloride and 40 μL of 5 mM ferrozine were added. After vortexing and reacting at room temperature for 10 min, the absorbance of the samples was measured at a wavelength of 562 nm using a microplate reader (Spectramax 190, Molecular Device).
The reducing power assay was conducted using the method described by Oyaizu (1986), with slight modifications. Briefly, 2.5 mL of 10% potassium ferricyanide and 2.5 mL of 0.2 M sodium PBS (pH 6.6) were added to 2.5 mL of the samples dissolved in ethanol and then reacted in an incubator at 50°C for 20 min. Subsequently, 2.5 mL of 10% TCA was added to terminate the reaction and the mixture was centrifuged at 2,841×g for 10 min. The supernatant was collected and mixed with a 0.1% ferric chloride solution in a ratio of 5:1 (v/v). Subsequently, the absorbance of the samples was measured at a wavelength of 700 nm to evaluate the reducing power.
To measure the antihypertensive activity using the angiotensin-converting enzyme (ACE) inhibitory assay, 50 mg/mL (w/v) of rabbit lung acetone powder (L0756, Sigma-Aldrich) was added to a 0.1 M sodium borate buffer (pH 8.3) containing 0.5 M sodium chloride. The mixture was stirred at 4°C for approximately 18–24 h for extraction. Subsequently, the supernatant was collected through centrifugation at 9,425×g for 30 min at 4°C to prepare the ACE solution. Next, a 0.1 M sodium borate buffer (pH 8.3) was prepared by appropriately mixing sodium tetraborate and boric acid to achieve a pH of 8.3. Hippuryl-His-Leu was added to the buffer to obtain a final concentration of 8.3 mM and the resulting solution was used as the ACE substrate.
Each sample (50 μL) was placed in a 2 mL tube, and a sample with DW only was used as the control. ACE substrate (50 μL) was added to each of the prepared samples and reacted for 10 min at 37°C. Thereafter, 50 μL of the ACE solution (25 mM/mL) was added and the mixture was incubated at 37°C for 30 min. Subsequently, 250 μL of 1 M HCl was added to a sample to stop the reaction and then 500 μL of ethyl acetate was added and the mixture was vortexed for 1 min. After centrifugation at 1,977×g for 10 min, 200 μL of the supernatant was taken and dried at 60°C for 30 min. Then, 1 mL of DW was added to it. The antihypertensive activity of the prepared sample was evaluated by measuring its absorbance at a wavelength of 228 nm using a UV/Vis Spectrophotometer (Agilent Technologies, Santa Clara, CA, USA).
A total of 21 eight-week-old female ICR mice were randomly distributed into the control and treatment cages. The experiment was conducted for 21 d after a two-week adaptation period. The daily feed intake of general ICR mice was limited to 5 g, and the peptide extract was diluted in 100 μL DW to obtain a concentration of 800 mg/kg and then administered orally once a day using an oral injection needle.
After the peptide extract feeding experiment was completed, all experimental animals were sacrificed using CO2 gas, and their heart blood was collected. Blood collected for antioxidant enzyme activity analysis was centrifuged at 2,000×g for 10 min at 4°C using a plasma separation gel tube (Gsmeditech, Wonju, Korea). The plasma obtained was used as a sample for the antioxidant activity analyses, and the serum obtained by centrifugation for 15 min using a serum separation gel tube (Gsmeditech) was used for ACE activity analysis.
Catalase activity of the samples were determined using an OxiTec Catalase Assay Kit (Biomax, Guri, Korea). Antioxidant activity was measured through three experiments using mice plasma samples. The prepared samples (25 μL) were added to a 96-well plate containing 25 μL of a 40 μM H2O2 solution. The resulting samples were reacted under dark conditions for 30 min at room temperature. After the reaction was completed, 50 μL of Oxi-Probe/HRP working solution was added to the wells and reacted at 20°C–25°C for 30 min. Subsequently, the absorbance of the samples was measured at 570 nm. The amount of enzyme that will decompose 1 μM of H2O2 per min at pH 7.0 at 25°C is equated to 1 unit (U) of catalase activity.
The peroxidase (POD) activity was determined by analyzing the content of H2O2 or peroxide present in the samples using an Oxi-Probe/HRP working solution and an OxiTec Hydrogen Peroxide/Peroxidase Assay kit (Biomax). The prepared samples (50 μL) were added to a 96-well plate with 50 μL of Oxi-Probe/H2O2 working solution and reacted under dark conditions for 30 min at 20°C–25°C. Then, the absorbance of the samples was measured at 560 nm using a microplate reader.
The superoxide dismutase (SOD) activity of the samples was determined by forming water-soluble tetrazolium salt (WST)-formazan complex through a redox reaction of xanthone oxidase and WST using a OxiTec SOD assay kit (Biomax). The prepared samples (20 μL) were added to a 96-well plate, and 200 μL of WST working solution was added to each well. Then, 20 μL of enzyme working solution was added to the well and mixed carefully. Thereafter, the plate was reacted at 37°C for 30 min, and the absorbance of the samples was measured at 450 nm using a microplate reader.
ACE activity was measured using an ACE activity assay kit (Elabscience, Houston, TX, USA). The absorbances of the sample and the blank were measured at 340 nm using a UV/Vis Spectrophotometer (Agilent Technologies). The ACE activity (U/L) was calculated using the following formula:
where, ε is the extinction coefficient (0.8 L/mmol/cm) of a wavelength of 340 nm at an optical distance of 1 cm, d is the optical distance of a quartz cuvette (0.5 cm), DF is the dilution factor, and the reaction time is 5 min.
For the analysis of the experimental results, a statistical analysis was conducted after obtaining the mean and SD using SPSS Statics 26 (IBM, Armonk, NY, USA). The Student’s t-test was used for analyzing the difference between two variables and the one-way analysis of variance method was used for analyzing the differences among three or more variables. The Student–Newman–Keuls post-test was conducted at a significance level of p<0.05 for each average difference.
Results and Discussion
Tables 2 and 3 present the molecular weight distributions of the peptide extracts derived from Chickso, Hanwoo, and Wagyu beef before and after in vitro digestion. The degree of decomposition of the peptide due to digestion could be predicted based on the change in molecular weight of the peptide extract. Irrespective of the breeds and cuts, there was an increase in the number of low-molecular-weight peptides after digestion. In the pre-digestion samples, peptides with a molecular weight of 3,000–10,000 Da were present. However, most of them were digested into low-molecular-weight peptides of 1,000 Da or less after digestion. The proportion of 200–1,000 Da peptides among the loin and shank peptide extracts of Chickso before in vitro digestion was approximately 58% and 39%, respectively, but after in vitro digestion, it was approximately 83% and 80%, respectively, indicating a significant increase in the proportion of low-molecular-weight peptides after digestion. These results indicate that the peptide bonds were cleaved by hydrolysis during in vitro digestion.
According to previous studies, the biological activity of peptides depends on the molecular structure and physicochemical properties of peptides, and small peptides have higher bioavailability and bioactivity (Udenigwe and Aluko, 2012). Therefore, the peptide extracts derived from the loin and shank, which are high in low-molecular-weight peptides of less than 3,000 Da, are expected to have efficient antioxidant and antihypertensive activities.
Tables 4 and 5 present the free amino acid compositions of the peptide extracts before and after in vitro digestion. The total free amino acid contents detected after the in vitro digestion of Chickso, Hanwoo, and Wagyu shank were 9,315.42 mg/100 g, 9,153.71 mg/100 g, and 6,864.45 mg/100 g, respectively, which were higher than those detected for the loin peptide extracts of each breed. Regardless of the cuts, the main free amino acids of the peptide extracts before in vitro digestion were glutamic acid, leucine, lysine, isoleucine, and arginine, and the main free amino acids detected while leucine, tyrosine, lysine, arginine, and phenylalanine were dominant after in vitro digestion.
Considering that the content of cysteine and methionine sulfur-containing amino acids known to have antioxidant activity (Atmaca, 2004) increased significantly compared to that before digestion, the peptide extracts can be expected to have higher antioxidant activities after the digestion process. According to Hougland et al. (2013), histidine, tryptophan, and tyrosine are also representative reactive amino acids that can exhibit effective antioxidant activity by reacting with active oxygen species. In addition, peptides with hydrophobic amino acids, such as valine and isoleucine, and aromatic amino acids, such as phenylalanine and tryptophan, contribute to inhibiting ACE activity (Aluko, 2015). Thus, the peptide extracts can also be expected to have higher antihypertensive activities after the digestion process.
The results of the antioxidant analysis based on the in vitro digestion of the loin and shank-derived peptide extracts of Chickso, Hanwoo, and Wagyu beef are shown in Figs. 2, 3, 4, and 5. Approximately 19% of ABTS radical scavenging ability (Fig. 2), 76% of DPPH radical scavenging ability (Fig. 3), 13% of iron chelating ability (Fig. 4), and 6% or more of reducing power (Fig. 5) increased (detail data are not shown). Comparison of the ABTS radical elimination ability of the peptide extracts before digestion showed that the loin had significantly higher antioxidant activity than the shank, and Chickso (75.01%) and Hanwoo (76.06%) loins had higher antioxidant activity than Wagyu loin (71.13%). Furthermore, the DPPH radical scavenging ability of Chickso loin (36.79%) before digestion was approximately 2.9 times higher than that of other breeds, and Chickso loin (46.34%) and shank (43.59%) had the highest DPPH radical scavenging abilities among the digested samples. There was no significant difference in the iron chelating ability between breeds and cuts, and Wagyu samples had the highest iron chelating ability among all pre- and post-digestion samples. Furthermore, Chickso shank (1.04) had the highest reducing power among all pre-digestion samples, and Hanwoo loin (1.07) had the highest reducing power among all post-digestion samples. Among the post-digestion samples, the reducing power of the Hanwoo samples was significantly higher than that of the Chickso and Wagyu samples. A comparison of the molecular weight distribution analysis presented in Tables 2 and 3 revealed that proteins have already been digested enough during peptide extraction to fully express their physiological activity, making it difficult to increase the antioxidant effect through additional decomposition during the digestion process. Chen et al. (1998) stated that low-molecular-weight peptides of 1 kDa or less have antioxidant activity. Similarly, Olagunju et al. (2018) reported that peptides with a molecular weight of 1–3 kDa have a higher antioxidant activity than peptides with other molecular weights. Lee and Hur (2017) also revealed that protein hydrolysates derived from beef source fiber proteins with a molecular weight of 3 kDa or less exhibit strong free radical scavenging ability. The differences in antioxidant activity between breeds and cuts may have been affected by the degree of hydrolysis according to the characteristics of the proteases, the size of peptide molecules, hydrophobicity, amino acid composition, and structural properties of the peptides produced (Pihlanto, 2006).
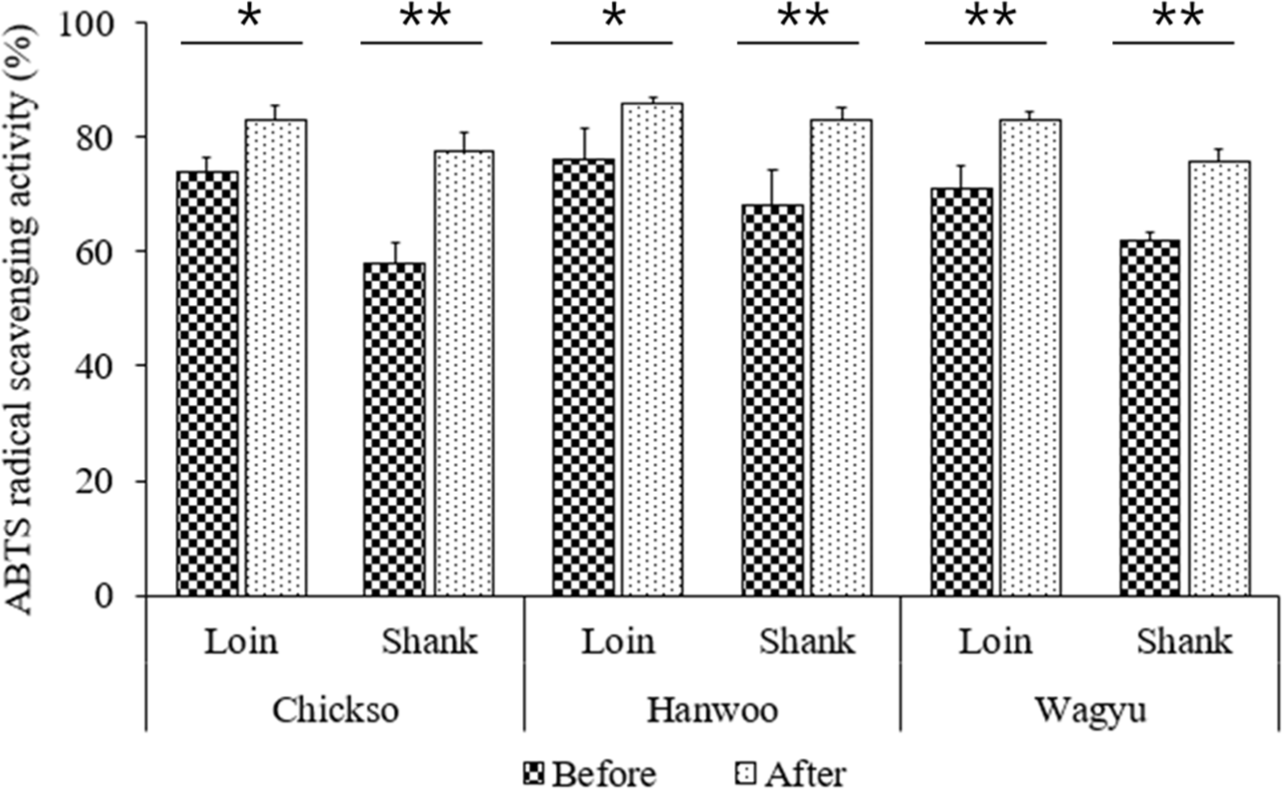
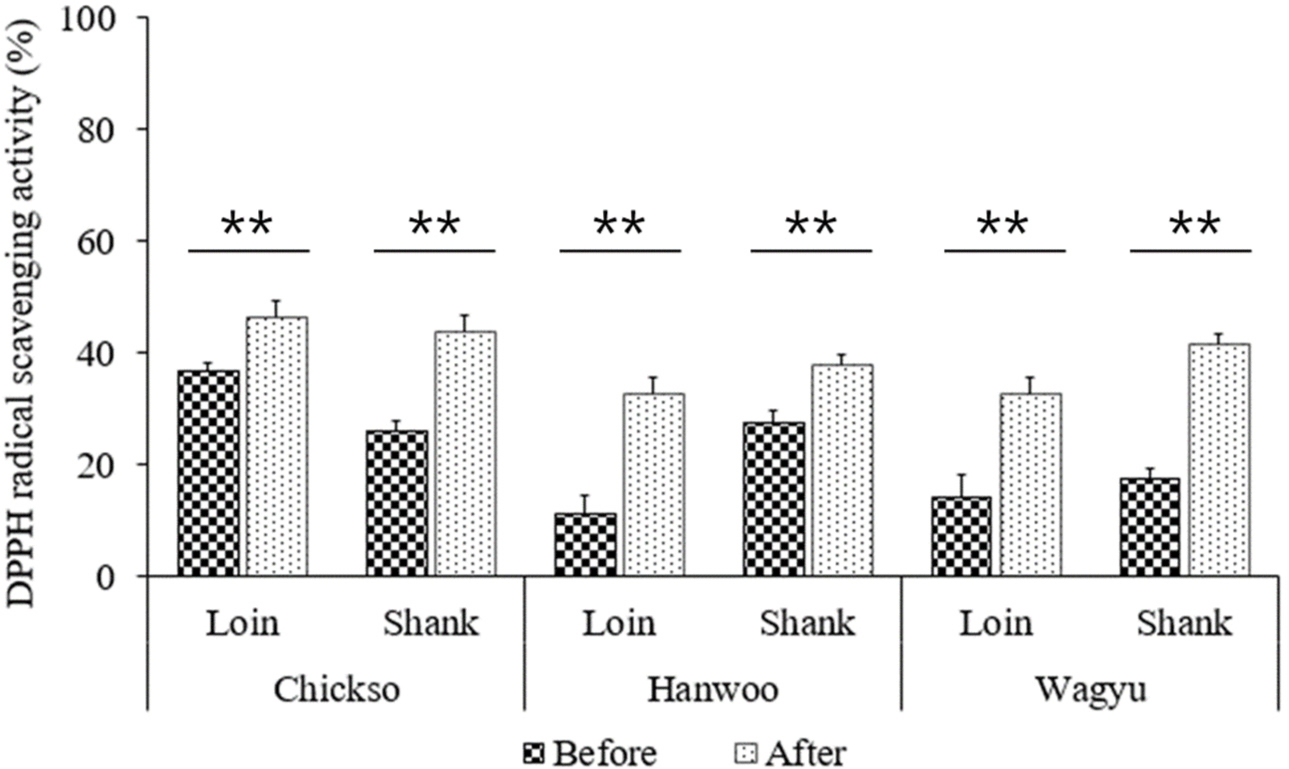
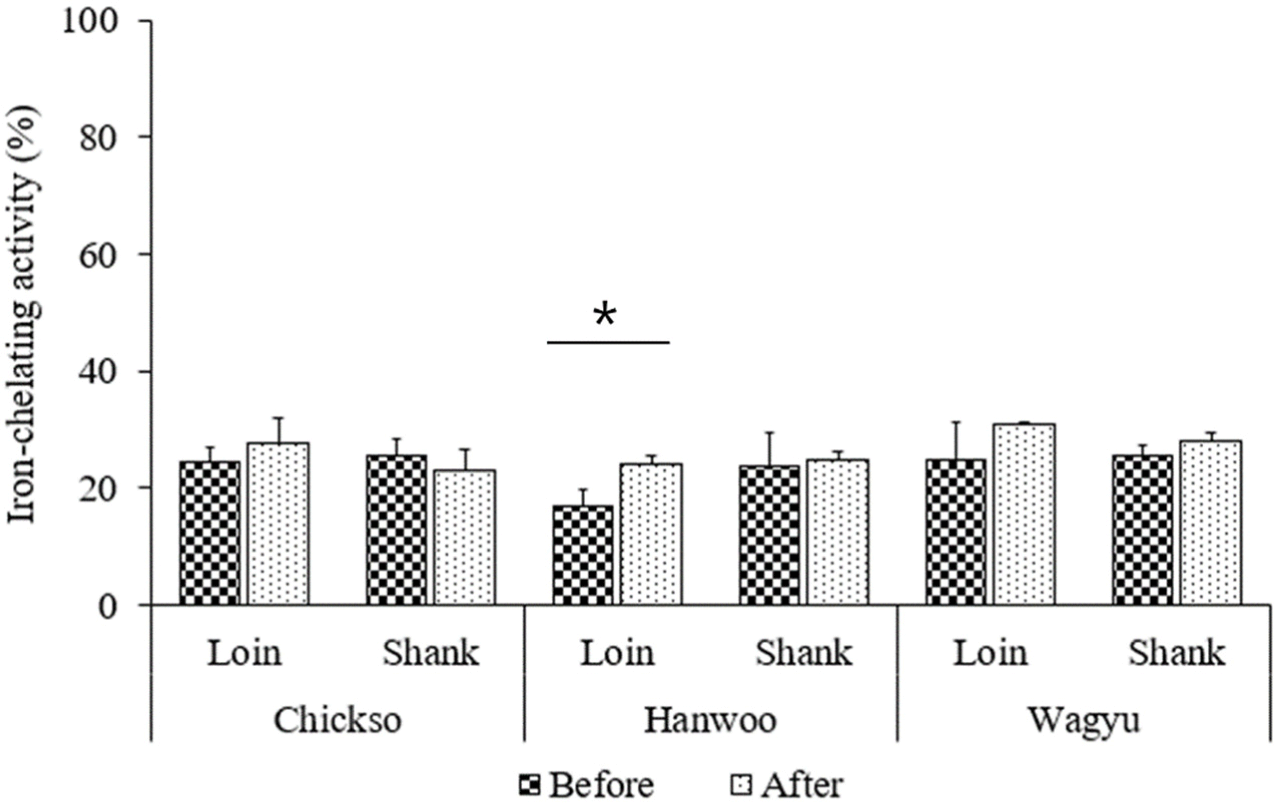
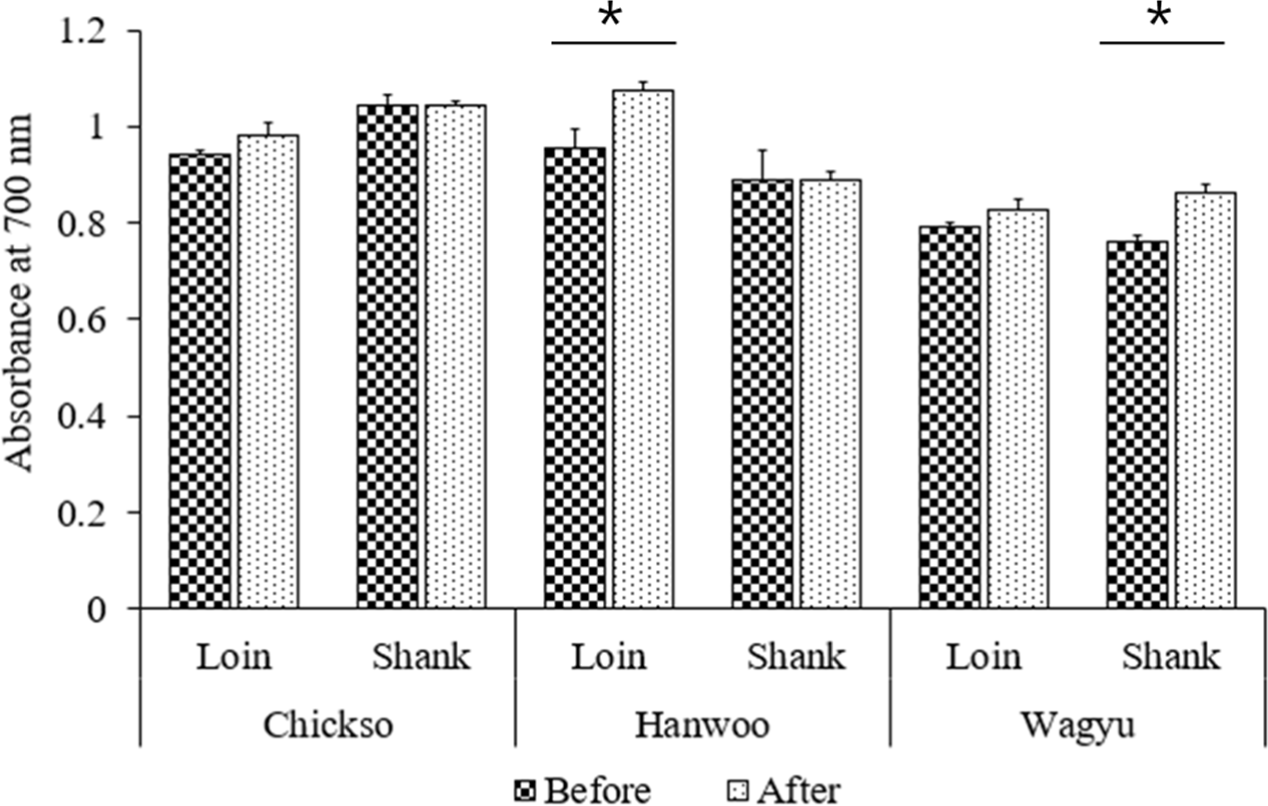
The current study revealed that the antioxidant activity increased slightly when the peptide extracts were hydrolyzed using the in vitro digestion model, and overall, the antioxidant activity of the Chickso and Hanwoo samples was higher than that of the Wagyu samples. These results are likely due to the high content of low-molecular-weight peptides such as glutathione (a crystalline peptide composed of glutamic acid, cysteine, and glycine), which are known to have excellent antioxidant effects (Pompella et al., 2003).
Fig. 6 shows the antihypertensive activities of Hanwoo, Chickso, and Wagyu peptide extracts based on the ACE inhibitor assay. All pre-digestion samples showed a high ACE inhibitory ability of more than 70%, and the peptide extracts of Chickso loin and shank had the highest antihypertensive activities. However, after the digestion process, the ACE inhibition ability in all samples decreased sharply to less than 30%. This was probably because of the enzymatic decomposition of peptides with antihypertensive activity or the loss of activity due to the pH and temperature conditions during the in vitro digestion process. The results of this experiment were similar to those of a previous study by Jang and Lee (2005), who reported that excessive hydrolysis lowers the antihypertensive activity of peptides. Because all samples showed high activity before digestion, it was decided that additional experiments using animal models were needed to determine whether the antihypertensive activity was retained during in vivo digestion.
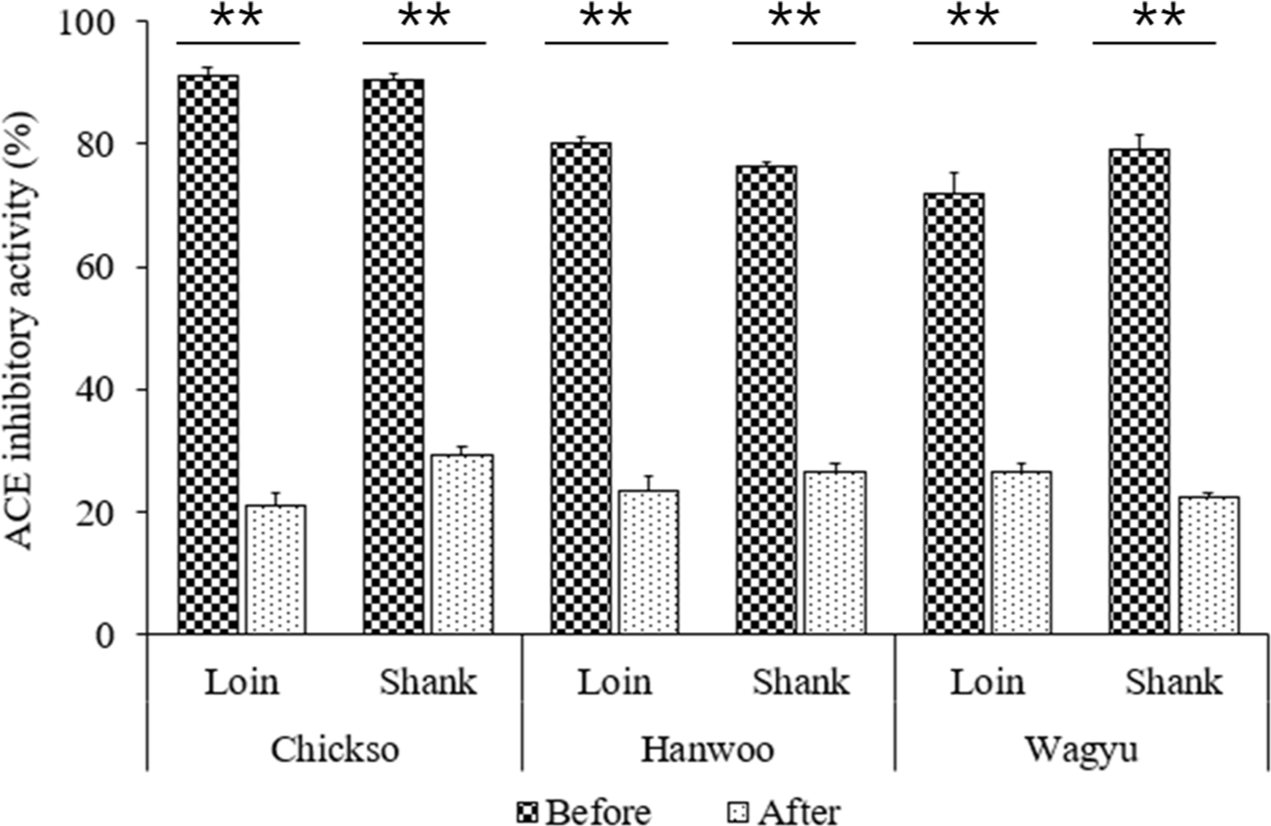
Fig. 7 shows the antioxidant activities observed in mice fed with the peptide extracts derived from the loin and shank of Chickso, Hanwoo, and Wagyu beef. All treatment groups showed catalase activity of more than 340 mU/mL, which was higher than that of the control, and the highest activity was observed in the treatment groups fed with peptide extracts derived from Chickso loin. The POD activity of all treatment groups, especially the groups fed with Chickso and Wagyu loin peptide extracts, was higher than that of the control by 0.38 mU/mL or more. The SOD activity of all treatment groups was similar to or higher than that of the control. In addition, the production rate of methionine, a sulfur-containing amino acid known to have antioxidant activity in proteins, and histidine and tyrosine, reactive amino acids, in Chickso and Wagyu loin/shank peptides were higher than that in the Hanwoo loin/shank peptide samples after digestion (Tables 4 and 5). In general, histidine-containing peptides exhibit antioxidant activity through a single electron transfer mechanism and histidine-containing peptides through a hydrogen atom transfer mechanism (Esfandi et al., 2019).
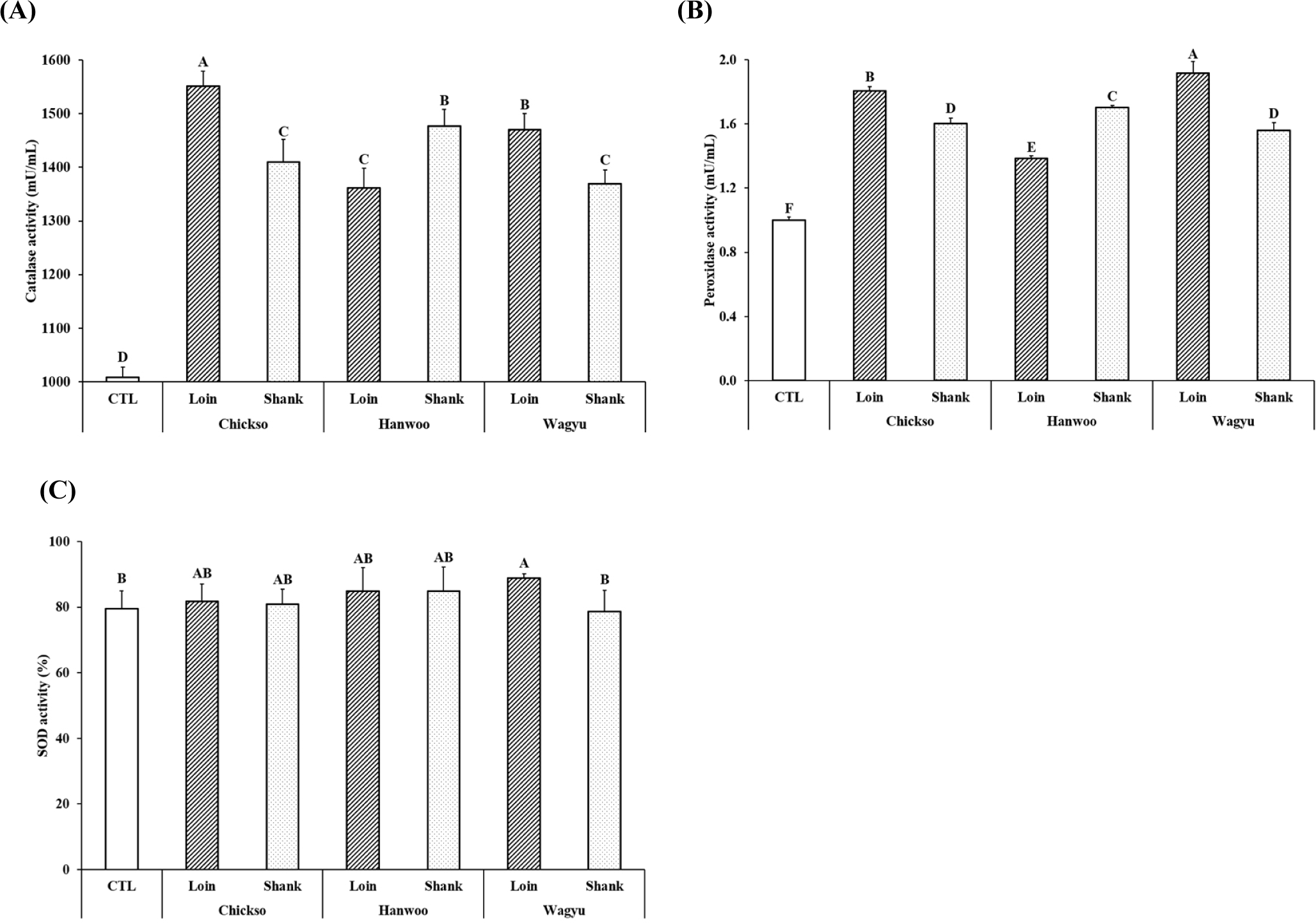
According to previous studies, peptide extracts derived from milk, fish, chicken breasts, and various animal materials are known to have excellent antioxidant activity in the body (Liu et al., 2016; Power et al., 2013; Sakanaka et al., 2005; Sun et al., 2012). In addition, the antioxidant activity of shank was higher than that of loin from Hanwoo (Oh et al., 2016). The study of Kim et al. (2018) demonstrated that the antioxidant octapeptide (Cys-Cys-Cys-Cys-Ser-Val-Gln-Lys; 888.60 Da) isolated and identified from Korean beef extract has high oxygen absorbance capacity. Also, free amino acids detected through the digestion process are known to have a positive effect on enhancing the antioxidant activity of enzymes. Most glutathione is synthesized between cells, and the reduced form of glutathione protects cells by reducing active oxygen (Dringen and Hamprecht, 1999; Gaucher et al., 2018; Levy et al., 1993; Piste, 2013). Lysine can increase the ability to free radical scavenging to protect against oxidative damage by upregulating the expression of antioxidant enzyme genes (Li, 2016). Methionine can act as an endogenous antioxidant, and is an efficient remover of almost all oxidizing molecules under physiological conditions such as H2O2 and hydroxyl radicals (Atmaca, 2004; Levine et al., 1996). The results of the present study also confirm that the consumption of beef-derived peptide extracts can have antioxidant effects on the body. Thus, beef-derived peptide extracts can be used as a pharmaceutical product to prevent cell oxidation.
Fig. 8 shows the antihypertensive activities in mice fed with the peptide extracts derived from the loin and shank of Chickso, Hanwoo, and Wagyu beef. Compared to the control group, which was only fed with general feed, there was no significant activity in any of the treatment groups. These results could be similar to the significant reduction in the ACE inhibitory ability of peptide extracts after digestion to less than the before digestion activity in in vitro digestion experiment (Fig. 6). It was judged that the peptide extracts with antihypertensive activity were digested into smaller peptides due to the mouse intestinal digestive enzymes and microorganisms, resulting in loss of antihypertensive activity (Escudero et al., 2014). The food-derived peptides produced after digestion have been shown to have an antihypertensive effect; however, this effect is reduced when peptides of already active size are consumed. In fact, peptides are known to have low bioavailability because of their weak intestinal mucosal permeability when administered orally (Hamman et al., 2005). Accordingly, the amount of bioactive peptides absorbed after digestion may not have been enough to increase the ACE inhibitory ability detected in the mouse serum, resulting in the non-significant difference between the control and the treatments (Mora et al., 2018).
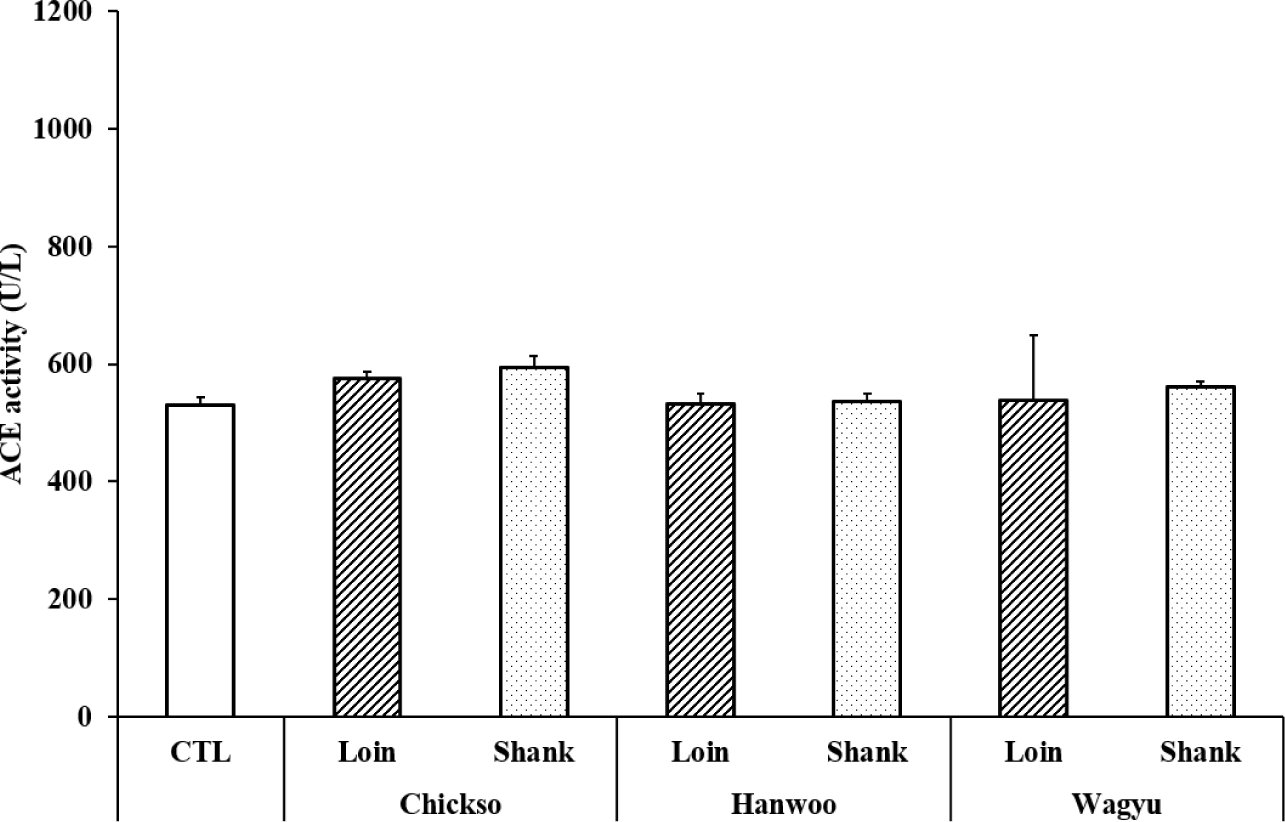
Therefore, additional research is needed to prevent peptides from losing their activity in the body. Furthermore, a previous study showed that peptides must be absorbed in the intestine in an undamaged form to reach the target site even after oral administration (Gianfranceschi et al., 2018). Therefore, research on small-sized peptides (formed from 2–5 amino acids) that can directly pass through the intestinal membrane without digestion and reach the target site (Vermeirssen et al., 2002) is needed.
Conclusion
This study was conducted to analyze the changes in the physicochemical properties and physiological activities of peptide extracts derived from the loin and shank of Chickso, Hanwoo, and Wagyu beef after in vitro and in vivo (in mice) digestion. Specifically, the changes in the molecular weight, amino acid composition, and antioxidant and antihypertensive abilities of the peptides due to the digestion process were analyzed.
The results revealed that there was no significant difference in the digestion rates of Chickso loin and shank peptide extracts during the in vitro digestion process, but the total free amino acid content and antioxidant activity of the Chickso and Hanwoo samples were higher than those of the Wagyu samples. Chickso loin and shank peptide extracts showed a high ACE inhibitory activity. Furthermore, in vivo experiments in mice showed high antioxidant activity in the treatment group fed with Chickso-derived peptide extracts.
Therefore, the peptide extract of Chickso, one of the traditional cattle breeds in Korea, is considered to have better bioactivity than the peptide extracts of Hanwoo and Wagyu. In the future, if research is conducted to maximize the activity of such peptide extracts in the body, it is believed that the genetic resources of Chickso will be valuable and should be preserved. In addition, if a composition with antioxidant and antihypertensive effects is established using conventional beef-derived peptides, it is expected to have applications in preventing, treating, or improving various diseases caused by high blood pressure or free radicals.