Introduction
Curdling is an essential process in the manufacturing of dairy products, such as yogurt and cheese, and the curd is produced by milk protein aggregation. In general, curd is formed by rennet and/or souring. As a result of curdling, soluble whey is mostly removed, and film structured cheese is prepared (Belitz et al., 2009). Casein is the main milk protein that accounts for ~50% of total milk proteins and plays a key role in curd formation. In traditional cheese manufacturing, rennet attacks the primary structure of κ-casein, thereby destabilizing whole casein micelles and promoting aggregation (Foltmann et al., 1979). Whey proteins, which account for the other majority of milk proteins, are rich in β-lactoglobulin (β-Lg) and α-lactalbumin (α-La) (Hernandez-Ledesma et al., 2005). These proteins are relatively stable under processing conditions, such as high temperature; thus, whey proteins rarely take part in aggregation caused by rennet or acids unless the milk is subjected to thermal treatment or exposed to calcium ions (Hinrichs, 2001). Consequently, the final cheese yield significantly depends on the degree of casein aggregation.
High pressure (HP) is an effective processing technology in protein-based food production. The impacts of HP on texturization and modification of cheese, tofu, surimi and restructured meat have been well documented (Cozzolino and Pierro, 2003; Hong et al., 2008; Hwang et al., 2007; Zhang et al., 2005). Structural unfolding and hydrophobic aggregation are involved in pressure-induced structural changes of food proteins (Messens et al., 1997). In past decades, HP research regarding protein gelation has been conducted under chilled, ambient or high temperature conditions. Recently, research interests have shifted toward high pressure low temperature (HPLT) processing due to its impact on protein gelation and technical development. In this case, research has focused on pressure-shift freezing (PSF), which has been recognized as a novel and extremely rapid freezing technique (Benet et al., 2004). Additionally, foods can be preserved in a supercooled state under high pressure and very low temperature, which is defined as high pressure sub-zero temperature (HPST) processing (Ritz et al., 2008). Nevertheless, the impact of HPLT processing on protein structure and gelation properties has been varied.
Microbial transglutaminase (TGase) is a protein cross-linking enzyme that promotes acyl transfer reactions (Belitz et al., 2009). This enzyme has a broad range of activity under different pH, temperature and pressure conditions. Lauber et al. (2001) reported decreased TGase enzyme activity after treating milk at >400 MPa and 60℃ for 20 min. Most food proteins act as a substrate for this enzyme; in particular, casein has been revealed to be extremely susceptible to TGase. Cozzolino and Pierro (2003) demonstrated that cheese prepared by whey incorporation with TGase produced greater yields and harder cheese textures. Therefore, utilizing TGase in the curdling process has potential advantages.
Glucono-δ-lactone (GdL) is a neutral cyclic ester of gluconic acid that is a generally recognized as safe (GRAS) food additive. GdL dissolves easily in aqueous solutions and slowly hydrolyzes to gluconic acid. GdL hydrolysis decreases solution pH values. GdL can be used to sour and acid-set protein gelation (Lucey et al., 2000; Ngapo et al., 1996). Therefore, this study investigated the effects of HPLT processing as well as TGase and GdL on the aggregation abilities of skim milk.
Materials and Methods
Commercial skim milk powder (Maeil Dairies Industry Co. Ltd., Korea) consisting of 35% protein, 52% carbohydrate, 1% fat and 1.5% ash was purchased from a local market. The milk powder (200 g) was suspended in 800 mL of distilled water (7% total protein) and dissolved at ambient temperature (~18℃) for 20 min. Aliquots of 50 mL of solution were packaged in poly nylon pouches and used as a sample stock. The sample stocks (total of 15 packages) were maintained at 4℃ prior to treatment (within 3 h). TGase (Activa TG-B, 60 Unit g−1 protein) was kindly donated by Ajinomoto Korea Inc. (Korea). All chemicals used in this study were analytical grade.
The sample stock packages were divided into five groups of three packages each. One group was used as the fresh control. Another group was frozen at −50℃ for 24 h (atmospheric freezing treatment, AF) and thawed in running water for 20 min.
The remaining three groups were pressurized by three types of processing using a lab-assembled HP system described in our previous study (Hong et al., 2008). In brief, the system consisted of a pressure vessel (300 mL working volume), pressure intensifier (HSF-300, Haskel International Inc., USA), temperature controller (FP80, Julabo Labortechnik GmbH, Germany) and mobile corder (MV104, Yokogawa Co., Japan). Ethanol was used as the pressure-transmitting medium. The compression and depression rates were 2.7 and 20 MPa/s, respectively.
For HPST treatment, one group of packages was pressurized at 200 MPa and 4℃, and then the vessel was cooled. Once the inner vessel temperature reached −20℃, the vessel was heated until the inner vessel temperature reached 4℃. Finally, the pressure was released, and the total pressure-holding time was estimated (154 min). HP treatment was conducted by pressurizing another group of milk packages at 200 MPa and 4℃ for 154 min. Both sample packages were maintained at 4℃ for 24 h. The final group of packages was used for PSF treatment. The sample packages were pressurized at 200 MPa and 4℃, and the vessel temperature was cooled until the inner vessel temperature reached −20℃. When the targeted temperature was reached, the pressure was released. Immediately after depression, the packages were maintained at −50℃ for 24 h and then thawed in running water for 20 min.
To evaluate the susceptibilities of skim milk to TGase, the treated sample stocks were again separated into two groups. One group was mixed with one volume of 4% TGase preparation (in distilled water, w/v), which yielded a final concentration of 3.5% milk protein and 2.4 Unit g-1 of enzyme in the sample. The other groups were mixed with 4% TGase preparation, as described above, but including a final concentration of 0.5% glucono-δ-lactone (GdL, w/v) to test the TGase susceptibility during acidification. All of the sample mixtures were incubated at 37℃ for 5 h. TGase and GdL were dissolved immediately prior to use. For replicate measurements, the above procedure was repeated three times using a new batch of skim milk stock as well as fresh TGase and GdL solutions on different days (n=3).
The pH values of the samples were determined using a pH meter (S220, Mettler Toledo GmbH, Switzerland). The pH values were measured at 0 h (immediately after adding TGase) and 5 h after incubation by directly inserting the electrode into the sample.
A total of 2 mL of each sample was transferred to a tube and centrifuged at 10,000 g for 3 min. The protein concentration in the supernatant was measured by the Biuret method (Gornall et al., 1949). The solubility of the treatment was calculated based on the percentage of the ratio of soluble proteins over total proteins.
Each sample was transferred into a transparent plastic holder, and the lightness of the sample was measured using a color reader (CR-10, Konica Minolta Sensing Inc., Japan) with an 8 mm measuring diameter and 8° illumination angle (CIE standard illuminant D65). CIE L* values were determined as indicators of lightness. Calibration was performed using a white standard plate before detection.
The viscoelastic moduli of the treatments were determined using a rheometer (Anton Paar GmbH, Austria) equipped with a TU1 plate (Anton Paar GmbH). Immediately after the TGase reaction, the milk samples were loaded onto the sample cell and covered with a cap to prevent evaporative loss and equilibrated at 30℃ for 1 min prior to scanning. The samples were oscillated at a frequency of 0.1 Hz and 0.628 strain at 30℃. Measurements were taken every 5 min for 5 h.
Sodium dodecyl sulfate-polyacrylamide gel electrophoresis (SDS-PAGE) was conducted using an electrophoresis system (EzCell, KOMA Biotech Co., Korea). The TGase-reacted milk treatments were sampled at 1 h incubation time intervals, centrifuged at 10,000 g for 3 min at ambient temperature, and the supernatants were mixed with the same volume of sample buffer (aspartate sample buffer, KOMA Biotech Co., Korea) composed of 0.15 M Tris-HCl (pH 8.45), 12% glycerol, 2% SDS and 0.003% coomassie blue G. To reduce proteins, 5% (v/v) β-mercaptoethanol was also added to the sample buffer. The sample mixture was boiled for 3 min, and 20 μL of the sample was loaded onto a 4-12% acrylamide gradient gel (Tris-Glycine-PAG Pre-case gel, KOMA Biotech Co., Korea). Peptide separation was conducted under a constant voltage of 150 V.
A completely randomized block design was adopted in the present study. The data obtained from three replicate experiments were analyzed by the general linear model using the SAS program (Ver. 9.0, SAS Institute Inc., USA). When the main effect was statistically significant (p<0.05), the means were separated by Duncan’s multiple range test.
Results and Discussion
The pH of skim milk showed different patterns depending on the applied processing type (Table 2). The AF treatment exhibited a pH of 6.67, which was slightly lower than the 6.69 pH of the control, although the pH of AF was not significantly different than the control. Meanwhile, all pressurized treatments had significantly higher pH values compared with the control, which ranged from 6.75-6.80 (p<0.05). Among the pressurized treatments, the pH values of the HP and HPST treatments (6.75-6.77) were lower than the 6.80 pH of the PSF treatment (p<0.05), whereas the pH values of HP and HPST were not significantly different. The pH changes in the milk were due to protein destabilization. After reacting with TGase for 5 h, a significant decrease in pH was observed in all of the samples except for the HPST treatment samples (p<0.05). In addition, the pH patterns of the samples activated by TGase were similar to those patterns prior to TGase reaction (0 h). However, the pressurized samples showed no significant differences. After reacting with TGase and GdL, the pH values of all samples were lower and ranged from 5.66-5.72 with similar patterns, except for the pressurized samples.
In frozen milk, decreased pH values have been attributed to the dissociation and precipitation of salts (Van Den Berg, 1961). Alternately, pressure-induced unfolding of milk proteins results in higher pH values in pressurized milk (Messens et al., 1997). Because protein unfolding is a prerequisite for protein aggregation, pressurization effectively contributes to the curdling process. Based on the pH changes in pressurized treatments, PSF treatment seemed to provide better protein aggregation than the HPST treatment. Proteins have been reported to denature during heating, freezing (cold-denaturation) and pressurization, and HPST processing has a structural impact due to cold- and pressure denaturation (Meersman et al., 2006). Nevertheless, cold-denaturation did not affect protein structure compared with pressure-denaturation, which explains the insignificant difference in pH values between the HP and HPST treatments.
Protein solubility indicated that HPLT (PSF and HPST) was effective at inducing protein aggregation (Fig. 1). Initially, the solubility of milk treated with AF and HP did not differ from that of the control and ranged from 91.7-92.8%. A significant decrease in the solubility of skim milk was observed in the PSF (70.4%) and HPST (80.4%) treatments. In particular, the decreased solubility in the PSF treatment was significant (p<0.05), indicating that PSF and HPST were more effective in inducing protein aggregation. After TGase treatment, the solubilities of the control and AF decreased by approximately 8%. However, the HP, PSF and HPST treatments decreased the solubility of skim milk by more than 20%. In the PSF treatment, the solubility was lower than 50% (49.6%). Adding GdL led to the lowest solubilities of skim milk in all treatments. The control and AF samples showed decreased solubilities that ranged from 72.6-74.1%, and decreases also occurred in HP (66.2%), PSF (45.8%) and HPST (54.1%). AF had a similar degree of solubility as the skim milk control. In contrast, the pressure treatment significantly lowered the protein solubility, especially in the PSF and HPST treatments. The TGase/GdL control treated with PSF indicated that the PSF treatment was more effective at protein aggregation than adding TGase and GdL. The solubility of the control with TGase/GdL was 72.6%, whereas PSF-treated skim milk without any additives showed 70.4% of solubility.
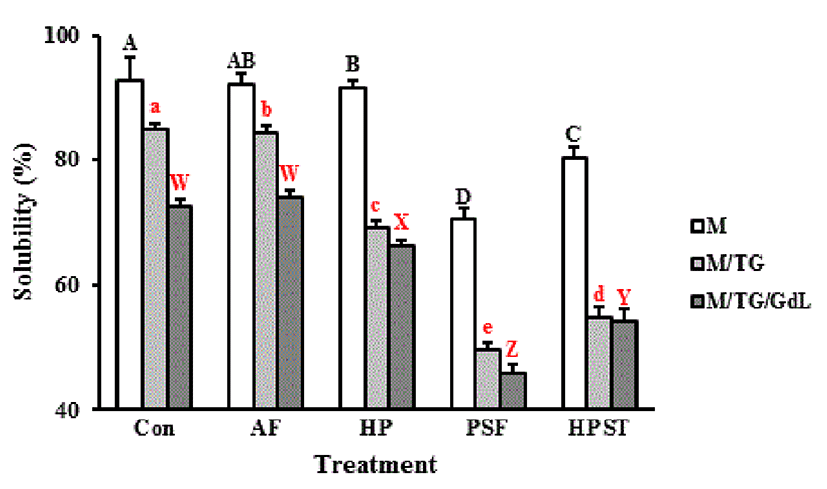
Denaturation that induces whey protein curdling should efficiently lead to an increase in cheese yield (Lopez-Fandiño et al., 1996). Kanno et al. (1998) demonstrated that the solubility of milk proteins decreased with increasing pressures up to 1,000 MPa. HP treatment transforms the three-dimensional conformation of proteins and results in the breakage of electrostatic bonds and hydrophobic interactions, changing the structure and hydration of proteins (Balny et al., 1992). TGase catalyzes the conversion of soluble protein into insoluble high molecular polymers by forming covalent cross-links and modifying the rheological properties of proteins (De Backer-Royer et al., 1992). This protein modification has presumably been attributed to the formation of ε-(γ-glutamyl) lysyl bonds in milk proteins, which results in curdling (Færgemand et al., 1998; Imm et al., 2000). In good agreement with this, the solubility of skim milk decreased after TGase activation, especially in the pressurized milk samples. We assumed that there were synergetic effects between pressurization and TGase activation on milk curdling.
The gap in solubility between TGase and TGase/GdL treatments was narrowed in the pressure-treated samples, which was caused by β-Lg denaturation that resulted from the high pressure treatment of the milk. β-Lg has a number of thiol groups for which the reactivity increases at alkaline pH and decreases at acidic pH (Arias et al., 2000). For this reason, high pressure treatment with the addition of GdL resulted in an acidifying milk property that was not an effective condition.
The L* values of the samples had similar patterns of protein solubility in all treatments (Table 1). The control and AF samples had L* values of approximately 80 in all treatments, while the PSF and HPST samples had lower values than the others. For PSF and HPST, TGase and GdL treatments decreased the L* values of skim milk, which induced protein aggregation. The L* value of HPST without additives was 69.08 and decreased to 67.60 and 58.56 with TGase and GdL activation treatments, respectively. The L* value of PSF, which decreased from 68.49 to 55.74 and 48.67, depending on the additive, was the lowest value of all of the treatments. Considering the solubility of skim milk, the L* value was influenced by protein aggregation. PSF had the lowest L* value and also had the lowest solubility.
HP treatment induces disintegration of casein micelles, which impart the white color in milk, to smaller casein particles, which decrease the lightness of milk by increasing the translucence of milk (Johnston et al., 1992; Ledward et al., 1995; Oberg, 1986). Casein micelles consist of many caseins bound by calcium phosphate, which contribute to light scattering. When HP treatment disrupts non-covalent forces, such as hydrogen bonds, ionic interactions and hydrophobic forces move individual caseins and calcium phosphate apart, causing light scattering to decrease, which affects L* values (Adapa et al., 1997). Considering that L* values and protein solubilities have similar tendencies, curdling due to TGase activation probably affected the color change as well as the solubility of milk, particularly decreasing the L* values by modifying casein micelles, as previously stated. Gaucher et al. (2007) observed that the lightness of skim milk decreased from 69 to 48 upon adding phosphate, forming an insoluble calcium phosphate salt that decreased the solubility of casein molecules, changing the pH to 6.80.
As depicted in Fig. 2, the soluble fraction of skim milk showed four major peptide bands and several minor bands. Low molecular weight (Mw) bands of ~10 and 15 kDa were estimated to be α-lactalbumin and β-lactoglobulin, respectively. In addition, bands at Mws of ~22 kDa were likely β- and κ-casein, whereas the band at ~27 kDa was αs-casein (Banker and Cotman, 1972). Upon physical treatments, such as freezing or pressurization, whey proteins were not affected; thus the bands maintained their intensity. The β- and κ-caseins were also not affected by the physical treatments, whereas αs-casein completely disappeared after PSF treatment, reflecting a relationship between the low solubility and high G' of PSF-treated skim milk. Casein micelles have been reported not to dissociate under pressures < 200 MPa (Huppertz et al., 2004), which was also confirmed in this study. However, the reason why PSF attributed to the aggregation of skim milk proteins was not fully understood in this study. Micelle dissociation and Ca2+ liberation likely occurred during PSF processing. These phenomena were not involved in HPLT processing in this study. Consequently, curd formation during PSF resulted from both pressurization and water phase transition.
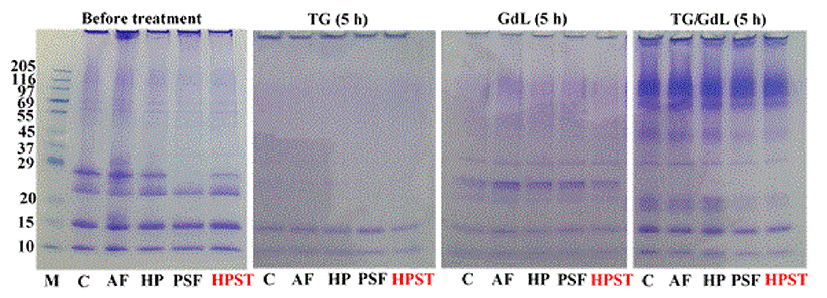
After TGase action, all casein bands completely disappeared, and whey proteins also lost their band intensities, which indicated that casein was a good substrate for TGase (Kuraish et al., 1997). Furthermore, TGase-mediated curdling was involved in the coagulation of casein with partial whey proteins. Alternately, acidification showed different SDS-PAGE profiles in which partial α-casein was still soluble, while β- and κ-casein bands significantly decreased in intensity, which was similar to the results of Lefebvre-Cases et al. (2001). The results indicated that either TGase or GdL affected the coagulation of skim milk proteins, and the effects of physical processing were not different upon enzymatic and acidified treatments. Similar patterns were also observed in an experimental combination of TGase and GdL. The SDS-PAGE pattern of the combined treatment revealed that most caseins disappeared, while low (~20 kDa) and high Mw bands (69-97 kDa) were generated. As reviewed by Kashiwagi et al. (2002), TGase action in acidic conditions causes deamidation and reorganization of proteins. Consequently, the results indicated that caseins play a key role in protein coagulation, and further exploration should be conducted to clarify the mechanisms involved in PSF-mediated milk protein coagulation.
Pressure treatment leads to substantial modification of milk (Anema et al., 2005). To investigate the viscoelastic properties of skim milk with various pressures and freezing treatments, oscillation tests were performed. Fig. 3. shows the change in storage modulus (G') 5 h after adding TGase to skim milk. Within 60 min, G' fluctuated after TGase activation, indicating that molecular cross-links had been formed in the skim milk. After the 5 h test, PSF had the highest final G' value of 19.8 Pa, whereas HPST only measured 0.24 Pa. Other treatments, including the control (0.02 Pa), AF (0.01 Pa) and HP (0.01 Pa) revealed values less than 0.1 Pa. A similar tendency was observed when skim milk was activated with TGase and GdL. PSF and HPST had higher G' values than the other treatments. AF showed a final G' value of 0.02 Pa 5 h after activation by TGase and GdL, whereas the control and HP had values lower than 0.01 Pa. HPST showed a value of 1.60 Pa after 5 h with TGase and GdL, which was approximately a seven-fold increase with TGase/GdL; however, PSF had a G' of only 1.11 Pa with TGase/GdL.
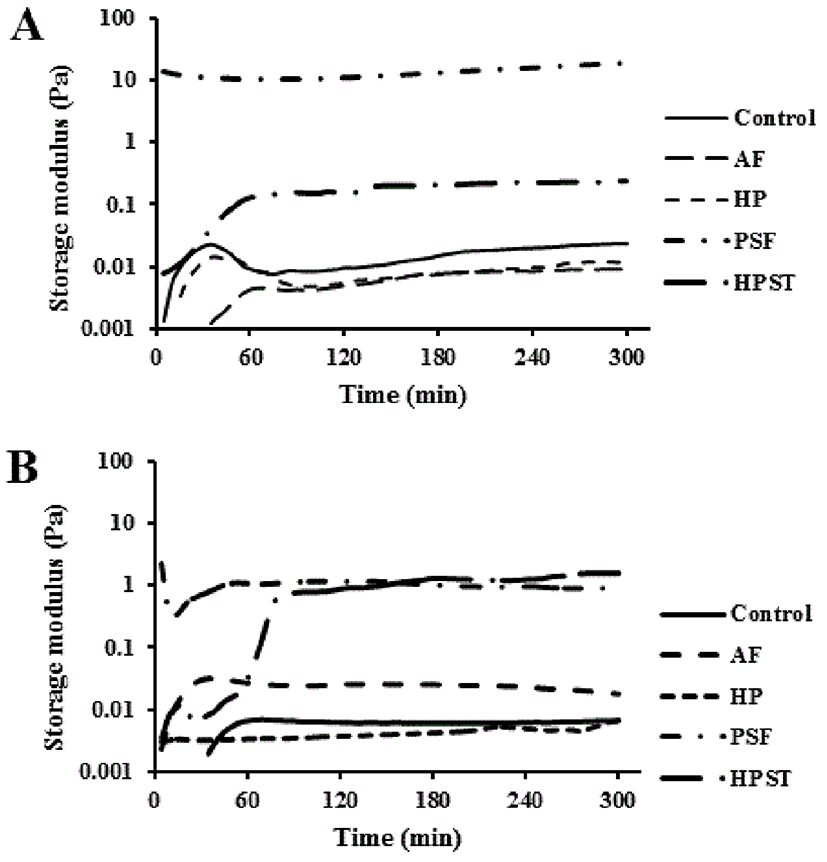
Previously, when milk gels showed a G' > 1 Pa, gelation was designated as the milk phase (Lucey et al., 1997). According to this criterion, only PSF and HPST were effective at curdling milk. TGase-treated PSF was the best treatment for curdling milk with the highest G' value. Freezing milk induced changes in its physical properties, such as protein flocculation and off-flavor development caused by ice crystal formation (Webb and Arbuckle, 1977). These changes affect the yield and quality of cheese and yogurt (Wendoff, 2001). While conventional freezing produces large ice crystals, PSF can produce a smaller number of ice crystals, implying less changes in physical properties. TGase treatment of milk yielded acid gels with increased stiffness compared with untreated milk that was caused by modification of protein networks through covalent cross-links in the milk system (Færgemand et al., 1998). PSF and TGase treatment of skim milk appeared to have a synergetic effect on curdling.
A few previous reports on the effect of milk gels derived from GdL activation on the rheological properties of milk have shown different results. Anema (2008) reported a decrease in the final G' values of milk gels acidified with GdL as the activation time decreased. However, Horne (2003) showed that G' values increased with decreasing GdL activation times. Considering the gel electrophoresis results in this study, while adding TGase/GdL induced more protein aggregation than the absence of GdL, TGase treatment led to the formation of harder curds without adding GdL, except in the HPST treatment.
Conclusions
Pressure treatments induced a significant pH increase in milk, and adding GdL decreased the pH values in all samples by approximately one unit due to the hydrolysis of GdL into gluconic acid. Pressurization also decreased the lightness of milk, especially during PSF treatment due to disintegration of casein micelles, which increased the translucence of the milk. While TGase and GdL activation also decreased lightness, the lightness of the control and AF changed by less than three units of lightness in all treatments. In all treatments, TGase and TGase/GdL treatments decreased the protein solubility of skim milk due to protein aggregation. PSF and HPST were effective curdling processes. In particular, PSF-treated milk was the least soluble, regardless of TGase and GdL additions. In the absence of TGase and GdL, PSF showed approximately 70% solubility, which was similar to the solubilities of the control with TGase and GdL. PSF had the weakest bands corresponding to κ-casein, which indicated that casein contributed to curdling. After TGase/GdL activation, thick bands corresponding to masses higher than 69 kDa were observed in all treatments, revealing protein aggregation. Regarding the rheological properties of treated skim milk, PSF had the highest G' value after TGase activation. Contrary to the protein solubility and gel electrophoresis results, the G' values of the curd from all of the treatments except HPST decreased in the presence of GdL. This phenomenon showed that GdL induced more protein aggregation than the absence of GdL. In conclusion, PSF was the most desirable processing method of skim milk for cheese manufacturing due to its curdling efficiency.