Introduction
The whey industry has a short history but continues to innovate, focusing on finding new uses and new markets for value-added ingredients. Whey manufacturers are capable of producing numerous whey products by adjusting protein levels, minerals, lactose contents, functional properties, and other specifications. Besides the functional properties like water binding, forming gels, emulsifying, foaming, fat binding, color and texture enhancing in food products (Jeewanthi et al., 2015a), whey proteins (WPs) have numerous nutritional properties which concern by food manufacturers. WPs contain a relatively high level of essential amino acids with a high nutritional value and are an important source of bioactive sequences (Korhonen, 2002; Silvestre et al., 2012). There has been a growing interest in recent years in bioactive peptides derived from WPs. Such peptides have been found to express various bioactivities with protective functions such as reducing allergenicity, regulation of digestion, nutrient uptake, and metabolic or physiological regulation. These biologically active peptides are inactive within the sequence of parent protein and release during mechanical or chemical cleavage.
The term bioactivity refers to food components that can affect biological processes or substrates and hence have an impact on body function or condition and health. Whey-based peptides have demonstrated activity that may reduce both hypertension and dyslipidemia. Literature has reported that bioactive whey peptides are involved in angiotensin-converting enzyme (ACE) inhibitory activity, opioid-like activity, antithrombotic activity, and cholesterol-reducing activity (Gerdes et al., 2001). ACE-inhibitory activity is currently among the most documented bioactivity of whey peptides (Gauthier et al., 2003). ACE is involved in hypertension, and its level is an important risk factor in the development of heart and vascular disease. In the human body, ACE converts the inactive peptide angiotensin I into the vasoconstrictive peptide angiotensin II and inactivates bradykinin (Meisel and Schlimme, 1996). The blockage of ACE and prevention of the amount of angiotensin II in the blood result in a decrease in blood pressure in hypertensive patients. β-lactoglobulin (β-Lg) is responsible for the majority of bioactive properties of WP products (Otte et al., 1996). Bioactive peptides have been found in the enzymatic hydrolysates of β-Lg and α-lactalbumin (α-La).
Several studies have suggested the need for rapid and cost-effective methods for the production and isolation of bioactive peptides from whey and that can also be used on a larger industrial scale (Tavares and Malcata, 2013; Zambrowicz et al., 2013). Bioactive peptides, including those that are inactive within the sequence of the parent protein, can be released in three ways: enzymatic hydrolysis, fermentation of milk with proteolytic starters, and proteolysis by microorganisms (Foegeding et al., 2002). Researchers have shown that limited enzymatic hydrolysis of WPs can result in improved functionality and biological activities (Pouliot et al., 2009; Spellman et al., 2009). Food industry uses several enzymes for food production. However, using particular enzymes for an each food has a great influence for the food quality.
WPC-35 is a protein source which available in market at a cheaper cost compared with the products of other WPC percentages or isolates. Adjusting the protein content of 35% from fresh whey needs less processing. This concentration of WPs widely use in food industry for baby foods, dairy foods, bakery products, confectionaries, chocolates, nutritional supplements, tablets, and as a replacer of skim milk. This study was aimed at identifying an enzyme that encompassing bioactive peptides and ACE-inhibitory effect of WPC-35 hydrolysates, out of six different food-grade enzymes which are using currently in food manufacturing industry. Moreover, this study concerned on identifying the most appropriate whey protein hydrolysates (WPHs) which can be added to food as long time hydrolyzed protein or partly purified peptides for formulation of a wide range of functional food products in health attributes.
Materials and Methods
WPC was produced using the method of Jeewanthi et al. (2014). Fresh mozzarella cheese whey remained after mozzarella cheese production at the lab was clarified and subjected to heat treatment at 70°C in a vat for 5 min after adjusting the pH to 7 from 6.32, followed by cooling to 50°C. Ultrafiltration (UF) was carried out with inlet and outlet pressures of 6 bar and 2 bar, respectively. The membrane module used was equipped with GR-60-PP flat sheet UF membranes (Danish Separation Systems AS, Denmark). The molecular weight cut-off range was 10 kDa. This experiment was carried out in a spiral wound-type module (DSS LabUnit M20, Alfa Laval Nakskov, Denmark), and the total effective surface area of the membranes was 0.036 m2. After filtration, the permeate was removed, and the retentate was subjected to spray drying using the B-191 Mini spray dryer (BUCHI, Switzerland) in order to obtain dried WPC at a protein content of approximately 35%. The volume reduction of whey was 75% to obtain WPC-35 (Jayaprakasha and Yoon, 2005). The following formula was used to calculate the volume reduction. The inlet and outlet air temperatures were 175°C and 75°C, respectively.
The protein content was measured by the Kjeldahl method (nitrogen factor 6.38), and fat by the Röse-Gottlieb method (AOAC, 2000). Moreover, total solid, ash, and lactose contents were determined according to the methods of AOAC (2000).
WPC-35 hydrolysates (WPHs) were produced according to the method of Jeewanthi et al. (2014). The enzymes were added to a 10% WPC-35 solution at a ratio of 1:25 (w/v) and placed in a shaking incubator (VS-8480S, Vision Scientific Co., Ltd., Korea) at 37°C. Aliquots of each enzyme solution were collected at 0.5, 1, 2, 3, 4, and 5 h while shaking at 180 rpm. The aliquots were then placed in a 95°C water bath for 10 min to inactivate the enzymes and cooled to room temperature. The mixture was centrifuged at 3,000 rpm (Combi-514R, Hanil Science Industrial Co., Ltd., Korea) for 30 min, and the upper solution was dried completely using a freeze dryer (FDU-1200, EYELA, Japan) for 24 h. The dried powder was then sealed and refrigerated.
The commercial enzymes used were alcalase 2.4L (Novozymes, Denmark, EC 3.4.21.62), protease M (Amano Enzyme, Japan), protease S (Amano Enzyme, E.C. 3.4.24. 28), trypsin (Novozymes, Denmark, EC 3.4.21.4), α-chymotrypsin (Sigma-Aldrich Co., USA, EC 3.4.21.1), and pepsin (Wako Pure Chemical Industries Ltd., Japan, EC 3.4.23.1).
Mini-PROTEAN tetra electrophoresis, which includes BIO-RAD gel electrophoresis system, was used to evaluate peptide formation of the hydrolysates. In brief, 10 mg of sample was dissolved in 1 mL of sample buffer (Sigma-Aldrich Co., USA) for 5 min at 100°C to minimize protein denaturing. Each sample was then stained with coomassie brilliant blue G (Sigma-Aldrich Co., USA). Distinct bands within the gel appeared after running WPH in a polyacrylamide gel. Seeblue plus2®pre-stained standard was used as the reference material. Degree of hydrolysis (DH) was estimated using 2,4,6-trinitrobenzene sulfonic acid (Sigma-Aldrich Co., USA) (Jeewanthi et al., 2015b).
The ACE-inhibitory activity of WPH was measured using a modified method, which used by Lim et al. (2011). ACE solution was prepared by stirring 1 g of rabbit acetone lung powder (Sigma-Aldrich Co., USA) with 10 mL of 0.1 M sodium borate buffer containing 0.4 M sodium chloride at 4°C for 24 h. From this solution supernatant was used after centrifuge (Combi-514R, Hanil Science Industrial Co., Ltd., Korea) for 30 min at 12,000 rpm. WPHs 10% solution sample of 50 μL was mixed with 100 μL of 0.1 M sodium borate buffer (pH 8.3). Then 50 μL of ACE solution was added and incubated at 37°C for 5 min. In addition, 50 μL of Hip-His-Leu solution (Sigma-Aldrich Co., USA) was added and incubated for 30 min. To stop the enzymatic reaction, 250 μL of 0.5 N HCl was added. Produced hippuric acid was extracted by vortexing for 15 s after addition of 1.5 mL of ethyl acetate. One milliliter of supernatant was transferred in to a clean glass test tube after centrifuging for 2,500 rpm for 10 min. Then the tubes were placed in dry oven at 100°C for complete evaporation and remained brown ring was dissolved well using 3 mL of deionized water. Each sample’s absorbance was measured using a 228 nm spectrophotometer (OPTIZEN 2120UV, Mecasys Co., Ltd., Korea). This experiment was performed in triplicate and the results are the average of three values. The ACE inhibitory activity was expressed in two ways, as a percentage of inhibition and IC50 value, which is defined as the concentration of WPC hydrolysates (mg/mL) necessary to reduce the activity of this enzyme at 50%.
The following formula was used to calculate the percentage of ACE-inhibitory activity:
A Q-Star mass spectrometer (Applied Bio-systems/MDS SCIEX, Canada) was used for the analysis of bioactive peptides of 3 h-hydrolyzed WPH by trypsin and protease S. The method used for analysis was the offline electrospray-ionization-mass (ESI/MS), which was identified by Schober et al. (2012) from their previous studies, as a suitable method for analyze of WPHs. The aliquots were diluted with acetonitrile at a ratio of 1:1. Each prepared diluted sample of 10 μL filled into nano ESI emitters (GC 120 F-10, DNU MS, Germany). The spray voltage was gradually increased to 1,600 volts, and the flow rate of the ionized molecules was analyzed at 2-200 μL/min.
Mean values and standard deviation were measured using the SAS software package. One-way analysis of variance (ANOVA) and Duncan’s multiple range test (p<0.05) were used to establish the significance of the differences. The peptide probability was calculated according to mascot probability scores (http://www.matrixscience.com). It was calculated the scores as −10 × Log (Peak value).
Results and Discussion
Usage of UF and spray drying methods to manufacture WPC-35 has a relatively easy scale-up, comparing to other membrane separation techniques or preparative chromatographic techniques. Short time heat treatment of this method provides an appropriate background to study the performance of WPH since the bioactive peptides remain intact and the WPs remain non-denatured manner. The protein concentration of 35% was able to achieve within a less processing time and inexpensive status due to minimal utilization of energy and time as well as its easy scale up, ease of membrane exchange, by contrast with other commercially available higher protein concentrations.
The following nutritional parameters were determined for the general compositions. The components of manufactured WPC-35 were 34.94% protein, 53.32% lactose, 3.50% fat, and 3.24% ash. The protein content of permeate was lesser than 0.1% along the UF process. The scientific study of Weigle et al. (2005) has been reported that an isocaloric high-protein diet with 30% protein, 20% fat, and 50% carbohydrate was markedly increased satiety and decreased energy intake, body weight, and body fat. Lactose is the major component of WPC-35 which considers as the least valuable component. The optimum results in high protein diets which aimed at weight reduction are achievable when intake is more balanced with carbohydrate (Luhovyy et al., 2005). Relatively lactose has a low glycemic index compared to the other carbohydrates in food and it is hard to utilize. This reason upgrades the value of lactose on top of the other health benefits such as increase mineral absorption, contribute as a probiotic and a prolonged energy supplier. Calcium, magnesium, and phosphorous are the major minerals available in WPC and zinc (Jelen, 1992) is available in trace amounts.
WPC is a complex blend of individual proteins. Compositional patterns were produced from the electrophoresis of WPHs, and the hydrolysate from protease S and pepsin can be seen in Fig. 1. In intact WPCs, the bands appeared in the range of 50-98 kDa, 16-22 kDa, and 6-16 kDa. It was shown that BSA (66.4 kDa), β-Lg (18 kDa), and α-La (14 kDa) are the distinct proteins of intact WPCs. Damodaran (1996) has reported that β-Lg remains as a dimmer with a molecular weight of 36 kDa between pH values 5.2-7.2. The pH values of all experimented WPHs solutions were laid between these ranges except pepsin hydrolysates. The pH values of pepsin hydrolysates were 2.94-2.63 (p<0.05) at 0-5 h hydrolysis (data not shown).
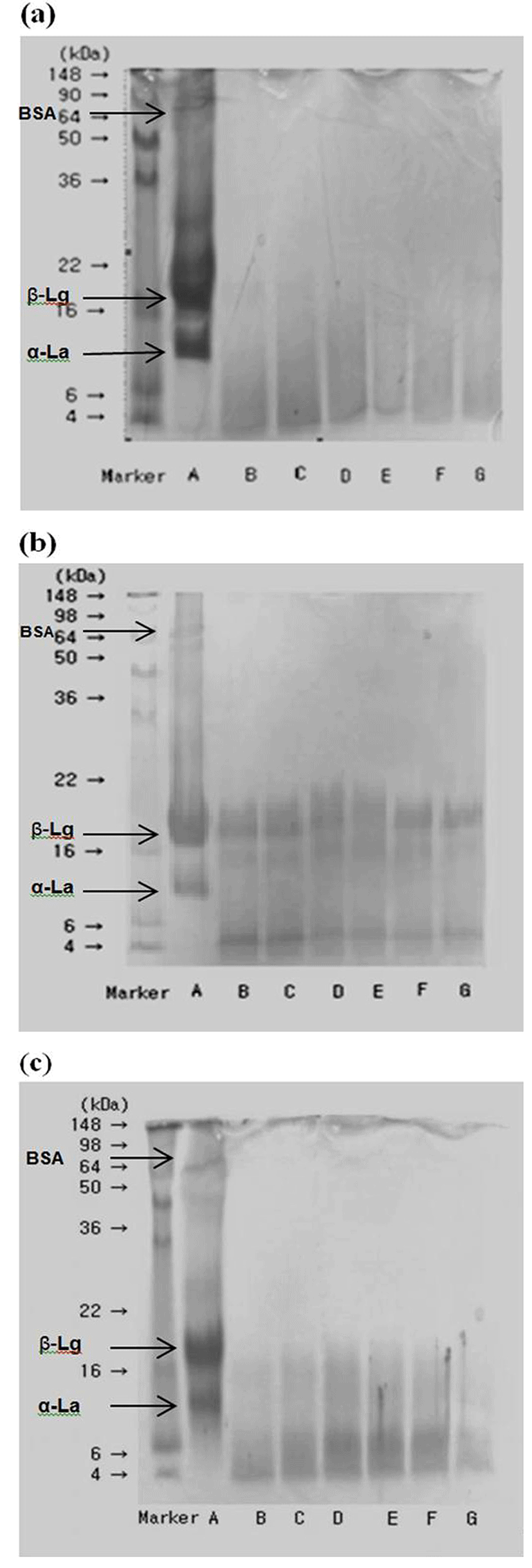
α-La is the second most abundant protein in WP. It is a compact globular protein with a similar structure and composition to human milk proteins. α-La considers as an important protein in WPH supplemented nutritional foods due to Cys, Ile, Leu, Trp, and Val residues. The structure of this protein is highly heterogeneous, α-helix forms with weak hydrophobic interactions.
Depending on the type of enzyme, the protein bands were located in different locations; however, the 2 h hydrolysis samples of every enzyme showed a common pattern in bands, where the degraded peptides commonly appeared between 16-22 kDa and 6-16 kDa. Protease S showed the highest proteolytic activity of the selected enzymes of WPC-35 (Fig. 1(a)), while pepsin showing the least (Fig. 1(b)). Our previous experiments showed that the protease S had the second highest DH among these enzymes for WPC-35 as 9.96-11.2% (hydrolyzed for 0.5-5 h) (Jeewanthi et al., 2014). In WPC-50 and WPC-60 (whey protein concentration with 50% and 60%), DH for protease S was reported in-between 26.4-28.77% (hydrolyzed for 0.5-5 h) and superior to enzymes named flavourzyme, protease A, protease M, and pepsin (Jeewanthi et al., 2015b). The results indicate that pepsin is less capable of hydrolyzing β-Lg within 5 h and available at a higher purity. DH of pepsin was reported as 8.26-10.34% for 0.5-5 h hydrolysis of WPC-35. In trypsin hydrolysis samples, the β-Lg and α-La contents decreased over time (data not shown). After 4 h, β-Lg was no longer detectable and much less α-La was observed. β-Lg was more rapidly broken down by trypsin and less rapidly by pepsin compared to α-La of whole whey (Pintado and Malcata, 2000). At the beginning of the reaction, trypsin partially hydrolyses β-Lg, although α-La and BSA are more resistant to hydrolysis (Custodio et al., 2005). All of the experimental results above agreed with the results. Trypsin was exhibited 8.78-11.23% of DH% (hydrolyzed for 0.5-5 h). Alcalase showed the second highest proteolytic activity, and peptide bands were found between 4-10 kDa (Fig. 1(c)). Chymotrypsin reacted with most of the β-Lg, visualized as increasingly weaker bands with time, and with little of the BSA (data not shown).
The preferred ACE inhibitors are those peptides that contain dicarboxylic amino acids in the N-terminal position and branched-chain amino acid residues, such as Val and Ile (Wu and Ding, 2002), while the C-terminal position is represented by hydrophobic amino acids such as Trp, Tyr, Phe or Pro (Yust et al., 2003). However, ACE shows poorer affinity when dicarboxylic amino acid in final position or residue Pro comes before the last position (Tavares and Malcata, 2013). ACE inhibiting peptides which have antihypertensive properties have been released through an enzymatic treatment of bovine β-Lg and WPCs (Wang et al., 2010). The calculated values for the ACE-inhibitory effect of different WPHs are shown in Table 1. The results showed 6.06% (IC50 = 0.972 mg/mL) of ACE-inhibitory effect in intact WPC-35. Mullally et al. (1997) also reported 10% lesser ACE-inhibitory effect in whey protein isolates (WPIs). Just after the hydrolysis of WPC-35, the values were heavily increased by at least tenfold of their initial value. Among the experimental enzymes, protease S and alcalase hydrolysates showed the strongest ACE-inhibitory effect, while trypsin hydrolysates showed the weakest effect. The experiments of Wang et al. (2010) reported similar results for alcalase followed with neutrase, by showing stronger ACE-inhibitory effect compared to trypsin in hydrolysates of WPIs 5% solution. According to our results, ACE-inhibitory activity can be accelerated by the higher proteolytic activities of the protease S after releasing many amino residues between 2-12 kDa those considering as the most responsible ACE-inhibitor peptides (Lopez-Fandino et al., 2006). With the reason of protease S is a thermostable neutral protease, the WPH solution has given a favorable atmosphere to perform enzyme activity than the other acidic or base enzymes. Alcalase treated WPHs are the second highest score resulted for ACE-inhibitory activity and Fig. 1(c) revealed that those peptides are lies between 4-10 kDa.
1IC50: Concentration of hydrolysates (mg/mL) necessary to the activity of this enzyme by 50%; a-cMeans with the different letters in a same column are significantly different by Duncan's multiple range test (p<0.05).
The pH of WPHs in a 10% solution of every enzyme was ranged between in 6.03-6.22 except pepsin hydrolysates (data not shown). On the other hand, it delayed the activity of trypsin which favors a base environment. Protease M, pepsin, and trypsin showed maximal activity with 3 h hydrolysates. The bioactive peptides responsible for ACE-inhibitory degradation, seemed starts and dominate or inhibit the novel formation of peptides at this level (Meisel and Schlimme, 1996). These results show that the specificity of the enzyme had an obvious effect on the resulting ACE-inhibitory activity besides every enzyme hydrolysates has capability of increasing ACE-inhibitory effect.
The major protein in WPCs is β-Lg which has 162 amino acids. This protein remains its primary structure unchanged in gastric digestion due to resistance of enzymatic or acidic digestion along the passage through stomach (Barros et al., 2001). Hydrolysis of intact structure of β-Lg activates the bioavailability of the peptides and recognition of those is important in food manufacturing industry since it is the major protein in WPHs.
Experimented WPH was selected for peptide analysis, looking at trypsin and protease S hydrolysates (3 h) showed the lowest and highest ACE-inhibitory activity respectively. Peptide analysis of β-Lg results are shown in Tables 2 and 3. These are the enzymes that showed ACE-inhibitory active peptides that fractioned by both ends from the parental proteins as LAMA and LDAQSAPLR within 3 h (least hydrolysis time).
1Peptide probability (PP), > 10, Peptide confidence: high; 2peptide having antioxidant activity; 3peptide having ACE-inhibitory activity.
1Peptide probability (PP), > 10, Peptide confidence: high; 2peptide having antioxidant activity; 3peptide having ACE-inhibitory activity.
They confirmed that protease S generated LDAQSAPLR (Leu-Asp-Ala-Gln-Ser-Ala-Pro-Leu-Arg) in fragment 32-40 and LVLDTDYK (Leu-Val-Leu- Asp-Thr-Asp-Tyr-Lys) in fragment 92-100 from β-Lg with a higher confidence levels. Moreover, in β-Lg, the hydrolysates by trypsin generated LAMA (Leu-Ala-Met-Ala) with a high confidence value, VAGTWY (Val-Ala-Gly-Thr-Trp-Tyr) and LDAQSAPLR. The peptides LAMA, LDAQSAPLR, LVLDTDYK, and VAGTWY have been identified as ACE-inhibitory peptides (Pihlanto-Leppala et al., 2000).
The presence of hydrophobic amino acids which Ala (A), Gly (G), Ile (I), Leu (L), Phe (F), Trp (W), Tyr (Y), and Val (V), and unique amino acids such as Pro (P) and Gly (G) in a peptide sequence increase the lipid inhibitory activity (Hermansen, 2000). The peptides LVLDTDYK (Leu-Val-Leu-Asp-Thr-Asp-Tyr-Lys), TKIPAVFK (The-Lys-Ile-Pro-Ala-Val-Phe-Lys), WYSL (Trp-Tyr-Ser-Leu), and VAGTWY has been reported as high radical scavenging active peptides (Conway et al., 2013; Zhang et al., 2013). VAGTWY, TKIPAVFK, and WYSL are shown in trypsin treated WPHs, while TKIPAVFK and LVLDTDYK in protease S treated hydrolysates. The peptides AASDISLLDAQSAPLR (Ala-Ala-Ser-Asp-Ile-Ser-Leu-Leu-Asp-Ala-Gln-Ser-Ala-Pro-Leu-Arg), PAVFK (Pro-Ala-Val-Phe-Lys), VLVLDTDYK (Val-Leu-Val-Leu-Asp-Thr-Asp-Tyr-Lys), and VAGTWY have been reported to hold antibacterial effects (Exposito and Recio, 2006). Protease S treated hydrolysates of β-Lg consisted VLVLDTDYK peptides and did not appear in trypsin WPH. Both peptides AASDISLLDAQSAPLR and PAVFK observed in both hydrolysates, approves the presence in antibacterial effects of hydrolyzed WPHs of both samples. The more proteolytic active enzymes with WPC are able to release these peptides of interest, resulting in more bioactivity of the peptides. WPHs can be used as a complementary treatment in combination with lifestyle modifications, such as weight reduction, exercise, and a controlled diet, which have been reported to be some of the most important tools for the effective reduction of blood pressure (Host and Halken, 2004). Careful selection of enzymes and temperature for proteolysis produces the maximum biological activity.
Conclusion
Based on the findings of this study, the hydrolysates of WPC-35 exhibited improved ACE-inhibitory activity by releasing peptides that are inactive in intact WPs with the influence of enzymatic activity. It is known that β-Lg and α-La are the prominent peptides in whey proteins and are available for bioactive functions like ACE-inhibitory effects after hydrolysis. Protease S had the highest proteolytic activity compared to the other enzymes for hydrolysis of WPCs. Protease S treated WPHs showed the highest ACE-inhibitory effect out of the other experimented enzymes, and second was alcalase. Protease S released ACE-inhibitory active peptide LDAQSAPLR and trypsin released LAMA, and LDAQSAPLR after 3 h hydrolysis. Among the experimented enzymes protease S had better performances in hydrolyzing WPC-35. WPCs had many other inactive bioactive peptides of antibacterial and antioxidant activity that are available to enhance by releasing with enzymatic hydrolysis. Based on the specific bioactive peptides of the hydrolysates, various applications and alterations could be carried out with proper selection of the enzymes.