Introduction
Until now, tenderness has been recognized as the most important quality characteristic of meat, and meat tenderness is affected by various factors includes protein, muscle fiber, post mortem condition, fat content, water holding capacity as well as the applied process (Lawrie, 1998). Various physical and biological techniques have been developed, particularly aging or enzymatic tenderization techniques, which have been representatively applied for production of tender meat. Aging is a natural tenderizing technique which provides not only tenderness, but also preferable flavor characteristics of meat. However, low yield of edible portion and long process period limited the aging technique in industrial application (Dashdorj et al., 2016). Enzymatic tenderization which used proteases is an alternate technique, nevertheless this technique involved uneven tenderization and difficulty in controlling enzymatic behavior (Gerelt et al., 2000).
Sous-vide (SV) cooking is lexically defined by cooking under a vacuum, while this process is recognized as cooking at a defined temperature for a long time (Sanchez del Pulgar et al., 2012; Naveena et al., 2016). Previously, the impacts of SV on meat quality have been conducted in a broad range of temperature (50°C–100°C) for various periods of time (3–24 h), and the results demonstrated that the SV process was advantageous in enhancing flavor (Schellekens, 1996), tenderizing (Laakkonen, 1973), better yielding (Vaudagna et al., 2008), delaying lipid oxidation (Naveena et al., 2016) and suppressing microbial growth (Roldan et al., 2013). Therefore, it is obvious that the SV process has a potential application to produce tender meat with extended shelf-life. Still, it was hard to predict the optimum SV condition of meat because the reported optimum condition was varying depending on meat sources, type of muscle, applied temperature, and time.
Pork loin consists mainly of moisture and protein while fat content is relatively low among porcine muscles. Cooking manifested less juicy and less tender pork loin, which resulted in a lower eating preference. It is expected that the SV process improves the eating quality of pork loin, nevertheless, there is no information regarding the best SV condition of pork loin. Jeong et al. (2018) reported that pork ham processed by SV at 61°C for 45–90 min exhibited lower cooking loss and shear force than control, however the advantages of the SV condition was not found in pork loin based on our preliminary study (unpublished data). To identify the optimum SV condition for pork loin, therefore, this study investigated the effect of SV temperature (50°C, 55°C, and 60°C) and time (12 and 24 h) on the quality characteristics of pork loin.
Materials and Methods
A total of three pork loins (M. longissimus dorsi, crossbreed of Landrace×Yorkshire×Duroc, 6 mon old hogs) were purchased at 24 h post-mortem from a local market. Excessive fat and connective tissue were trimmed off and 8 slices with 10 mm thickness from each muscle were sampled. The sliced meat was weighed, and vacuum packaged in UV-sterilized vacuum pouches (laminated with nylon and low-density polyethylene) using a vacuum packer (HZ-206T, Hanbyul digital, Korea) with the maximum vacuum pressure. All packaged slices were placed at 4°C prior to thermal processing (within 3 h). For replicated experiments, the sample preparation was repeated three times on different days (n=3).
The samples were divided by 8 groups and each group consisted of three slices. One group was used as a fresh control (FC). Another group was heated in a 75°C water bath for 30 min and used as a cooked control (CC). The remaining 6 groups were applied to SV treatment. In this study, SV was conducted at three temperatures (50°C, 55°C, and 60°C) for two times (12 and 24 h). When all thermal treatments were completed, the cooked sample was cooled down in 4°C water for 30 min and kept at 4°C prior to analysis (within 2 h).
The meat packages were opened, and surface exudate was gently removed using a tissue. The meat was weighed again, and cooking loss was calculated based on percentage weight loss over initial weight. As an indicator of juiciness, the expressible moisture (EM) was conducted by the method of Montero Garcia et al. (1996) with minor modifications. Approximately, 1 g of meat (WM) was taken from three random places of each sample. The meat was placed on gauze (moisture absorber) in a test tube and centrifuged at 1,500×g for 20 min using a 4°C centrifuge (1248R, Labogene Co., Korea). After centrifuging, a meat pellet was carefully removed, and the tube was weighed (W1). The tube was dried at 105°C for 24 h and weighed again (W2). The EM of meat was calculated using the following equation.
Each 5 g piece of meat was taken from three slices and the meat was homogenized with 45 mL distilled water. The pH of meat was measured using a pH meter (FE20, Mettler Toledo, USA).
The differential scanning calorimetry (DSC) profile of treatments was measured using a differential scanning calorimeter (200F3, Netzsch GmbH, Germany) calibrated by indium. Approximately 20 mg samples taken from two random places were placed on a metal fan and sealed tightly. The fan was linearly heated from 20°C to 90°C at a rate of 0.5°C/min. An empty fan was used as a reference.
Shear force as an indicator of meat tenderness was determined using a texture analyzer (CT3, Brookfield Engineering Labs Inc., USA) equipped with a probe (TA 25/1000, Brookfield Engineering Labs Inc., USA). Six meat strips (1×1×5 cm) were sampled from each sample and sheared by a table speed of 1 mm/s. The force required to cut the strip was recorded and expressed as shear force.
A total of 5 g of the sample was taken under aseptic conditions to estimate the aerobic count of treatment. The meat was added into 45 mL of sterilized water and homogenized using a stomacher (BaqMixer 400W, Interscience, France) for 90 s. The homogenate was diluted until ×106, and 1 mL was spread on petri-film (Aerobic, 3M Co. Ltd., USA). The film was incubated at 37°C for 48 h, and the total plate count (TPC) was expressed as a log colony forming unit.
A color reader (CR-10 Plus, Konica Minolta Sensing Inc., Japan) calibrated by white standard board (L*=100.5, a*=−2.4, b*=5.6) was used to measure the surface color of meat samples. Color was taken from 6 random positions of each meat sample.
A completely randomized design was adopted to estimate the effect of SV treatment on the quality of pork loin. All data collected from each experiment were averaged and three averages from three entirely repeated experiments were expressed as mean±SD (n=3). One-way analysis of variance (ANOVA) was conducted using R statistical software (version 3.5.1, R statistics, New Zealand). When the main effect was significant (p<0.05), Tukey’s honestly significance test was performed as a post hoc test.
Results and Discussion
All treatments had significantly lower cooking loss than that of control (p<0.05) (Table 1). In comparison of the treatments, temperature and time had an influence on the cooking loss of pork loin in different ways. In general, cooking loss of pork loin tended to be proportional to the temperature, and meat processed at 50°C showed the lowest cooking loss among treatments, which was in general agreement with the previous report (Tornberg, 2005). Treatment at 50°C showed lower cooking loss with increasing cooking time (p<0.05). At a temperature higher than 55°C, process time did not affect the cooking loss of pork loin, however cooking loss of treatment at 60°C for 24 h tended to be higher than that processed for 12 h. Cooking loss of meat is related with the shrinkage of muscle fiber and connective tissue, and the direction of thermal shrinkage to the muscle fiber axis is dominantly changed at around 60°C from transverse to longitudinal (Purslow et al., 2016). Based on the review of Tornberg (2005), transverse shrinkage attributed to expand the gap space between muscle fibers and endomysium, thereby reducing the amount of drip generation. Alternately, denaturation of connective tissue protein which is responsible to the longitudinal shrinkage of muscle accounted for most of the cooking loss of meat, mainly due to shortening of muscle fibers (Tornberg, 2005). It was reported that onset denaturation temperature of porcine connective tissue was 57.2°C–61.4°C in DSC analysis (Voutila et al., 2007) which would explain the increase in cooking loss of pork loin processed at 55°C.
The estimated EM of cooked pork loin was in accordance with the result of cooking loss. The FC showed low EM compared to cooked treatments due to the high water binding property of native meat proteins. On the other hands, thermal processed meat proteins undergo denaturation, hence the EM of cooked samples is closely dependent on the amount of residual moisture which contributes to eating quality such as juiciness (Schonfeldt et al., 1993). Among treatments, the EM tended to decrease with increasing cooking temperature. Regardless of time, SV at 50°C showed higher EM than that of CC (p<0.05). Based on the results, SV process of pork loin was more beneficial to retain moisture than traditional cooking methods (high temperature short time cooking), and optimum temperature was 50°C which was lower than those estimated using other cuts rather than pork loin (Jeong et al., 2018).
Compared to pH 5.68 of FC, all cooked treatments had high pH (p<0.05), and the pH of treatments were affected by cooking conditions (Table 1). In comparison of cooked treatments, all SV treatments, excluding those processed at 50°C for 12 h, showed higher pH than that of CC (p<0.05). It was generally found that cooking manifested an increase in pH of meat which was resulted from the structural changes of meat proteins (Fletcher et al., 2000). It was reported that the increase in pH of cooked meat was due to a loss of acidic amino group (Lawrie, 1998), an exposure of basic amino residues (Forrest et al., 1975) and/or a formation of free hydrogen sulfide (Vasanthi et al., 2007). Despite relatively low cooking temperature of SV, long processing time would manifest higher pH of SV treatments than that of CC.
Based on DSC thermogram, typically three endothermic peaks from FC were obtained at 55°C, 64.8°C, and 78.7°C (Fig. 1). The peak temperature was identical to previous porcine research (Deng et al., 2002). The first peak which is associated with myosin disappeared in all cooked samples, reflecting that myosin was completely unfolded at the cooking condition adopted in this study. The onset temperature of myosin was 50°C and extended cooking (>12 h) at 50°C caused denaturation of myosin thereby causing the disappearance of the first peak of all cooked treatments. Alternately, the second peak was still observed in SV treatments processed at 50°C–55°C, however, the peak tended to shift towards a higher temperature with increasing temperature. The result was not fully understood in this study, while Kim et al. (2005) reported that the second peak temperature increased when sarcoplasmic protein was added into pollock surimi. Based on the report of Xiong et al. (1987), the second peak of meat was related with four components including sarcoplasmic proteins, collagen, myosin (tails), and actomyosin. It could be assumed that an interaction between sarcoplasmic protein and other components would manifest the movement of the second peak temperature. The third peak which indicates actin denaturation was also found in cooked meat at 50°C–55°C. Alternately, the actin peak was completely disappeared at 60°C which would be due to relative long processing time of SV treatment.
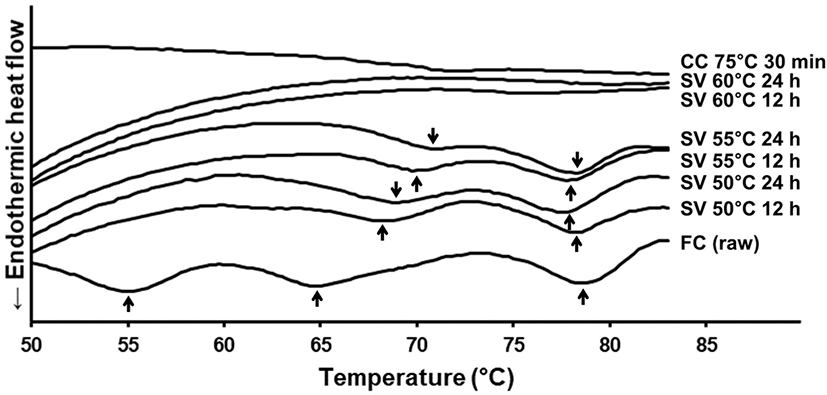
It was obvious that the SV had an advantage to produce tender meat compared to traditional cooking (Table 1). Regardless of process condition, all SV treatments showed lower shear force than that of CC (p<0.05). Lower cooking loss of SV treatments would account for better tenderness than CC which was in accordance with previous reports (Purslow et al., 2016; Tornberg, 2005). However, the shear force of SV treatment did not show any pattern to applied temperature or time. Excluding SV at 50°C for 24 h, the main contributor affecting the shear force of pork loin processed by SV was temperature (p<0.05), and impact of time was not shown. Conversely, process time at 50°C was an important factor to provide tenderness of pork loin (p<0.05). Tenderness of SV processed meat was affected by various factors of which a structural change of meat proteins would have a key role to regulate the tenderness. Still the sarcoplasmic proteins were not denatured completely at 50°C and intrinsic proteases such as cathepsins and collagenases probably retained their proteolysis activity at this temperature (Ertbjerg et al., 2012; Hamdy, 2008). Eventually, the time-dependent tenderization effect of pork loin processed at 50°C would be manifested by the action of enzymes (Tornberg, 2005). Meanwhile, thermal inactivation of the enzymes occurred at above 55°C, hence the cooking time did not affect the shear force of pork processed at higher than 55°C. However, temperature-dependence of shear force was probably due to thermal disintegration of triple-helical structure of collagen (Baldwin, 2012). Consequently, the results demonstrated that SV was an effective cooking method to obtain tender meat compared to traditional cooking methods.
In this study, SV was processed at a moderate temperature (50°C, 55°C, and 60°C) at which there was a risk of foodborne pathogenic growth, hence the important factor to be considered in SV was not only tenderization, but also impact of sterilization. Despite the fresh control that showed 3.81 Log CFU/g of TPC, the TPC was not detected in all cooked treatments (Table 1). Based on the review of Baldwin (2012), 4.4°C–60°C is the danger zone of meat, while meat safety can be obtained by cooking at 52.3°C for 12 min. Extended cooking time (12–24 h) would diminish the potential microbial growth and provide an acceptable sterilization for SV processed meat. By combining low cooking loss and tenderness, SV was favorable to apply for meat rather than traditional cooking methods from the eating quality, safety and organoleptic points of view (Roldan et al., 2013; Schellekens, 1996). For color, all cooked treatments exhibited typical cooked meat color which was characterized by high L* with low a* and b*. Among cooked treatments, SV did not show a difference in color with those of CC. Consequently, the results demonstrated that the SV process at a moderate temperature did not provide a drawback in microorganisms and color compared to traditional cooking methods.
Conclusion
This study demonstrated that the SV process has a potential application to produce tender meat with high moistness. Degree of protein denaturation, structural changes in myofibrillar systems and connective tissue as well as enzyme activity would involve in the tenderness and moistness of SV processed meat. Despite the SV process being conducted at a moderate temperature (50°C, 55°C, and 60°C), long processing time (12 and 24 h) diminished the risk of microbial growth and residual uncooked color. Consequently, the SV was a better cooking method for low fat lean meat such as pork loin.