Introduction
Most consumers in Iran and other countries are inclined to have fresh, natural color, properly-chilled, and delicate meat of a typical texture without muscle drop, leakage and microbial contamination. In contrast, industrial-commercial consumers, producers, and vendors are more inclined to consume frozen meat owing to its long storage capability and quality preservation (Atanassova et al., 2018). Currently, various methods of meat storage have been developed throughout the world. However, freezing is the most efficient and widely used method for maintaining the nutritional value of meat even after long storage (Rahman et al., 2014). Freezing plays an important part in preserving the quality and safety of meat (Leygonie et al., 2012). However, the consequences of freezing and thawing are still a major problem affecting the quality of meat (Leygonie et al., 2012).
During the freezing process, ice crystals are formed in the extracellular space, causing mechanical damage and volume changes in cellular structures (Dalvi-Isfahan et al., 2016; Wang et al., 2018). The crystal formation causes punctures in the cell membrane and the leakage of proteins, vitamins, and minerals from the intracellular into the extracellular space; this contributes to microbial growth (Leygonie et al., 2012), thereby reducing the quality of fresh meat (Hui et al., 2004); therefore, the price of frozen meat is lower compared with fresh meat. The lower marketability of frozen meat has led to some adulteration and illegal sale of frozen-thawed meat as fresh one (Ballin and Lametsch, 2008). Appropriate analytical methods are required to enforce the regulations and obtain comprehensive information on the measures of adulteration in the supply of frozen-thawed meat instead of fresh meat. For the detection of adulteration, various studies have been conducted on the use of different methods such as enzymatic methods, particularly hydroxyacyl-coenzyme A dehydrogenase (HADH), DNA-based methods, IR and NMR techniques, biographical imaging methods and electron microscopy, impedance measurement, and sensory methods (Ballin and Lametsch, 2008; Chen et al., 2016; Elahi et al., 2016; Grunert et al., 2016; Sánchez-Alonso et al., 2014). Nevertheless, most of these methods are not thorough enough to differentiate fresh from frozen-thawed meat because each has its own pros and cons in diagnosis. Tan et al. (2012; 2013) introduced a spectroscopy method based on the Maillard reaction to identify the minced veal of chicken and pork and their freshness index (Ames, 1998; Tan et al., 2012; Tan et al., 2013). A large number of researchers have indicated the use of Maillard reaction by studying the taste, color and aroma of food, its effect on the sensory characteristics, and the toxicity of the resulting material (Chen et al., 2018; Jiang et al., 2018; Karangwa et al., 2016; Meinert et al., 2009; Tamanna and Mahmood, 2015).
Maillard's reaction is a non-enzymatic browning between the amine group of free amino acids, peptides, or proteins and the carbonyl reactive groups of reducing sugars or carbonyl components such as aldehydes and ketones derived from the oxidation of lipids, occurring during the thermal or food storage processing (Ames, 1998; Karangwa et al., 2016). At the end of this reaction, brown nitrogenous polymers and copolymers, known as melanoidin, are formed, inducing a change in the colors from brown to black. The pH and browning intensity are two distinctive features of Maillard reaction that change following 95°C heating. Protein is the main substrate for the reaction of Maillard. The changes in protein during the freeze-thaw cycle lead to protein oxidation and reduce its functional properties. Storage of meat under freezing conditions significantly reduces the amount of denaturation enthalpy, the number of sulfhydryl groups, and the protein solubility, and increases the carbonyl and disulfide content of the protein. The free NH2 group may react with the aldehyde compounds resulting from lipid oxidation (Tan et al., 2012; Tan et al., 2013). Protein changes in the freezing process also include changes in the amino acid side chains and polymer formation, reduced ATPase activity, altered amino acid composition, increased sensitivity to proteolytic enzymes, and reduced protein water holding capacity (WHC) (Hui et al., 2004; Tan et al., 2012; Tan et al., 2013). Besides, the total amount of essential and nonessential amino acids and the amount of each protein amino acid decreases throughout the freezing storage (Baranenko et al., 2014). These changes are dependent on the structural specification of amino acids, the chemical structure of their radicals, their solubility in the water, and the freezing temperature of the meat (Baranenko et al., 2014). As a result, denaturation, oxidation, and structural changes of protein reduce the reaction of the protein and ribose, thereby decreasing the production of melanoidin and changing the Maillard reaction parameters such as color, pH, and absorption rate. Therefore, the Maillard reaction relies on measuring the absorbance of melanoidin (A420*) obtained from the extracted minced veal and ribose could provide an alternative method.
To date, the application of the Maillard reaction has not been studied as a means of differentiating between fresh red meat and frozen-thawed meat. This method was previously evaluated in poultry and pork meat by some researchers (Ivanova et al., 2014; Tan et al., 2013). The purpose of this paper was to evaluate the capability of applying the Maillard reaction as an alternative method for differentiation and identification of from frozen-thawed minced veal. The Maillard parameters such as pH changes, colorimetric parameters (CIE a*, CIE b*, and CIE L*), and absorbance at 420 nm were measured at different heating times to find the most optimal separation condition and cut-off value.
Materials and Methods
Sucrose and D-ribose for Maillard reaction and other chemical materials (analytical grade) used in this research were purchased from Sigma Aldrich (St. Louis, MO, USA). The selected materials under research included 500 g meat isolated from the Biceps femoris muscles of three calves aged no more than six months purchased from the butcher’s. The meat was placed in a refrigerator at 4°C for 24 h after slaughter.
At first, all visible fats were removed from the meat surface. The samples collected from each calf (3 batches) were separately divided into three equal parts. Samples were cooled in a refrigerator at 4°C two hours before being used in a grinding machine (PA-82; Mainca, Barcelona, Spain) equipped with a 4.5 mm plate. A total of 180 packed veal samples were stored at different temperatures, including 20 packed samples in a chilled condition at 4°C, 20 packed samples in a super chilled condition at −4°C, and 20 packed samples in a freezing condition at −18°C from each batch. Four replicates of the samples were taken for five different heating times. The chilled samples were tested on the first day, while others were tested after one month. Prior to analysis, each sample was thawed for 4 h at 5°C under natural convection.
The chemical compositions of the samples were determined following the standard methods prior to the experiment. Ash, moisture, and protein content in meat samples were determined based on the standard method (AOAC, 2010). Lipid was extracted for total lipid content, determined following the procedure of Bligh et al. (1959). Results were reported based on moisture percentage (Bligh and Dyer, 1959).
Induction of the Maillard reaction in the minced veal samples and the extraction of water-soluble melanoidin from samples extracted by heating ribose with minced veal were performed according to the procedure described by Tan et al. (2012) with certain modifications. In this experiment, ribose was employed to induce the Maillard reaction and sucrose was applied as a blank. Minced veal was manually mixed (10% w/w) with D-ribose in a 30 mL universal glass bottle individually. The bottle which contained 7 g ribose-minced veal mixture (ca 6.4 g minced veal and 0.64 g D-ribose) was heated at 95±1°C for 20, 40, 60, 80, and 100 min in the water bath (water / fluid bath GFL 1008, Berlin, Germany). The heated samples were instantly made cold in ice water, left at room temperature for up to 1 hour before analysis, and ground to obtain homogenous powder by use of a mortar and pestle; 20 mL of distilled water was then added. In the following, the extraction procedure was done according to Tan et al. (2013). Controlling was done with sucrose, a non-reducing sugar, instead of ribose. Absorbance was judged at 420 and 550 nm employing a UV-Vis spectrophotometer (Lambda 25, Perkin Elmer, CA, USA) for the absorbance of brown polymers generated in the Maillard reaction as the browning index (A420*). The corrected absorbance value was calculated as proposed by Tan et al. (2013). Any turbidity in the extract was corrected by absorption at 550 nm according to equation (1):
where A420* is the absorption of browning at 420 nm, A550 is the absorption of 550 nm for samples and B is the absorption at 420 nm for a blank sample (Morales and Van Boekel, 1998).
The pH of blank and meat samples was assessed through applying a digitalized pH meter (MP230, Mettler Toledo, Greifensee, Switzerland), (AOAC, 2010). The calibration of pH meter was performed by use of two standard buffers of pH 4.0 and 7.0 at 23°C.
The color of the supernatant was measured using a Hunter Lab colorimeter (Color Flex EZ Spectrophotometer, Reston, USA). The color was evaluated via CIE L*, CIE a*, and CIE b* system. CIE L*, CIE a*, and CIE b* values were determined as indicators of lightness, redness, greenness, yellowness and blueness, respectively (+a*=redness, –a*=green, +b*=yellow, –b*=blue).
Statistical analysis was performed on the average of four replicates of the samples. In data analysis, the normality of the data was primarily investigated using a one-sample Kolmogorov-Smirnov test with Lilliefors correction and Shaprovix. For normal data, analysis of variance and Tukey and Kruskal-Wallis tests were employed, and for abnormal data, the Dan-Bonferroni tests were applied. In the same analysis, the results of mixed models were further used. To cluster the results, hierarchical algorithm and the Complete Linkage (or Furthest Neighbor) was utilized. The software used in this study was IBM-SPSS software (SPSS, Windows version 20, SPSS Inc., Chicago, IL, USA) and SAS version 9.4 (SAS Institute Inc., Cary, NC, USA), (Proc glimmix procedure). The significance level was considered to be less than 0.05.
Results and Discussion
Table 1 shows the mean analysis of the physicochemical compositions of the three batches of fresh meat samples. There was no significant difference (p>0.05) regarding the physicochemical characteristics of the meat samples.
Table 2 shows the results pertaining to the evaluation of Maillard parameters depending on different storage temperatures. The Maillard's browning reaction was commenced by adding ribose to meat samples and after heating at 95°C. In the present study, ribose-induced method was used owing to its high Maillard reaction in terms of pH reduction and browning intensity (Ashoor and Zent, 1984; Beck et al., 1990; Tan et al., 2012). However, in the blank sample, the color did not change due to the presence of sucrose instead of ribose, which is consistent with the results of other researchers (Tan et al., 2012; Tan et al., 2013). Browning in all the minced veal samples was shown by increasing the heating time. The process of these changes at different heating times is shown in Fig. 1, where a gradual reduction occurred in CIE L* and pH (Fig. 1c and 1d), and an increase was observed in CIE b* up to 60 min and CIE a* up to 80 min, after that time (Fig. 1a and 1b).
Data are represented as mean±SD of four measurements per sample. Values in the same column for the same variable with the same letter are not significantly different (p>0.05).
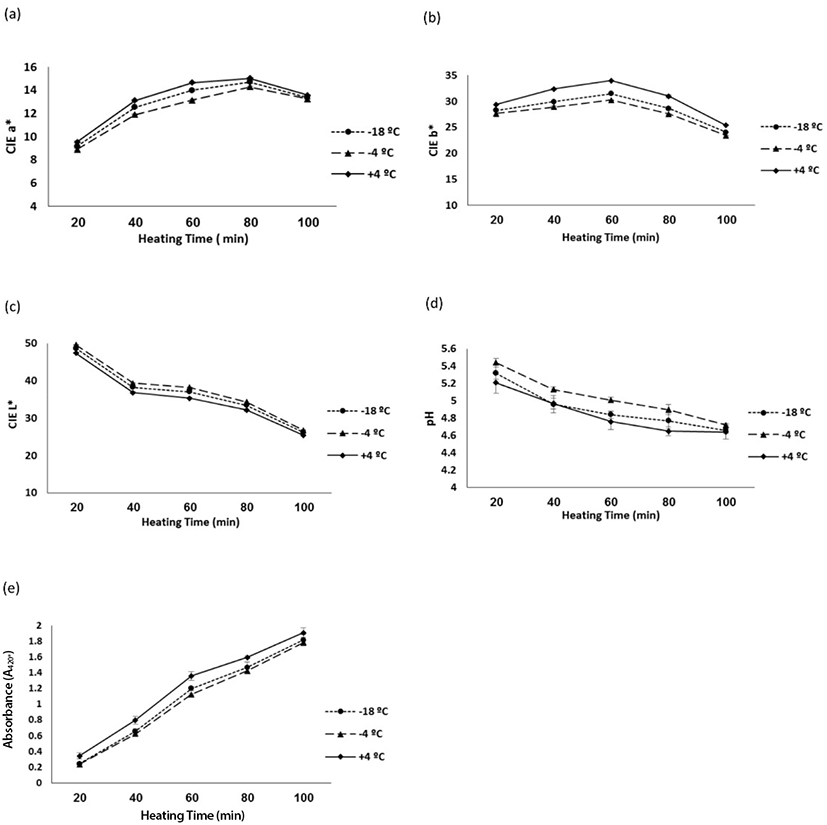
The extracts obtained from the Maillard reaction became darker and more yellowish. As reported by another researcher, the formation of browning polymers (melanoidins) reduces the CIE L* and increases the CIE b* (Morales and Van Boekel, 1998; Tan et al., 2012). Increase in CIE b*, CIE a*, and A420* value may be explained by the formation of yellow pigments in non-enzymatic browning reactions in the meat between amine and lipid oxidation products, increasing at longer heating times (Wang et al., 2018; Xia et al., 2009).
Changes in pH value and color parameters were used as an indicator for the development of the Maillard reaction as indicated by other researchers (Ivanova et al., 2014). Moreover, the present results are in line with those of Tan et al. (2012). The obtained results in this study showed that the extracts of chilled samples heated with ribose had less pH and CIE L* compared with the frozen ones (Table 2). However, CIE b*, CIE a*, and A420* values in the extract of fresh-chilled veal were significantly (p<0.05) higher than frozen-thawed samples (Table 2). Furthermore, the extract yielded from fresh-chilled veal became darker and more yellowish compared with frozen samples, and the extract yielded from frozen meat samples at −18°C became darker and more yellowish compared with super chilled at −4°C (Table 2). This can be attributed to the biochemical changes in the meat proteins during the freezing temperatures and storage conditions which can reduce the access to the basic amino acids participating in the Maillard reaction (Tan et al., 2013). These results are consistent with a similar research conducted by Dalle Zotte et al. (2016) on rabbit meat. They asserted that pH, CIE b*, and CIE a* of the frozen rabbit meat were lower than the non-frozen one (Dalle Zotte et al., 2016).
The pH value was lower in the extracts obtained from fresh-chilled samples in comparison with the frozen ones; the pH of frozen samples was significantly (p<0.05) lower than those stored at higher temperatures (−4°C). Chilled, super-chilled, and frozen veal samples were reduced by 11%, 13%, and 12.5%, respectively (Table 2). This can be ascribed to factors such as loss of basic amine groups and formation of less basic compounds by amine and condensation between the free amino and carbonyl groups of the substrates, yielding organic acids (Beck et al., 1990; Tan et al., 2012; Tan et al., 2013).
In this study, the browning intensity of the extract was evaluated by measuring the absorbance at 420 nm with a spectrophotometer as well as the direct analysis of the color. The A420* value increased significantly (p<0.0001) with the increase in the heating time up to 100 min. The A420* value achieved from the extracted fresh-chilled meat was the highest; moreover, the A420* value achieved from the extracted frozen meat sample was higher than the extract of the super-chilled sample, possibly due to the undesirable effects of storage on meat proteins (Fig. 1e). Baranenko et al. (2014) reported that the number of amino acids was significantly reduced in frozen meat in comparison to the fresh meat and at higher temperatures of freezing compared with lower temperatures (−24°C compared to −35°C). This process was expressed by the reaction of the carboxylates and the formation of nitrogen compounds (Baranenko et al., 2014).
Factorial experiment was performed to study the effects of two factors, namely the type of minced veal (X1) (fresh-chilled at 4°C, super chilled at −4°C and frozen meat at −18°C) and the duration of heating (X2) (20, 80, 60, 40, and 100 min), on the output of the Maillard reaction via ribose induction (pH, CIE L*, CIE a*, CIE b* and A420*). The results showed that the impact of X1 and X2 was significant on all variables (p<0.0001) (Table 3). The interaction between X1 and X2 significantly influenced the Maillard reaction parameters, indicating that they did not function independently; therefore, the changes in CIE and A420* values were pertinent to their interactions. The results of this study further showed that with the increase up to 60 min in the heating time, the distance between each parameter increased among the three types of meat stored at different temperatures (Fig. 1). This can be due to the progression of the Maillard reaction and accumulation of the reaction products. However, by raising the heating time up to 100 min, these parameters became closer and the differentiation distance among the three types of meat was reduced (Fig. 1), due to the increased moisture and pH reduction, limiting the Maillard reaction (Easa et al., 1996; Tan et al., 2012). The rate of the reaction is low at the highest or lowest water activity (aw) values (Davidek et al., 1990; Macrae et al., 1993). The effect of pH is highly dependent on the moisture content (Harrison and Dake, 2005). In this study, by ribose induction in veal following the heating, Maillard reaction was developed, yielding increased amounts moisture and reduced pH. The results showed that the A420* value and the browning factor in both fresh and frozen-thawed meat samples were increased by raising the heating time; however, according to the different dendrograms drawn corresponding to different heating times, the highest subtraction was found to be due to the lowest similarity and maximum confidence interval between fresh and frozen-thawed meat at 60 min compared to 80 and 100 min. Moreover, in all parameters, the maximum confidence interval between meat samples stored at three different temperatures was obtained following 60 min heating at 95°C (Fig. 1), which is in accordance with the findings of Tan et al. (2012).
Complete linkage ensures that all items in a cluster are within a maximum distance (or minimum similarity) from one another (Johnson and Wichern, 2007). Therefore, in this research, complete linkage clustering was used to group the fresh and frozen-thawed meat samples (4°C, −4°C, and −18°C) with different Maillard reaction parameters over the heating time. After grouping all 36 samples into three statistically significant clusters, all the dendrograms showed similar patterns with different degrees of similarities. A rendered dendrogram relying on 60 min of heating classified the meat samples into statistically significant clusters: cluster 1 (samples 1–12 of super-chilled minced veal), cluster 2 (samples 13–24 of frozen minced veal), and cluster 3 (samples 25–36 of fresh-chilled minced veal), with the lowest similarity (slightly more than 25%) between clusters 12 (cluster 1 and 2 totally) and clusters 3 (Fig. 2). Similarities between these clusters increased at 20 and 100 min of heating time. Therefore, Maillard reaction can further be employed to differentiate between chilled minced veal and frozen-thawed meat. The analysis of the confidence interval has been done for separation purposes by other researchers (Kumar et al., 2008; Tan et al., 2012; Virkler and Lednev, 2009). The results showed no overlap between the two types of meat and the two freezing storage temperatures (−4°C and −18°C). Moreover, fresh-chilled and frozen-thawed meat were significantly different regarding Maillard reaction parameters (p<0.05) (Fig. 2).
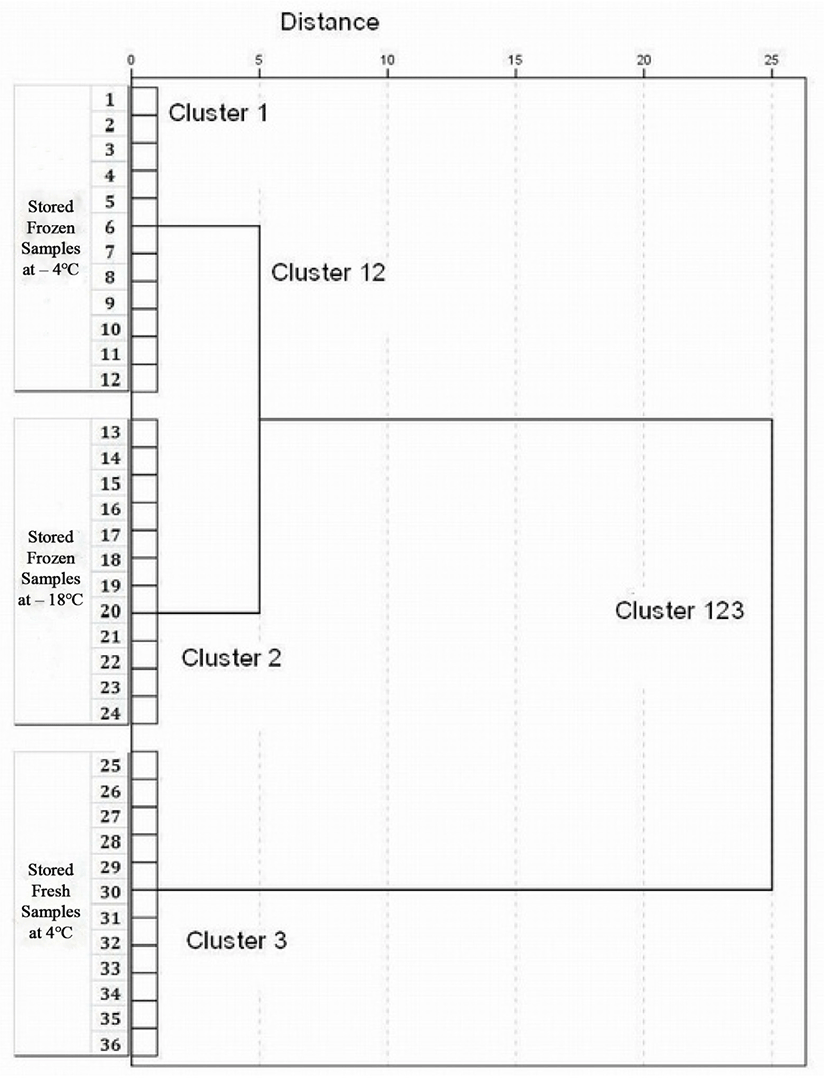
Fig. 3 shows the A420* value obtained from fresh-chilled, super-chilled, and frozen samples respectively stored at 4°C, −4°C, and, −18°C, as a function of heating time of 60 min at 95°C with ribose. The results obtained from the receiver operating characteristic (ROC) curve and area under the curve corroborated the suitability of the cut-off value (threshold limit) of 1.25 for the evaluation and differentiation of a minced veal. The A420* cut-off value was used to determine whether the veal samples were previously frozen or not. Therefore, the obtained A420* value should be compared with the cut-off value. The results of this study showed that the procedure is suitable for distinguishing between chilled and frozen-thawed veal using the proposed cut-off value of A420*. The result of the variance analysis was used to compare the mean changes of A420* value between different batches of different calves. The results showed that the A420* value in the frozen-thawed veal stored at −4°C and −18°C was reduced by approximately 17.22±3.53% and 11.68±2.49% compared with fresh-chilled veal. This result further indicated that there was no statistically significant difference (p>0.05) between the mean changes of three minced veal stored at −4°C and −18°C compared to 4°C obtained from three different calves (3 batches). According to the results obtained in this study, the possible use of the Maillard reaction for differentiation between fresh and frozen thawed minced veal was suggested instead of the current analytical techniques.
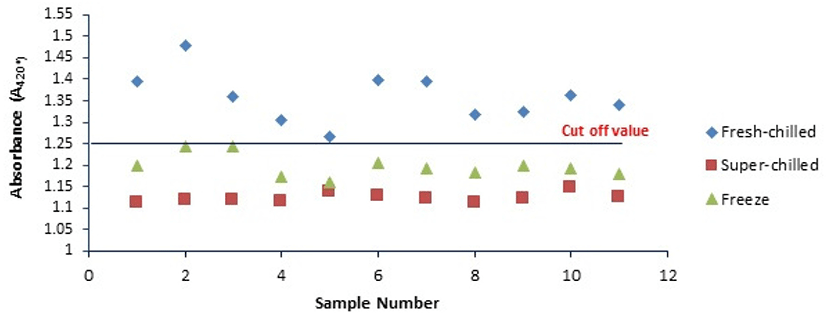
Conclusion
Storage under freezing conditions affects the protein quality of minced veal, thereby impacting its functional characteristics. A420* value was related to the storage duration in both fresh and frozen-thawed meat. Due to denaturation and oxidization proteins, the obtained A420* value of fresh-chilled minced veal was higher than the previously frozen one. On the other hand, A420* value was lower in samples stored at a higher temperature (−4°C), implying the possible applications of the Maillard reaction to reveal the inadequate temperature during frozen storage. The results showed the capability of diagnostic criteria and differentiated between fresh and frozen-thawed minced veal. Nonetheless, more research is required to investigate the impact of the storage temperature, freezing time, repeated freeze-thaw cycles, freezing rate, and type of muscle tissue on ribose-induced Maillard reaction, to try out the proposed method and make it a diagnostic tool for differentiating between fresh and frozen-thawed meat.