Introduction
Meat flavor plays a major role in determining consumers’ liking and acceptance of the meat (Khan et al., 2015). Meat flavor is the complex combination of unique tastes and aromas perceived in the mouth, tongue, and nasal cavity, arising from the interaction of various volatile organic compounds (VOCs) and taste components produced through thermal and non-thermal processes (Dashdorj et al., 2015; Kerth and Miller, 2015; Xu et al., 2023). Raw meat is generally described as bloody-like aroma with metallic and salty tastes; however, during the thermal or non-thermal processes such as cooking, aging, curing, etc., a strong meaty flavor develops along with roasted and fatty aroma and umami taste, depending on the type of chemical reaction (Kosowska et al., 2017; Xu and Yin, 2024). In particular, the formation of meat flavor is largely influenced by cooking methods, such as grilling, boiling, frying, roasting, etc. (Lee et al., 2021; Suleman et al., 2020). Among them, high-temperature heating can contribute to the meat flavor owing to the Maillard reaction which occurs at high temperatures (Yoo et al., 2020). Maillard reaction is a non-enzymatic reaction between the amino group from amino acids, peptides, or proteins and the carbonyl group from reducing sugars to produce Maillard reaction products (MRPs) which are responsible for meat color and flavor (Chiang et al., 2019; Liu et al., 2023; Ma et al., 2020).
A Maillard reaction model system is effective in understanding the mechanism of Maillard reaction and investigating the characteristics of MRPs in a simple way (Xu et al., 2013; Zou et al., 2019). This model system includes a mixture of amino acids or peptides which provide amino groups and reducing sugars which contain carbonyl groups (Xiao et al., 2015). The precursors determine the sensory characteristics of MRPs. A simple model system consisting of a pure amino acid and a reducing sugar would yield a limited composition of VOCs (Xia et al., 2021). Adding protein hydrolysates or lipids in a model, on the other hand, can enrich the flavor of MRPs. In contrast to plant or yeast proteins which can partially simulate the natural meat flavor, animal proteins and fats act as excellent precursors for producing rich and pure meaty flavor with diverse kinds of VOCs owing to their abundant contents of amino acids (Chai et al., 2018; Chiang et al., 2022; Xiao et al., 2015; Ye et al., 2022).
The purposes of using animal resources as the precursor of a model system are: (1) to upcycle the animal by-products as value-added products for food sustainability; (2) to enhance the sensory attributes or bioactivity of animal protein hydrolysates; (3) to improve the meaty flavor of the MRPs, e.g. adding animal fat into the plant protein-based model systems; (4) to understand the mechanism of animal peptides on the participation of Maillard reaction and the formation pathways of the representative VOCs (Chiang et al., 2019; Nie et al., 2017; Ye et al., 2022; Zou et al., 2019). Nonetheless, the information about the generation of meaty flavor from animal-derived flavor precursors via the Maillard reaction model system is still limited (Liu et al., 2015).
This review comprises the comprehensive literature on the sensory characteristics of MRPs that were prepared via the model system with animal resources and summarizes the factors that influence the degree of reaction, composition of VOCs, and sensory attributes. Information about the flavor precursors in meat products and their contribution to meat flavor is also provided for a better understanding of the experimental design of Maillard reaction model systems.
Meat Flavor Formation through the Maillard Reaction
The flavor of raw meat can be described as salty, metallic, and bloody taste with little aroma intensity (Kosowska et al., 2017; Van Ba et al., 2012). However, when the flavor precursors present in meat participate in the Maillard reaction, lipid oxidation, and the interactions between the products from the Maillard reaction and lipid oxidation via thermal processes, the cooked meat flavor with roasted and savory aromas and umami taste develops (Resconi et al., 2013). Flavor precursors in meat are constituted of non-volatile water-soluble components such as amino acids, peptides, reducing sugars, and thiamine, and lipid components (Dashdorj et al., 2015; Song et al., 2012). Water-soluble components contribute to the generation of typical meat flavor, while lipids are responsible for species-specific meat flavor (Sun et al., 2014). The amount of each flavor precursor is a critical factor in the development of the meat flavor, as the reaction during the Maillard reaction and lipid oxidation occur differently depending on the relative contents of flavor precursors.
Amino acids and peptides in the meat are largely responsible for the meat flavor. In terms of the Maillard reaction, the participation of individual amino acids of alanine, aspartic acid, phenylalanine, proline, serine, and tyrosine contributes to the floral aroma, while glycine, lysine, threonine, and valine give a caramel odor, whereas the sulfur-containing amino acids including cysteine and methionine produce a strong meaty aroma, as each amino acid favors the formation of different VOCs (Aaslyng and Meinert, 2017; Wong et al., 2008). For instance, Li and Liu (2022a) added cysteine to heated enzymatic pork trimmings hydrolysate and reported an increase in the sulfur-containing VOCs such as 2-furfurylthiol which were absent in non-enzymatic samples. Further, the participation of amino acid mixtures or peptides from the thermal or enzymatic degradation of meat proteins in the Maillard reaction generates strong, complex, and long-lasting sensory characteristics of MRPs (Zhang et al., 2020; Zou et al., 2019). Therefore, meat flavor can be improved through adequate cooking methods or aging processes which produce many amino acids and peptides (Kim et al., 2019; Suleman et al., 2020). The low-molecular-weight peptides present in meat such as anserine, carnosine, and glutathione can also be used as precursors for the development of meaty flavor after thermal processes (Zhou et al., 2019). For peptides, the reaction mechanism in the Maillard reaction and the sensory properties of the MRPs are greatly determined by their amino acid composition, sequence, and molecular weight, which will be discussed later.
In the case of reducing sugars, ribose is a representative carbonyl donor in meat which exists mainly as inosine 5′-monophosphate with small proportions of ribose 5′-phosphate and free ribose (Mottram and Nobrega, 2002). Other nucleotides such as adenosine 5′-monophosphate and guanidine 5′-monophosphate also participate in the Maillard reaction (Xu et al., 2018). Additionally, glucose and glucose 6′-phosphate in meat were found as important flavor precursors for meaty flavor (Li and Liu, 2021). However, the amount of reducing sugars in the meat is not high, and therefore the addition of sugars can help increase the degree of Maillard reaction in meat. For instance, Aliani and Farmer (2005) observed that the two- to fourfold increase in the natural concentration of ribose in chicken meat significantly enhanced its roasted flavor. Similarly, Li and Liu (2021) reported that a higher concentration of xylose in pork hydrolysate promoted the formation of flavor compounds after heat treatment such as furfural and 2-pentylfuran which were responsible for enhanced roasted and sweet aromas.
Animal fat, as mentioned earlier, plays a vital role in the formation of characteristic meat flavors, such as beef, pork, and chicken flavor (Ye et al., 2022). Animal fat includes triglycerides and phospholipids, which the latter mainly contribute to the sensory characteristics of cooked meat (Aaslyng and Meinert, 2017; Kosowska et al., 2017; Mottram and Edwards, 1983). Oleic acid, palmitic acid, and stearic acid are representative fatty acids that derive from the degradation of triglycerides; linoleic acid, arachidonic acid, eicosatetraenoic acid, palmitoleic acid, and linolenic acid come from the degradation of phospholipids (Fu et al., 2022). Free fatty acids that were released by the degradation of triglycerides and phospholipids during cooking are oxidized to produce hydroperoxides, which are subsequently converted into a variety of VOCs such as aldehydes, ketones, alcohols, carboxylic acids, esters, etc. (Sun et al., 2022). Therefore, the principle in species-specific meat flavor that arises from lipids is based on the differences in fatty acid profiles and the resulting carbonyls (Arshad et al., 2018). The polyunsaturated fatty acid composition is much higher in poultry compared to ruminants, which differentiates the chicken meat flavor from beef or lamb flavor (Jayasena et al., 2013). Furthermore, the intermuscular fat content and the degree of its unsaturation are also responsible for the flavor of meat (Khan et al., 2015). The moderate oxidation of lipids is beneficial for meat flavor; however, excessive lipid oxidation may deteriorate the sensory quality due to the high aldehyde contents that elicit off-flavor (Lee et al., 2024).
In addition to the proteins, carbohydrates, and lipids, thiamine also affects the meat flavor (Sun et al., 2014). It presents a relatively high content in muscle, approximately 0.08–0.11 mg, 0.17–0.18 mg, 0.23 mg, and 0.81–0.88 mg in 100 g of beef, lamb, chicken, and pork, respectively (Ramalingam et al., 2019). Thiamine can be easily degraded during thermal processes (Sun et al., 2022), resulting in the formation of many nitrogen- or sulfur-containing VOCs, such as furans, thiols, thiophenes, and sulfides, which are the major contributor to meaty and roasted aroma (Khan et al., 2015). Wei et al. (2017) reported that marinating Chinese chicken with salt, xylose, cysteine, and thiamine significantly enhanced the production of sulfur-containing VOCs, such as benzothiazole and 4-methyl-5-thiazoleethanol, which contributed to a stronger meat-like flavor.
The mechanisms of the Maillard reaction from the early stage, starting from the interaction between amino group and carbonyl group to the final stage of the production of melanoidins and the formation of representative VOCs during the reaction are well-documented previously (Diez-Simon et al., 2019; Sohail et al., 2022; Sun et al., 2022).
Maillard reaction can be divided into three stages (Fig. 1; Wang et al., 2022). It starts from the condensation between the amino group from an amino acid or a peptide and the carbonyl group from a reducing sugar at thermal conditions (Chiang et al., 2019). Then, the primary intermediates called Amadori rearrange products are produced through Amadori rearrangement (Sun et al., 2022). These compounds participate in further reactions in the intermediate stages including enolizations and condensations to form a variety of intermediates depending on the reaction condition (Lotfy et al., 2021). For example, at low pH conditions, Amadori rearrangement products undergo 1,2-enolization, subsequently releasing the amino compounds and producing furans, furfurals, hydroxymethylfurfural, etc. (Bassam et al., 2022). On the other hand, at high pH, Amadori rearrangement products are degraded via 2,3-enolization and generate reductones and various fission products like acetol, diacetyl, and puryvaldehyde (Kutzli et al., 2021). Particularly, the abovementioned compounds after 2,3-enolization of Amadori rearrangement products are highly reactive and interact with aldehydes and aminoketones that arise from the Strecker degradation, the process of degradation of amino acids in the presence of dicarbonyl compounds (Van Ba et al., 2012). As a result, numerous nitrogen-containing heterocyclic compounds responsible for savory flavor are formed. The final stage includes the formation of the brown macromolecular pigment melanoidin.
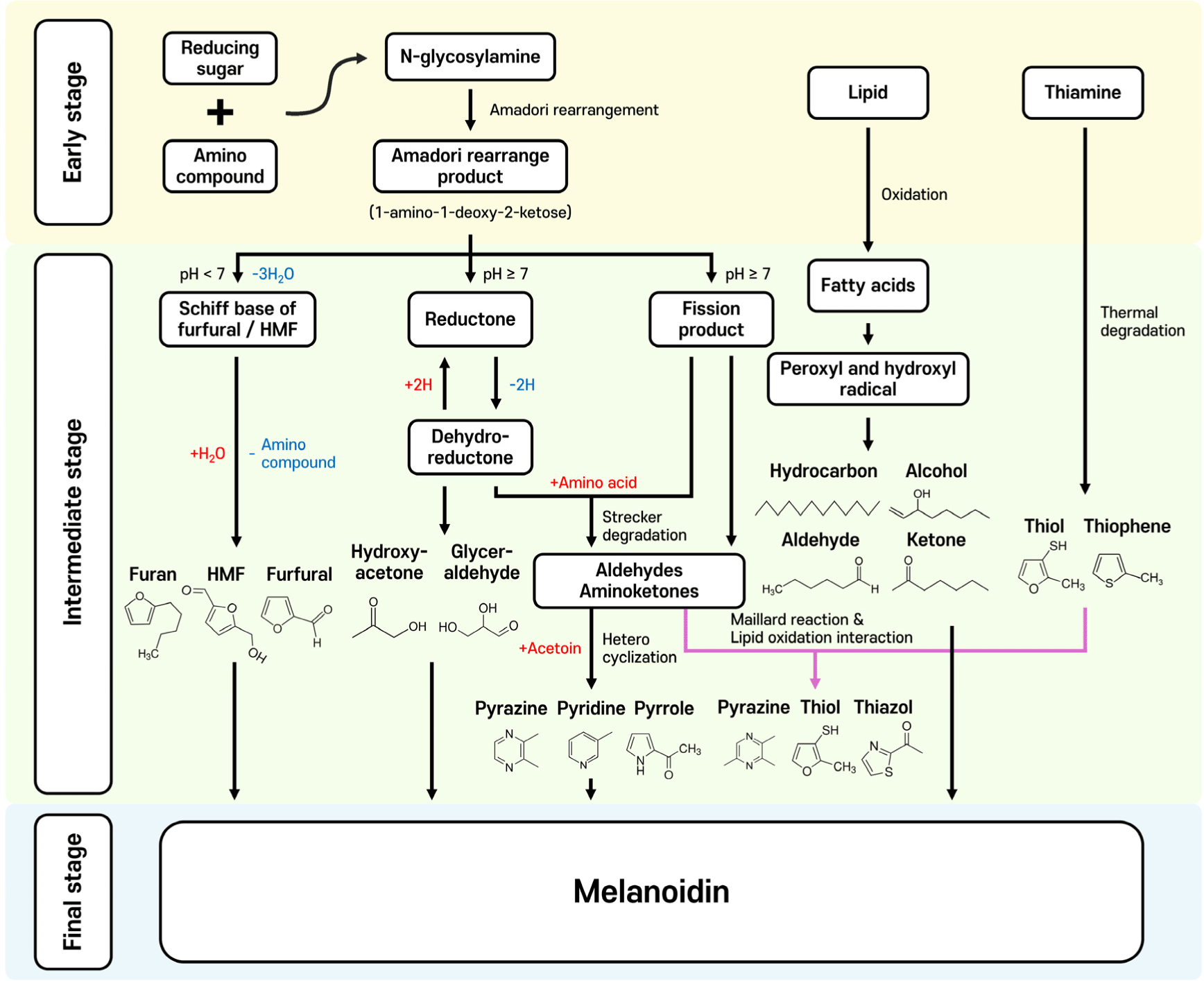
Additionally, the products from the Maillard reaction and lipid oxidation or thiamine degradation can interact directly or indirectly, affecting the overall VOC composition through the alteration of various nitrogen- and sulfur-containing heterocyclic compound contents that are responsible for meaty and roasted flavor (Liu et al., 2023).
During the Maillard reaction, many VOCs are formed through the fragmentation and degradation of flavor precursors (amino acids and reducing sugars) and their interactions (Fig. 1). Among them, it is generally recognized that oxygen-, nitrogen-, and sulfur-containing heterocyclic compounds are major contributors to the meaty flavor of MRPs. Table 1 summarizes the list of VOCs that are frequently identified in the animal resources-based model systems.
No. | Compound | Odor characteristics | References |
---|---|---|---|
Acids | |||
1 | Acetic acid | Sour, pungent | 1, 3, 14, 15, 19, 20, 23, 25, 26, 30 |
2 | Benzoic acid | 14, 19, 25, 26 | |
3 | Butanoic acid | Cheesey | 1, 14, 23, 25 |
4 | Decanoic acid | 3, 14, 16, 20, 24, 25 | |
5 | Hexanoic acid | Fatty, pungent, sweet | 1, 3, 7, 8, 13, 18, 20, 21, 24, 25 |
6 | Heptanoic acid | Cheesy, fatty, sour, rancid | 1, 4, 7, 13, 21, 24, 25 |
7 | Nonanoic acid | Cheesy, diary, waxy | 1, 3, 6, 7, 13, 14, 16, 17, 21, 24, 25 |
8 | Octanoic acid | Cheesy, fatty, greasy, oily, pungent, rancid | 1, 4, 6, 7, 8, 13, 14, 15, 16, 20, 21, 24, 25 |
9 | Pentanoic acid | Acidic, sweaty, rancid | 3, 6, 7, 24, 25 |
10 | Propionic acid | Beany | 23, 25, 26 |
11 | 3-Methylbutanoic acid | 13, 23, 26 | |
Alcohols | |||
12 | Benzyl alcohol | 9, 11, 13, 14 | |
13 | 1-Heptanol | Fruity, nutty, waxy | 2, 7, 9, 13, 14, 15, 17, 18, 19, 21, 24, 25 |
14 | 1-Hexanol | Fermented, green, oily | 2, 4, 7, 8, 9, 11, 13, 14, 15, 18, 24 |
15 | 1-Octanol | Citrus, green, fatty, fruity, mushroom, pungent, waxy | 2, 3, 4, 7, 9, 11, 13, 14, 15, 18, 19, 20, 21, 22, 23, 24, 25, 26, 28 |
16 | 1-Octen-3-ol | Earthy, meaty, mushroom, oily | 2, 3, 4, 8, 9, 11, 13, 15, 17, 18, 19, 21, 24, 25, 28 |
17 | 1-Pentanol | Baked, oily, pungent | 2, 4, 7, 8, 11, 13, 14, 15, 18, 19, 24 |
18 | 1-Penten-3-ol | 9, 13, 15 | |
19 | 2-Ethyl-1-hexanol | Citrus, oily, sweet | 4, 9, 13, 15, 17 |
20 | 2-Furanmethanol | Burnt | 1, 2, 13, 23 |
Aldehydes | |||
21 | Benzaldehyde | Almond, nutty, oily | 1, 2, 4, 5, 6, 8, 9, 10, 11, 13, 14, 15, 16, 17, 20, 22, 23, 25, 26, 28 |
22 | Benzeneacetaldehyde | Fermented, flowery, fruity, sweet | 3, 4, 6, 8, 10, 25, 26 |
23 | Decanal | Citrus, pungent, soapy, tallowy, waxy | 2, 3, 4, 13, 16, 17, 18, 19, 28 |
24 | Heptanal | Fatty, fresh, fruity, green, oily | 2, 3, 4, 7, 8, 9, 11, 13, 14, 15, 17, 18, 19, 21, 22, 24, 26, 29 |
25 | Hexadecanal | 4, 13, 16 | |
26 | Hexanal | Grass, green | 2, 4, 6, 8, 9, 13, 14, 15, 17, 18, 19, 20, 21, 22, 25, 26, 28 |
27 | Methional | Earthy, potato | 4, 8, 25, 30 |
28 | Nonanal | Citrus, fatty, green, nutty, soapy, waxy | 2, 3, 4, 7, 8, 9, 11, 13, 14, 16, 17, 18, 19, 21, 22, 24, 25, 28 |
29 | Octanal | Citrus, fatty, fruity, pungent, sour | 2, 3, 4, 7, 11, 13, 14, 15, 16, 18, 19, 21, 22, 23, 24, 25 |
30 | Pentanal | Baked, fermented | 2, 4, 8, 9, 15, 17, 18, 19 |
31 | Tetradecanal | Creamy, greasy | 4, 16, 17 |
32 | Undecanal | Fatty, pungent, waxy | 4, 13, 16, 17, 22 |
33 | 2-Dodecenal | Fatty, pungent | 18, 19, 25 |
34 | 2-Methylbutanal | Cocoa, coffee, nutty | 4, 8, 9, 15, 23 |
35 | 2-Methylpropanal | Chocolate, fresh, malty, pungent | 4, 6, 9, 10 |
36 | 2-Octenal | 8, 15, 20 | |
37 | 3-Methylbutanal | Chocolate, fatty, greasy, malty | 4, 6, 9, 10, 15, 17, 18, 20, 22, 30 |
38 | (E)-2-Decenal | Earthy, fatty, greasy, waxy | 2, 3, 4, 11, 13, 17, 18, 21, 24 |
39 | (E)-2-Heptenal | Fatty | 2, 15, 18, 19, 21, 24 |
40 | (E)-2-Hexenal | 2, 13, 19, 24 | |
41 | (E)-2-Nonenal | Cucumber, fatty, greasy, green, tallowy | 2, 3, 4, 11, 18, 19, 22, 24 |
42 | (E)-2-Octenal | Greasy, green, herbal | 2, 3, 4, 6, 18, 19, 21, 22, 24 |
43 | (E)-2-Undecenal | Fresh, fruity | 3, 4, 24 |
44 | (E,E)-2,4-Decadienal | Chicken, fatty, fried, greasy | 2, 3, 4, 9, 11, 12, 13, 19, 21, 24 |
45 | (E,E)-2,4-Heptadienal | 9, 19, 21, 24 | |
46 | (E,E)-2,4-Hexadienal | Fatty, green | 9, 18, 24 |
47 | (E,E)-2,4-Nonadienal | Fatty, waxy | 2, 3, 9, 11, 12, 13, 19, 21, 24 |
Ester | |||
48 | Ethyl acetate | Fruity | 6, 15, 26 |
Furans | |||
49 | Furfural | Almond, baked, caramel, sweet | 1, 6, 7, 8, 9, 10, 11, 12, 13, 14, 15, 18, 20, 22, 27, 28, 29 |
50 | 2-Acetylfuran | Almond, balsamic, nutty, sweet | 4, 14, 15, 18, 20, 23, 27, 29 |
51 | 2-Butylfuran | Spicy, sweet, winey | 6, 8, 10, 12, 22, 24, 26 |
52 | 2-Ethylfuran | Burnt, earthy, malty, sweet | 6, 8, 9 |
53 | 2-Furanmethanol | Caramel, roasted | 10, 18, 25 |
54 | 2-Heptylfuran | 7, 8, 21, 28 | |
55 | 2-Hexylfuran | 2, 4, 7, 8, 11, 24, 28 | |
56 | 2-Methylfuran | Chocolate, ethereal | 1, 4, 7, 9, 11, 14, 20 |
57 | 2-Octylfuran | 6, 7, 8, 21, 24, 25 | |
58 | 2-Pentylfuran | Buttery, earthy, fatty, green bean, meaty | 2, 3, 4, 6, 7, 8, 9, 11, 13, 14, 15, 18, 19, 20, 21, 22, 24, 25, 26, 28 |
59 | 3-Phenylfuran | 1, 4, 5, 6 | |
Hydrocarbons | |||
60 | Dodecane | 3, 4, 5, 6, 19 | |
61 | Tetradecane | 3, 4, 5, 6, 9, 17, 19, 28 | |
62 | Toluene | Paint | 5, 19, 23, 29 |
Ketones | |||
63 | Acetophenone | Almond, hawthorn | 13, 23, 25 |
64 | 1-Octen-3-one | Earthy, herbal, mushroom | 6, 15, 19 |
65 | 2-Butanone | Camphor, ether, fruity, sweet | 4, 10, 14, 15, 23 |
66 | 2-Decanone | Floral, greasy | 3, 4, 21, 24, 26 |
67 | 2-Heptanone | Fatty, fruity, soapy, sweet | 3, 4, 7, 8, 9, 14, 17, 18, 24, 25, 26, 28 |
68 | 2-Nonanone | Fruity, herbal, musty, soapy, sweet | 3, 4, 11, 13, 14, 16, 18, 21, 22, 25, 26 |
69 | 2-Octanone | Earthy, fruity, herbal | 2, 3, 4, 6, 7, 13, 14, 17, 20, 25 |
70 | 2-Pentanone | Fruity, sweet | 14, 22, 23 |
71 | 2-Undecanone | Fatty, fruity, oily | 4, 11, 13, 24, 26 |
72 | 2,3-Octanedione | Asparagus, coriander | 2, 4, 26 |
73 | 2,3-Pentanedione | Almond, buttery, fruity, toasted | 4, 14, 22, 23 |
74 | 3-Octanone | Cheesy, fresh, mushroom, musty, sweet | 4, 6, 26 |
75 | 5-Methyl-2-hexanone | Sweet | 1, 22, 28 |
Phenol | |||
76 | Phenol | 13, 14, 15 | |
Pyrazines | |||
77 | Pyrazine | Nutty | 10, 20, 26, 29 |
78 | Methylpyrazine | Nutty, roasted | 2, 9, 10, 23 |
79 | Tetramethylpyrazine | Chocolate, meaty, roasted | 3, 10, 25 |
80 | Trimethylpyrazine | Baked, nutty, roasted | 1, 3, 10, 14, 23 |
81 | 2-Ethyl-5-methylpyrazine | Popcorn | 1, 2, 10, 25 |
82 | 2-Ethyl-6-methylpyrazine | 1, 3, 25 | |
83 | 2-Methylpyrazine | Chocolate, meaty, nutty, roasted | 1, 4, 14, 18, 25, 26, 29 |
84 | 2,3-Dimethylpyrazine | Popcorn, roasted | 10, 14, 23, 26 |
85 | 2,5-Dimethylpyrazine | Chocolate, cocoa, creamy, meaty, nutty, popcorn, roasted | 1, 4, 5, 10, 12, 14, 18, 23, 25, 26 |
86 | 2,6-Dimethylpyrazine | Chocolate, meaty, nutty, roasted | 1, 2, 9, 14, 15, 23, 25, 26 |
87 | 3-Ethyl-2,5-dimethylpyrazine | Nutty, roasted | 1, 9, 10, 16, 23, 25, 26 |
88 | 3,5-Diethyl-2-methylpyrazine | Baked | 1, 16, 23 |
Pyridine | |||
89 | Pyridine | Burnt, roasted | 10, 12, 13, 29 |
Pyrroles | |||
90 | Pyrrole | Nutty, pungent | 14, 15, 25 |
91 | 1-Furfurylpyrrole | Caramel, coffee, nutty, toasted | 1, 4, 14, 25 |
92 | 2-Acetylpyrrole | Nutty | 1, 18, 20, 23, 27 |
Thiazoles | |||
93 | Thiazole | Fishy, meaty, nutty | 4, 11, 20, 27, 29 |
94 | 2-Acethylthiazole | Meaty, nutty, roasted, toasted | 4, 10, 20 |
95 | 4-Methylthiazole | Green, nutty, vegetable | 4, 9, 20 |
Thioethers | |||
96 | Bis(2-methyl-3-furyl)disulfide | Meaty, roasted, sulfurous | 4, 6, 20, 30 |
97 | Dimethyl disulfide | Onion, sulfurous | 1, 5, 6, 7, 14, 28, 30 |
98 | Dimethyl trisulfide | Alliaceous, sulfurous | 1, 5, 6, 14, 30 |
Thiols | |||
99 | 2-Furfurylthiol | Burnt, chocolate, coffee, meaty, roasted, toasted | 4, 6, 7, 11, 16, 17, 22, 25, 27, 28, 29, 30 |
100 | 2-Methyl-3-furanthiol | Coffee, meaty, sulfurous | 4, 6, 7, 11, 16, 17, 20, 22, 23, 25, 27, 28, 29, 30 |
Thiophenes | |||
101 | 2-Ethylthiophene | Meaty, styrene | 18, 22, 27 |
102 | 2-Hexylthiophene | Green, meaty | 11, 27, 28 |
103 | 2-Methylthiophene | Onion, sulfurous | 18, 20, 22, 23, 27, 30 |
104 | 2-Pentylthiophene | Fatty, fruity, green, meaty, sweet | 18, 27, 28 |
105 | 2-Thiophenecarboxaldehyde | Sulfurous | 6, 18, 22 |
106 | 2,5-Dimethylthiophene | Nutty, sulfurous | 6, 18, 27 |
107 | 3-Methylthiophene | Greasy, sulfurous, winy | 4, 6, 7, 11, 18, 30 |
108 | 3-Thiophenecarboxaldehyde | Meaty, roasted, sulfurous | 6, 10, 11, 22, 25 |
109 | 5-Methyl-2-thiophenecarboxaldehyde | Meaty, roasted, sulfurous | 10, 18, 23, 27, 30 |
References: 1. Chiang et al. (2019); 2. Chiang et al. (2022); 3. Cui et al. (2022); 4. de Sousa Fontes et al. (2024); 5. Dong et al. (2019); 6. Gao et al. (2020); 7. Lee et al. (2024); 8. Li and Liu (2021); 9. Li et al. (2021b); 10. Liu et al. (2015); 11. Ma et al. (2020); 12. Normah and Noorasma (2018); 13. Song et al. (2012); 14. Song et al. (2016); 15. Song et al. (2017); 16. Sun et al. (2014); 17. Wang et al. (2019); 18. Wei et al. (2020); 19. Xia et al. (2021); 20. Xiao et al. (2015); 21. Xu et al. (2011); 22. Xu et al. (2013); 23. Xu et al. (2019); 24. Yang et al. (2015); 25. Ye et al. (2022); 26. Zhang et al. (2020); 27. Zhao et al. (2019); 28. Zheng et al. (2022); 29. Zhou et al. (2019); 30. Zou et al. (2019).
Oxygen-containing heterocyclic compounds include furans, furanones, and furfural. A major pathway for the formation of oxygen-containing heterocyclic compounds is sugar degradation (Wei et al., 2020). Amadori rearrange products are also the precursors of oxygen-containing heterocyclic compounds through 1,2-enolization and cyclization steps (Zheng et al., 2023). Specifically, acidic condition favors the formation of furans (Xu et al., 2011). Furfural, a representative VOC in the Maillard reaction, has a strong caramel and sweet flavor (Lotfy et al., 2021). 2-Pentylfuran is another compound that is frequently found in the animal resources-based model system and elicits a meaty and beany flavor (Song et al., 2017). Owing to their strong flavors, oxygen-containing heterocyclic compounds greatly influence the flavor of MRPs.
The formation of sulfur-containing heterocyclic compounds such as thiols and thiophenes during the Maillard reaction is mainly attributed to the reaction of H2S that is released from sulfur-containing amino acids such as cysteine and methionine. These compounds are mainly responsible for the typical meaty and roasted flavor (Lee et al., 2021). However, excessive concentrations of sulfur-containing heterocyclic compounds may negatively affect the sensory attributes due to the strong sulfurous and burnt odors (Xu et al., 2011). Major compounds found in the model system are 2-methyl-3-furanthiol, 2-furfurylthiol, and 2-methyl-3-(methylthio)furan (Huang et al., 2023; Zou et al., 2019).
Pyrazines, pyridines, and pyrroles are classified as nitrogen-containing heterocyclic compounds. These compounds are responsible for meaty, roasted, nutty, and savory flavors (Lee et al., 2021). In contrast to furans, high pH condition favors the formation of nitrogen-containing heterocyclic compounds (de Sousa Fontes et al., 2024). Additionally, a high heating temperature is necessary for the interaction between amines and α-dicarbonyls through the Strecker degradation to produce nitrogen-containing heterocyclic compounds (Liu et al., 2015).
In addition, aldehydes may account for a large portion depending on the type and composition of precursors within the model (Wei et al., 2020). Aldehydes are derived from lipid oxidation during thermal treatment or Strecker degradation (Liu et al., 2023; Zheng et al., 2022). These VOCs elicit species-specific fatty odors (Shen et al., 2021). Therefore, aldehydes are regarded as important VOCs in the formation of meat flavor. Other kinds of VOCs e.g. acids, alcohols, esters, and ketones, have high odor thresholds. However, these compounds have a complementary role in the overall flavor of MRPs and can be utilized as precursors for further reactions (Ye et al., 2022).
Maillard Reaction Model System Consisting of Animal Resources
A list of Maillard reaction model systems with animal resources in the previous literature is presented in Table 2. Most studies applied aquatic products, beef, chicken, and pork protein extracts or hydrolysates into the model system. In particular, enzymatic hydrolysates from animal by-products such as bones and fish heads are widely used for the model systems, as a direct application of these hydrolysates into the food industry is limited due to their bitter tastes and undesirable off-odors. The conversion of animal by-product hydrolysates into flavorful compounds through the Maillard reaction has been reported in many studies (Chiang et al, 2019; Sun et al., 2014; Zhang et al., 2020).
Amino compound | Carbohydrate | Lipid | Reaction condition | References |
---|---|---|---|---|
Amino compounds from a single source | ||||
Amino acid (Cys) | Glucose | Chicken fat | 140°C for 2 h (pH 5.5) | Yang et al. (2015) |
Amino acid (Cys) | Xylose | Lard | 120°C for 1 h (pH 5) | Xu et al. (2011) |
Amino acid (Cys) | Xylose | Mutton tallow | 120°C for 40 min | Ma et al. (2020) |
Amino acid (Cys) | Xylose | Tallow | 120°C for 90 min (pH 5.5) | Lee et al. (2024) |
Amino acid (Cys, Met) | Xylose | Tallow | 120°C for 2 h (pH 7) | Ye et al. (2022) |
Gelatin (beef) | Ribose | - | 95°C for 15–60 min | Tan et al. (2012) |
Gelatin (pork) | Ribose | - | 95°C for 15–60 min | Tan et al. (2012) |
Hydrolysates (shrimp) | Arabinose, fructose, glucose, ribose, xylose | - | 55°C for 6–24 h (pH 6.5) | Laroque et al. (2008) |
Hydrolysates (porcine plasma protein) | Fructose, galactose, glucose | - | 95°C for 30–360 min | Liu et al. (2014) |
Hydrolysates (chicken bone) | Galactose | - | 100°C for 90 min | Nie et al. (2017) |
Hydrolysates (fish skin gelatin) | Galactose | - | 70°C for 36 h | Karnjanapratum et al. (2016) |
Hydrolysates (glycosylated bovine bone collagen) | Galactose | - | 95°C for 150 min (pH 9) | Qi et al. (2024) |
Hydrolysates (glycosylated chicken bone collagen) | Galactose | - | 95°C for 150 min (pH 9) | Qi et al. (2024) |
Hydrolysates (glycosylated porcine bone collagen) | Galactose | - | 95°C for 150 min (pH 9) | Qi et al. (2024) |
Hydrolysates (porcine plasma protein) | Galactose | - | 95°C for 30–360 min | Liu et al. (2016) |
Hydrolysates (chicken liver) | Galactose, glucose, fructose, maltose, sucrose, xylose | - | 90°C for 130 min (pH 8) | Xiong et al. (2020) |
Hydrolysates (salmon frame) | Glucosamine | - | 120°C for 60 min | Sharma et al. (2024) |
Hydrolysates (chicken bone extract) | Glucose | - | 100°C for 2 h (pH 6.5) | Chiang et al. (2022) |
Hydrolysates (grass carp bone) | Glucose | - | 120°C for 1 h (pH 6–8) | Li et al. (2021b) |
Hydrolysates (half-fin anchovy) | Glucose | - | 120°C for 100 min (pH 9) | Song et al. (2018) |
Hydrolysates (shrimp by-product) | Glucose | - | 110°C for 10 h (pH 6.5) | Zha et al. (2015) |
Hydrolysates (chicken bone) | Glucose, xylose | - | 113°C for 10 min (pH 6.5) | de Souza Cunha et al. (2023) |
Hydrolysates (mechanically deboned chicken residue) | Glucose, xylose | - | 90°C–120°C for 1 h | Sun et al. (2010) |
Hydrolysates (mussel) | Glucose, xylose | - | 115°C for 2 h (pH 6) | Dong et al. (2019) |
Hydrolysates (anchovy) | Ribose | - | 110°C for 30 min (pH 7) | Su et al. (2016) |
Hydrolysates (bovine bone) | Ribose | - | 113°C for 10 min (pH 6.5) | Chiang et al. (2019) |
Hydrolysates (bovine bone) | Ribose | - | 113°C for 10 min (pH 6.5) | Chiang et al. (2020) |
Hydrolysates (flatfish by-product) | Ribose | - | 121°C for 38 min (pH 8.26) | Choe et al. (2016) |
Hydrolysates (chicken) | Ribose, xylose | - | 100°C for 3 h (pH 7.5) | Zeng et al. (2017) |
Hydrolysates (antarctic krill) | Xylose | - | 105°C for 50 min (pH 8.5) | Zhang et al. (2020) |
Hydrolysates (beef tallow) | Xylose | - | 100°C for 1 h | Cui et al. (2022) |
Hydrolysates (bovine bone marrow) | Xylose | - | 120°C for 70 min | Xu et al. (2018) |
Hydrolysates (chicken liver) | Xylose | - | 120°C for 90 min (pH 7) | Chen et al. (2021) |
Hydrolysates (fish head) | Xylose | - | 150°C–190°C for 1–2 h | Gao et al. (2020) |
Hydrolysates (fermented pork) | Xylose | - | 100°C for 60 min (pH 5.5) | Li and Liu (2022b) |
Hydrolysates (goat protein) | Xylose | - | 121°C for 1 h (pH 4 or 6) | de Sousa Fontes et al. (2024) |
Hydrolysates (mud clam) | Xylose | - | 120°C for 2 h (pH 7.4) | Normah and Noorasma (2018) |
Hydrolysates (pork trimmings) | Xylose | - | 90°C–100°C for 1 h (pH 5.5) | Li and Liu (2021) |
Hydrolysates (Takifugu obscurus) | Xylose | - | 120°C for 2 h (pH 7.4) | Wang et al. (2019) |
Hydrolysates (tuna viscera) | Xylose | - | 120°C for 1 h | Boonbumrung et al. (2023) |
Hydrolysates (yeast fermented pork) | Xylose | - | 100°C for 1 h (pH 5.5) | Li and Liu (2022a) |
Meat (beef) | Ribose | - | 95°C for 20–100 min | Akbarabadi et al. (2020) |
Meat (chicken) | Ribose | - | 95°C for 1 h | Tan et al. (2013) |
Meat (pork) | Ribose | - | 95°C for 1 h | Tan et al. (2013) |
Meat (crocodile) | Xylose | - | 100°C for 192 min (pH 12) | Li et al. (2021a) |
Peptides (chicken) | Glucose | - | 90°C–150°C for 30–240 min | Bai et al. (2017) |
Peptides (glutathione) | Glucose | Chicken fat | 140°C for 5 h (pH 6.5) | Zhao et al. (2019) |
Peptides (chicken) | Ribose | - | 180°C for 2 h (pH 6.3) | Zhou et al. (2019) |
Peptides (smooth hound hydrolysates) | Sucrose | - | 90°C for 120 min | Abdelhedi et al. (2017) |
Peptides (beef hydrolysates) | Xylose | - | 125°C for 2 h (pH 5.5) | Zou et al. (2019) |
Peptides (chicken) | Xylose | - | 80°C–140°C for 30–120 min (pH 6.5) | Liu et al. (2015) |
Powder (mussel) | Fructose, glucose, ribose, xylose | - | 100°C–140°C for 60–150 min | Xin et al. (2022) |
Powder (oyster) | Galactose, glucose, xylose | - | 120°C for 30–150 min (pH 7.0-7.2) | Fu et al. (2023) |
Protein (porcine plasma) | Fructose, galactose, glucose | - | 100°C for 1–5 h | Benjakul et al. (2005) |
Amino compounds from multiple sources | ||||
Amino acid (Cys)+hydrolysates (bovine bone marrow) | Glucose, xylose | - | 110°C for 1 h | Xu et al. (2019) |
Amino acid (Cys)+hydrolysates (HVP) | Glucose, xylose | Lard | 105°C for 1 h (pH 6) | Song et al. (2017) |
Amino acid (Cys, Met)+ hydrolysates (bovine bone+HVP) | Glucose, xylose | Tallow | 110°C for 100 min (pH 5.5–8.0) | Chai et al. (2018) |
Amino acid (Cys, Glu, Met, Pro)+hydrolysates (beef+HVP) | Glucose, xylose | Tallow | 120°C for 2 h (pH 6.5) | Song et al. (2012) |
Amino acid (Cys, Glu, Met, Pro)+hydrolysates (beef+HVP) | Glucose, xylose | Tallow | 110°C for 2 h (pH 6.5) | Song et al. (2014) |
Amino acid (β-Ala, Cys, Gly)+ hydrolysates (chicken breast) | Glucose, xylose | - | 105°C for 1 h (pH 6.5) | Xiao et al. (2015) |
Amino acid (Cys)+hydrolysates (beef) | Xylose | - | 125°C for 2 h (pH 5.5) | Kang et al. (2019) |
Amino acid (Cys)+hydrolysates (bovine bone) | Xylose | - | 110°C for 90 min (pH 7) | Song et al. (2016) |
Amino acid (Cys)+hydrolysates (bovine bone) | Xylose | Tallow, phospholipid | 115°C for 2 h (pH 6.5) | Zheng et al. (2022) |
Amino acid (Cys)+hydrolysates (bovine bone marrow) | Xylose | - | 120°C for 1 h | Begum et al. (2020) |
Amino acid (Cys)+hydrolysates (chicken) | Xylose | - | 100°C for 2 h (pH 6.5) | Zhang et al. (2019) |
Amino acid (Cys)+hydrolysates (chicken bone) | Xylose | - | 105°C for 90 min (pH 7) | Wang et al. (2016) |
Amino acid (Cys)+hydrolysates (chicken bone extract) | Xylose | - | 105°C for 30–90 min (pH 7) | Sun et al. (2014) |
Amino acid (Cys)+hydrolysates (flaxseed protein) | Xylose | Chicken fat | 120°C for 2 h (pH 7.5) | Wei et al. (2020) |
Amino acid (Cys)+hydrolysates (peony seed meal protein) | Xylose | Chicken fat | 120°C for 2 h (pH 7.5) | Xia et al. (2021) |
Amino acid (Cys)+peptide (beef) | Xylose | - | 140°C for 90 min (pH 4.8) | Xu et al. (2013) |
The resultant MRPs possessed a variety of VOCs, including representative VOCs responsible for meat flavor such as 2-furfurylthiol, 2-methyl-3-furanthiol, furfural, 2-pentylfuran, 2,5-dimethylpyrazine, and hexanal (Table 1). Depending on the content of sulfur-containing amino acids in the animal protein hydrolysates used, there might be a need for additional amino acid input into the model system to better develop a complex and long-lasting meat flavor (de Sousa Fontes et al., 2024).
A lot of by-products from aquatic products such as fish heads, bones, and skins are discarded in the industry (Gan et al., 2022). Instead of processing these by-products into animal feed ingredients or fertilizers that have a low market value, upcycling them into value-added food ingredients through enzymatic hydrolysis is regarded as an effective strategy (Li and Liu, 2021). However, a strong bitter taste, unpleasant fishy odor, and off-flavor of fish hydrolysates are limiting factors for their application as flavorings (Dong et al., 2019; Gao et al., 2020; Li et al., 2021b). To address this issue, the utilization of aquatic products protein hydrolysates in the Maillard reaction has been widely conducted.
The application of Antarctic krill hydrolysates into the model system was conducted by Zhang et al. (2020). An Antarctic krill contains a high fluorine content of which continuous consumption may provoke osteosclerosis, renal disease, or allergies. However, the bitterness of the defluorinated Antarctic krill hydrolysates makes them unacceptable to consumers. The Maillard reaction of Antarctic krill hydrolysates with xylose successfully enhanced the sweet, roasted, shrimp meat aromas, owing to the generation of pyrazines which were the main VOCs in the MRPs, along with aldehydes and ketones. Dong et al. (2019) applied ultrasonic-assisted thermal treatment for the production of mussel meat hydrolysates-based MRPs in an attempt to reduce the bitter taste of the mussel. Heating for 90 min effectively suppressed the bitter taste and increased the pleasant meat and fishy odor intensities of MRPs. In another study conducted by Li et al. (2021b), the grass carp bone hydrolysates-based MRPs showed an increased ratio of umami-sweet taste amino acid to total amino acid, which could lead to the flavor enhancement of the hydrolysates. Furthermore, after the Maillard reaction, the kinds of aldehydes, alcohols, ketones, pyrazines, and other VOCs increased, which may contribute to the masking effect of fishy off-odor. Meanwhile, the flavor of protein hydrolysates from aquatic products can be improved by adding cysteine and xylose into the Maillard reaction model system. Normah and Noorasma (2018) investigated the effect of the addition of cysteine and xylose on the flavor of mud clam hydrolysates and found that the additional cysteine and xylose significantly strengthened the meaty flavor, umami and caramel taste due to the increased amount of sulfur-containing heterocyclic compounds, furfural, and umami amino acids, and decreased the fish off-odor and bitter taste intensity of the MRPs. Gao et al. (2020) added fermented tilapia fish head hydrolysate into the cysteine-xylose model system and optimized the reaction condition through response surface methodology based on the production of 2-methyl-3-furanthiol and 2-furfurylthiol, which are two important VOCs in exhibiting meaty flavor. The authors suggested that the flavor of fish hydrolysates could be improved by the production of sulfur-containing heterocyclic compounds via the Maillard reaction.
A few studies used beef hydrolysates derived from the hydrolysates in the model system (Akbarabadi et al., 2020; Kang et al., 2019; Song et al., 2012). Zou et al. (2019) revealed that specific peptides such as Cys-Gly-Val from beef hydrolysates acted as effective precursors for key VOCs like 2-methyl-3-furanthiol and 4-butyl-2,5-dimethylthiazole, significantly enhancing roasted and meaty flavors. However, a major source of beef protein hydrolysates that has been universally used in the previous literature is bovine bone, a high volume of meat by-product found in the industry (Chiang et al., 2019; Song et al., 2016; Xu et al., 2018; Zheng et al., 2022). Bovine bone marrow extract, or bone collagen, contains approximately 20%–30% of protein content (Song et al., 2016; Xu et al., 2019). Owing to their abundant protein content, the utilization of bovine bone hydrolysates in the Maillard reaction can contribute to the production of value-added products.
Xu et al. (2019) compared the sensory attributes of two MRPs that contained hydrolyzed or unhydrolyzed bovine bone marrow extracts. The results revealed that 35 VOCs were found in the former, whereas only 22 VOCs were identified in the latter. Furthermore, the overall content of the VOCs and the intensity of meaty aroma were much higher in the MRPs prepared with hydrolyzed bovine bone marrow extracts, suggesting that moderate hydrolysis is essential for bovine bone marrow extract. Similarly, Begum et al. (2020) investigated the effect of the Maillard reaction on the flavor development of bovine bone marrow extracts and their hydrolysates. The authors observed that the meaty, roasted, and caramel aromas and umami, kokumi, and mellow tastes were significantly enhanced in MRPs when the bovine bone marrow extracts were enzymatically hydrolyzed. On the other hand, the undesirable fishy and sulfurous aromas and bitter taste were weakened.
In addition to muscle and bone protein hydrolysates, beef tallow is a great precursor that significantly contributes to the formation of beefy flavor in MRPs. A different percentage from 2% to 20% of enzymatically hydrolyzed beef tallow was added into the model system containing bovine bone hydrolysates, hydrolyzed vegetable proteins, and yeast extract and heated at 110°C for 100 min (Chai et al., 2018). The authors reported that the intensities of meaty and beefy flavors were the strongest while that of off-flavor was the weakest in the MRPs when containing 10% of beef tallow hydrolysates, whereas additional tallow increased tallowy flavor but decreased beefy flavor. Zheng et al. (2022) investigated the role of phospholipids on the flavor of the bovine bone protein hydrolysate-based MRPs. The results showed that the furan, thiophene, thioethers, and alcohol contents were significantly higher when the model system included phospholipids, suggesting that phospholipids could influence the VOC composition and the sensory characteristics of MRPs.
Chicken meat is a widely available meat product. Chicken meat has a strong umami taste due to the high content of umami amino acids and flavor nucleotides (Jayasena et al., 2013). Owing to this advantage, chicken meat is also a great participant in the improvement of the umami taste of MRPs. Zhang et al. (2019) prepared chicken peptide hydrolysates on the model system and reported the enhancement of boiled chicken flavor and umami taste, continuity, mouthfulness, and overall acceptance. Xiao et al. (2015) compared the effect of the chicken base content on the flavor of MRPs. Depending on the chicken base content, the intensity of caramel and meaty flavor, continuity, and umami taste of MRPs differed significantly, whereas the MRPs without chicken base exhibited the strongest off-flavors with the lowest sensory attributes abovementioned. This result confirms that the addition of chicken meat can be beneficial for meat flavor improvement.
Chicken bone proteins as well as chicken meat proteins have been frequently used for the model system due to the low cost (Chen et al., 2021). Conversely, in Asia and Australia, meat flavorings that were prepared through the Maillard reaction of chicken bone hydrolysates are regarded as a non-conventional food (Wang et al., 2016). Therefore, the processing of hydrolysates from chicken by-products would be promising for the production of profitable and marketable meat flavorings (Sun et al., 2014). The bitter taste of chicken bone hydrolysates could be reduced through the Maillard reaction which alters the free amino acid composition, ultimately affecting the taste of the products (Sun et al., 2014). Especially, the umami intensity of the chicken bone hydrolysate-based MRPs showed an increase until 60 min of reaction and then a decrease at 90 min.
Meanwhile, Chicken liver has a high cholesterol content and exhibits an unpleasant smell. Few studies utilized chicken liver protein hydrolysates on the Maillard reaction model systems (Chen et al., 2021; Xiong et al., 2020). Unfortunately, these studies mainly focused on the bioactivity of the MRPs rather than their organoleptic attributes. Future studies on the utilization of chicken liver protein as meat flavorings through the Maillard reaction would broaden the market value of chicken liver.
In addition to protein sources, chicken fat has been extensively studied using model systems due to its ability to amplify meat flavor (Wei et al., 2020; Xia et al., 2021). Zhao et al. (2019) demonstrated that the interactions between the degradation products of chicken fat and the intermediate products from the Maillard reaction generated unique VOCs including 2-pentylthiophene and 2-pentylpyridine that enriched stewed meat-like aromas. Similarly, Yang et al. (2015) showed that the inclusion of chicken fat in a cysteine-glucose reaction system suppressed the formation of sulfur-containing VOCs while promoting the formation of alkyl heterocyclic compounds and aliphatic aldehydes and ketones which led to the enhancement of species-specific flavor.
In terms of pork, bone proteins, plasma proteins, and gelatins as well as meat have been applied to the model system (Benjakul et al., 2005; Qi et al., 2024; Tan et al., 2012; Tan et al., 2013). However, only limited literature focused on the sensory attributes of porcine protein hydrolysates-based MRPs (Li and Liu, 2021; Li and Liu, 2022a; Li and Liu, 2022b). A major finding was the evident savory, roasted, meaty, fruity, and sweet flavors of the MRPs which contained yeast and/or lactic acid bacterium-fermented pork hydrolysates, with a dominant content of 2-furfurylthiol. The glycation of pork plasma and muscle hydrolysates through the Maillard reaction with glucosamine was shown to be effective in reducing their bitterness and increasing the favorable sensory characteristics (Fu et al., 2020).
Some researchers added lard to the model system to identify the role of lard in the formation of pork flavor in the MRPs. Song et al. (2017) found that aldehydes were the main compounds in the lard, such as hexanal, (Z)-2-decenal, and (E,E,)-2,4-decadienal. Lard, especially enzymatically hydrolyzed lard, showed a considerable effect on the increase in the meaty and porky aroma intensity. The increase in the lipid oxidation-derived VOCs such as alcohols, alkylfurans, and acids was observed by the addition of lard, on the other hand, it showed a suppression effect of the formation of sulfur-containing heterocyclic compounds. In a similar study conducted by Xu et al. (2011), the presence of lard in a cysteine-xylose model system had an effect on suppressing the formation of sulfur-containing VOCs while increasing the production of lipid oxidation-derived VOCs, which may have a benefit on balancing sulfurous and fatty and roasted characteristics, highlighting the key role of lard in modulating flavor profiles in the Maillard reaction systems.
Factors Affecting the Flavor of Maillard Reaction Products
The development of meat flavor through the Maillard reaction is greatly influenced by various parameters: source and concentration of the reactants, degree of lipid oxidation, heating temperature and time, initial pH, buffer type and concentration, etc. (Chiang et al., 2019; Shen et al., 2021; Zeng et al., 2017). It is essential to modulate the abovementioned parameters and select the suitable reaction condition to strengthen the desirable organoleptic attributes of MRPs while limiting the formation of off-flavor and toxic compounds (Fig. 2).
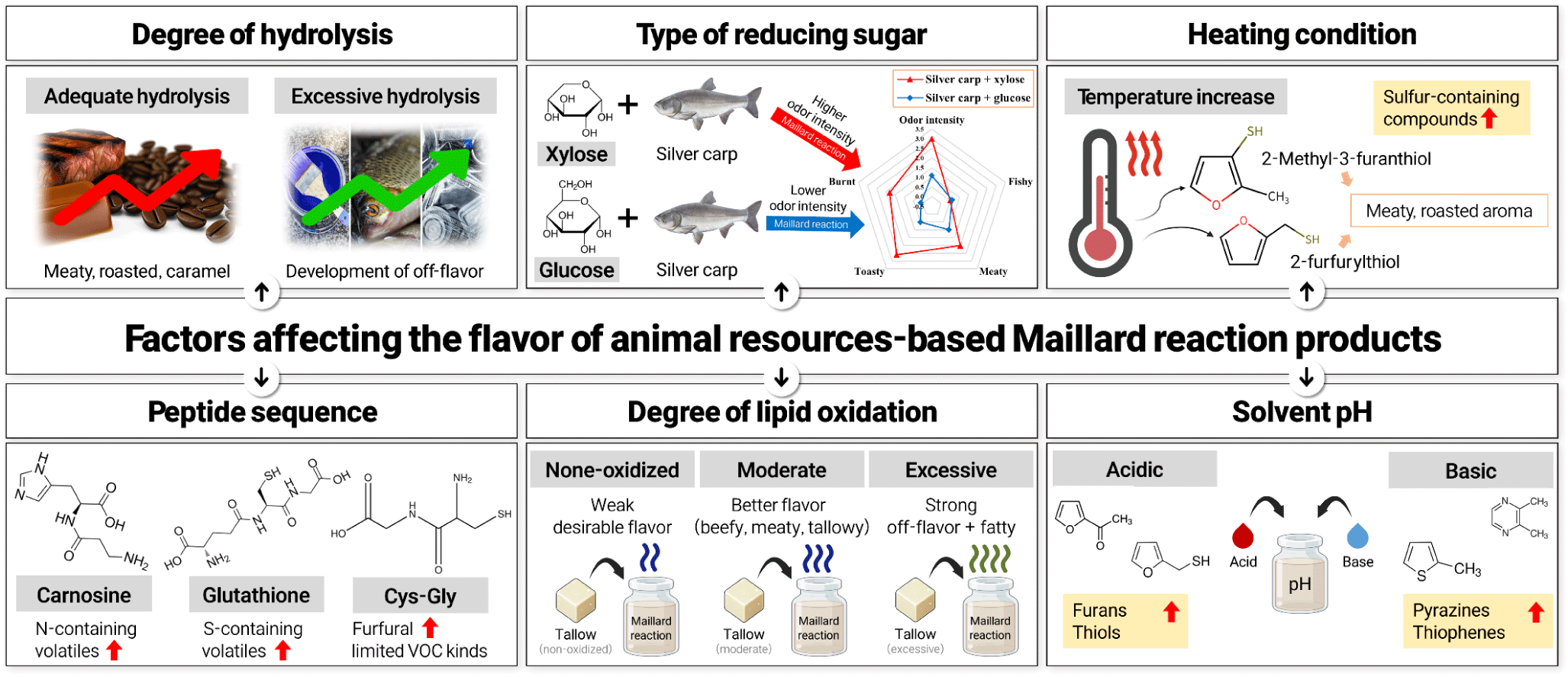
In a simple model system consisting of one or two kinds of pure amino acids and a reducing sugar, the type of amino acid determines the flavor characteristics of the MRPs (Aaslyng and Meinert, 2017; Wong et al., 2008). On the other hand, when the model system contains animal protein hydrolysates or their resulting peptides, the mechanism of the Maillard reaction is greatly influenced by the degree of hydrolysis, molecular weight, and amino acid composition and sequence of the peptides (Normah and Noorasma, 2018).
When MRPs are prepared with animal protein sources, enzymatic hydrolysis of protein is necessary to provide amino acids and peptides that are the main participants in the Maillard reaction. If the degree of hydrolysis of the sample is low, it would lead to a weak meat flavor of MRPs. On the other hand, MRPs containing animal protein hydrolysates with an excessive degree of hydrolysis may exhibit a strong burnt and sulfurous aroma which can hamper the perception of meaty flavor. Zhang et al. (2019) found that MRPs containing chicken protein hydrolysates prepared for 2–3 h of hydrolysis procedures showed the strongest boiled chicken flavor, mouthfulness, and overall acceptance. Xu et al. (2019) reported that the bovine bone marrow hydrolysate with a 10% degree of hydrolysis was the most effective in enhancing the meaty and roasted flavor and suppressing the formation of burnt aroma in MRPs.
Enzymatic hydrolysis of protein produces peptides with varying molecular weights. Many researchers observed that low-molecular-weight peptides at approximately 1–5 kDa or smaller are the main precursors for the production of meaty aroma, which is attributed to a large number of free amino groups with high reactivity toward reducing sugars (Nie et al., 2017). Kang et al. (2019) observed that MRPs containing beef hydrolysates with <1,000 Da showed higher intensity of meaty aroma, umami, and kokumi taste, whereas MRPs with beef hydrolysates >5,000 Da did not show any improvement in the meat flavor.
After hydrolyzing animal proteins, the resultant hydrolysates contain various kinds of amino acids and peptides. The reactivity of each amino acid may vary when they are present in the peptides (Xu et al., 2013). For example, cysteine releases H2S more easily when present as a free amino acid rather than as a peptide form (Zou et al., 2019). The authors also identified that within peptides, Cys-Gly-Val showed a better contribution to the meaty aroma, followed by glutathione, compared to Leu-Cys. It was revealed that the position of cysteine, leucine, isoleucine, or phenylalanine at the N- or C-terminus affects the reactivity of the peptides toward the Maillard reaction, which the former favors the reaction whereas the latter shows less reactivity (Kang et al., 2019). Meanwhile, leucine, isoleucine, valine, and phenylalanine in the peptide can participate in the Strecker degradation although these amino acids are bound to the peptide (Liu et al., 2015).
Finally, the different amino acid sequence of the peptide affects the formation of VOCs in MRPs. Zhou et al. (2019) observed that pyrazines and pyridines were generated in the model system consisting of carnosine, whereas sulfur-containing heterocyclic compounds instead of nitrogen-containing heterocyclic compounds were the main VOCs in the model systems which possessed glutathione or chicken protein-derived dipeptide Cys-Gly due to the presence of cysteine in the peptide sources. Xu et al. (2013) compared the resultant VOCs from the Maillard reaction of three beef protein peptides, Asp-Trp-Glu-Phe-Pro-Asp-Pro-Lys, Gly-Val-Thr-Val-Phe-Glu-Asp-Leu-Lys, and Glu-Thr-Asp-Asn-Leu-Asp-Ser-Val-Pro-Arg, and found that the formation of 2-furfurylthiol was facilitated only when the first peptide was put into the model system.
The content of reducing sugar in the meat hydrolysates is a trace level (Li and Liu, 2021). Without reducing sugars added, the model system containing animal protein hydrolysates would not exhibit strong meaty flavors (Chai et al., 2018). Therefore, the addition of reducing sugar is essential for provoking a desirable flavor. However, the rate of reaction differs significantly depending on the type of reducing sugar, due to the different chemical structure that determines its reactivity (Chiang et al., 2019; Liu et al., 2014; Zheng et al., 2022). It is well-documented that pentoses have a higher reaction rate than hexoses (Gao et al., 2020; Xu et al., 2013; Zou et al., 2019). In the study conducted by de Souza Cunha et al. (2023), the chicken bone hydrolysate-based MRPs with xylose showed a higher browning intensity and higher proportions of aldehydes such as decanal, octanal, pentanal, phenylacetaldehyde, (E)-2-octenal, (E,E)-2,4-decadienal compared to those with glucose. Among aldopentoses, ribose was found to be more reactive with shrimp hydrolysates during Maillard reaction compared to xylose and arabinose, partially due to the higher proportion of acyclic carbonyls with less stable ring form of ribose (Laroque et al., 2008). Meanwhile, the nature of reducing sugar also affects its reactivity. Mottram and Nobrega (2002) compared the reactivity of three forms of ribose and found that ribose 5′-monophosphate was the most reactive, followed by free ribose, and inosine 5′-monophosphate was the least reactive in the model system.
Compared to amino acids, the effect of reducing sugar on the sensory attributes of MRPs is less significant (Chiang et al., 2019; de Souza Cunha et al, 2023; Zheng et al., 2022). However, as the type of reducing sugar can largely influence the rate of the reaction and formation of VOCs, it is still important to consider which sugar should be used in the model system studies.
The addition of animal lipids into a model system results in a differentiated flavor characteristic of MRPs. In many cases, the heating condition for the Maillard reaction model system would not be intense enough to cause lipid oxidation, and therefore a fresh, non-oxidized lipid exists mainly as insoluble triglycerides, partially contributing to the overall meat flavor (Song et al., 2017; Ye et al., 2022). Therefore, a moderate degree of lipid oxidation is a prerequisite for animal fats to influence the overall flavor of MRPs. Nonetheless, excessive lipid oxidation should be avoided as it produces high content of lipid oxidation-derived VOCs, especially aldehydes such as hexanal, octanal, and nonanal which give an unpleasant odor at high concentrations (Ma et al., 2020; Song et al., 2012).
It was found that the choice of pre-oxidation method of animal lipid exhibited a significant effect on the sensory characteristics of MRPs. Recently, the combination of enzymatic hydrolysis and mild thermal treatment (below 100°C) proved its effectiveness in enhancing the overall flavor of meat. Ye et al. (2022) found that the addition of moderately-oxidized beef tallow through enzymatic hydrolysis combined with mild thermal oxidation (90°C for 3 h) enhanced the meaty, umami, fatty, and overall taste of the MRPs.
Additionally, oxidized animal fat has an inhibitory effect on the formation of sulfur-containing heterocyclic compounds during the Maillard reaction (Xia et al., 2021). In general, sulfur-containing heterocyclic compounds are highly associated with meaty and roasted flavor; however, at high concentrations, these compounds have strong sulfurous odors with unpleasant aromas (Xu et al., 2011). Following oxidation, the degradation products of lipids react with amino acids and attenuate the release of H2S, subsequently suppressing the formation of sulfur-containing heterocyclic compounds (Wang et al., 2022; Zhao et al., 2019). This, in turn, mitigates the strong sulfurous odors from sulfur-containing heterocyclic compounds and strengthens the evident meaty aroma (Yang et al., 2015). The improvement of the desirable flavor of MRPs with the addition of moderately-oxidized animal fats is highly attributed to the formation of sulfur-free oxygen-containing heterocyclic compounds which present caramel, sweet, fruity, and nutty aromas (Ye et al., 2022).
Ketones, which mainly derive from sugar degradation, can be formed at temperatures below 80°C (Liu et al., 2015; Sun et al., 2022). On the other hand, the formation of important VOCs including nitrogen-, oxygen-, and sulfur-containing heterocyclic compounds favors high temperatures above 100°C (Sohail et al., 2022). As the rate of Maillard reaction increases with the increase in heating temperature, a high-temperature heating (100°C–120°C) of the model system has been widely used to generate a strong, pleasant roasted, and meaty flavor. However, the Maillard reaction at an excessively high temperature (>150°C) may give rise to burnt and off-flavors with hazardous compounds including acrylamide, polyaromatic hydrocarbons, heterocyclic amines, benzenes, formaldehyde, etc. (Augustine and Bent, 2022; Bassam et al., 2022).
Chai et al. (2018) reported that MRPs from the bovine bone hydrolysates-based model system prepared at 90°C had low intensities of meaty, beefy, and tallowy flavor, but these flavor intensities then increased gradually as the temperature rose and peaked at 110°C. However, when the heating temperature exceeded 120°C, the burnt and off-flavor of the MRPs became noticeable. Similar results were reported in the chicken protein-based Maillard reaction model system (Liu et al., 2015). A low-temperature heating was beneficial for broth-like flavor with strong umami and kokumi tastes, whereas a higher temperature heating led to a dominant nutty/roasted aroma of MRPs. In a study conducted by Gao et al. (2020), the tilapia fish head hydrolysate-based MRPs contained more 2-furfurylthiol as the heating temperature increased from 150°C to 190°C.
Heating time is another factor that determines the VOC composition of MRPs. A balance between the production of desirable VOCs that elicit meaty and roasted flavors and the limitation of burnt odor, off-flavor, and bitterness can be achieved by carefully controlling the heating time of model systems. The taste of MRPs changes as the composition of amino acids and carbohydrates changes by the thermal degradation of animal peptides, caramelization, and the interaction between amino acids and reducing sugars, along with the effect of newly produced polymers by Maillard reaction (Dong et al., 2019; Sun et al., 2014). Commonly adopted heating time for animal resources-based model systems ranges from 60–120 min, or up to 180 min (Sun et al., 2010; Xin et al., 2022; Ye et al., 2022). Few studies conducted a very short (–30 min) or long period (300–600 min) of the thermal process for model systems (Chiang et al., 2019; Karnjanapratum et al., 2016; Su et al., 2016; Zhao et al., 2019).
When preparing an aqueous model system, either distilled water or buffer can be used. The rate of the Maillard reaction and subsequent formation of VOCs vary depending on the pH of the model system (Mottram and Nobrega, 2002). Specifically, the amino group of the amino acids becomes more protonated when the solvent is acidic, which decreases the interaction with carbonyl groups (de Sousa Fontes et al., 2024). Subsequently, an acidic pH condition favors the formation of furans and thiols, while suppressing the formation of pyrazines. On the other hand, higher pH catalyzes the reaction by promoting the formation of Schiff base, leading to the increased formation of VOCs, especially pyrazines and Strecker’s aldehydes (Lotfy et al., 2021).
In the case of using buffer solutions, the pH of the model systems ranged from 5.5 to 7.5 in most studies. In the study conducted by Chai et al. (2018), MRPs which contained bovine bone hydrolysates and were prepared at pH 6.5 and 7.0 showed dominant meaty, beefy, tallowy odors and low degree of off-flavor. Li et al. (2021b) applied response surface methodology to optimize the pH, heating temperature, and heating time for preparing grass carp bone protein hydrolysate-based MRPs, and found that the highest degree of graft would be obtained at initial pH of 7.07 when heated at 118.33°C for 1.75 h. de Sousa Fontes et al. (2024) compared two goat protein hydrolysates-based model systems with different pH adjustment, and observed that more acidic condition (pH 4) led to strengthened meaty aroma, whereas less acidic condition (pH 6) increased sweet, fatty, and goat aroma. On the other hand, a strong alkaline model system was adopted in some studies, e.g. pH 8.26 for flatfish byproduct hydrolysates; pH 8.5 for Antarctic krill hydrolysates; and pH 12 for crocodile meat protein sources (Choe et al., 2016; Li et al., 2021a; Zhang et al., 2020).
Conclusion
The Maillard reaction model system is an effective tool for understanding the mechanism of the reaction and developing the meat flavor or flavor additives from a mixture of amino acids and reducing sugars. Although animal resources are less highlighted than plant proteins as reactants in the model system, these precursors have great potential in terms of both academic research and industrial application due to their great contribution to meat flavor enhancement with animal by-products. Considerations should be made when animal resources are applied to the model system, such as the source of amino groups, type of reducing sugars, degree of lipid oxidation, and heating and solvent conditions. For now, these model systems face challenges in simulating meat flavor as the formation pathways of meat flavor in real meat are much more complex to elucidate. Future studies with a more complex combination of flavor precursors from animal resources in more diverse reaction conditions may broaden our understanding of the formation of meat flavor and provide an insight into the upcycling of animal by-products into high market-value products.