Introduction
White meat, such as chicken meat, is considered healthier than red meat because of its relatively lower fat, cholesterol, and iron contents (Jaturasitha et al., 2008). Unlike the global chicken meat consumption, the per capita consumption of poultry meat in Korea has risen by approximately 3 kg over the last few decades, based on a study by the Korean Ministry of Agriculture and Forestry. Globally, however, this hastily growing consumption of chicken meat is centered on a limited number of fast-growing broiler strains regulated by commercial breeding businesses in intensive fattening systems (Jaturasitha et al., 2008). On the other hand, a special attention in slow-growing chickens has been increasing recently since of the consumers’ demands for healthier food (Fanatico et al., 2007).
Despite the increasing circumstances of slow-growing chickens, Korean native chickens (KNCs) have not been raised in sufficient numbers because of their low productivity and undesirable meat characteristics. Usually, native chicken meat in diverse countries has a unique taste and texture that draws the attention of domestic consumers, thereby it leads the price by approximately 2–3 folds that of commercial broilers (Ding et al., 1999). On the other hand, however, meat quality is affected significantly by numerous factors, such as genetics, age, body weight, feed, and other environmental conditions (Chen et al., 2002; Jaturasitha et al., 2008; Moore et al., 2008; Mora et al., 2008). The meat pH, fat, cholesterol, fatty acids, antioxidant dipeptides (carnosine and anserine), and fiber size contribute to the processing functionality and consumer acceptance of meat (Jeon et al., 2010). Owing to critical characteristic for successful product formulation and process control, pH has a great effect on meat quality (Bianchi et al., 2005). However, the pH of meat is regulated by a wide verities of factors, such as genetics (breed lines), gender, the manner of holding animals, transport, lairage conditions and time–pre-slaughter stress, method of slaughter, technological parameters, postmortem handling, and storage time of meat (Ristic and Damme, 2013). Although the genotype has a minor impact on the fatty acid profile of muscle lipids compared to feeding, a difference in growth intensity caused by the genotype could still affect the fatty acids essential to human health (Jaturasitha et al., 2008). The lipid composition of meat is influenced primarily by the birds’ diet, even though other factors, such as age and breed line, also influence the fatty acid profile (Popova et al., 2016). One of the most significant quality indices in chicken meat is the cholesterol content, which needs to be as low as possible (Osada et al., 2006). Poultry strains and ingredients of the feed are the most contributing factors to decreasing the cholesterol level in the meat. In addition, dietary cholesterol is strongly allied with coronary heart disease and arteriosclerosis (Simopoulos, 2004). Recently, many challenges have been implemented to reduce the cholesterol contents in chicken meat by addition with dietary supplements, such as omega-3, garlic, and copper (Chowdhury et al., 2002). However, in Korea, several researchers have conducted experiments on the quality characteristics of KNC meat over the past few years and showed that KNC has nutritional qualities, including a unique flavor and texture (Ali et al., 2019; Jayasena et al., 2013; Jeon et al., 2010).
As endogenous compound, the functional ingredients in chicken meat, such as carnosine, anserine, and functional compounds, has increased considerably with a great interest (Mora et al., 2008; Peiretti et al., 2012; Purchas et al., 2004). A recent study by Ali et al. (2019) compared the carnosine, anserine, and contents of raw meat from three different new native chicken strains in Korea. It has been reported that carnosine (β-alanyl histidine) and anserine (1-methyl carnosine), were assumed a stratagem for poultry production (Schmid, 2009). Anserine is an N-methylated derivative of carnosine, dominated in non-mammalian species, such as poultry and it occupies the similar biological activities to carnosine (pH buffer in muscles, antiglycation, antiaging, antioxidation, and neurotransmitter functions) (Schmid, 2009). Compare with carnosine, anserine is the most ample dipeptide initiated in most types of poultry meat, including KNCs (Ali et al., 2019; Jung et al., 2013).
The muscle fiber number and the cross-sectional area also increased with the body weight and age of poultry (Berri et al., 2007). On the other hand, the chick weight at hatching increases with increasing hen age. The morphology of the muscle arrangements in a state of original or changed after treatments can be related to eating tenderness in meat industry (Jones et al., 1977). Muscle structure differs according to the muscle types, species, and breed of animal or bird, all of which contribute to the metamorphoses in muscle texture.
In Korea, the Ministry of Agriculture, Food and Rural Affairs, and Ministry of Oceans and Fisheries, RDA, has conducted a native chicken breed restoration program through the Golden Seed Project to maintain national resources and consumers’ demand. As a part of this program, a few commercial meat-type native chicken strains were developed. The different quality characteristics were studied recently and compared with those of commercial broilers and a commercial native chickens (Ali et al., 2019). Of them, two new native chicken strains (A and B) were proposed as candidates for selection, considering their nutritional and bioactive attributes under this study. Therefore, a study of the differences in the quality characteristics between white semi-broiler/broiler and two native chicken strains (A, and B) under controlled environmental factors is warranted. Accordingly, this study compared the quality attributes of breast and thigh meat from white semi-broilers (W) and broilers with two new native chicken strains (A and B) raised and slaughtered at similar live weights at two production stages.
Materials and Methods
At an experimental farm of Harim (Gimje, Korea), 200 male chicks from new native chicken strains (A and B) were reared for 5 and 12 wk respectively under similar conditions and diets. Mating combinations from the paternal line and two separate maternal lines were used to produce the new native chicken strains. W was raised for 5 wk and broiler chickens were also raised to the same slaughter weight (about 1.9 kg) as the new native chicken strains at 12 wk, and used as the controls. Newly bred A and B were slaughtered alongside the W and broiler chickens of equal live weight. The carcasses were vacuum-packed and stored at –20°C before the study. After chilling at 4°C for 24 h, the left and right sides of the carcasses’ breast (pectoralis major) and thigh (biceps femoris) muscles were dissected. After sorting the samples, they were minced in a food mixer (Kitchen Aid, 5KPMS, Benton Harbor, MI, USA). The goal supernatant was then rendered from the minced meat samples under various conditions to conduct the study.
Sigma-Aldrich provided the standards (carnosine and anserine) (St. Louis, MO, USA). Cholesterol (Tokyo Chemical Industry, Tokyo, Japan) and 5-cholestane were used as the external and internal criteria (IS) in cholesterol analysis (Sigma-Aldrich, St. Louis, MO, USA).
The pH in the breast and thigh from W, broiler, A, and B treatments were measured using a pH meter (Seven ExcellenceTM, METTLER TOLEDO, Greifensee, Switzerland). For fat contents, the lipids were extracted from 5 g of muscle with chloroform/methanol (2:1), according to the (Folch and Lees, 1951). The carnosine and anserine contents were determined using a slight modification of the method reported by Mora et al. (2007), where 2.5 g of pulverized meat samples from each chicken were homogenized with 7.5 mL of 0.01N HCl at 1,130×g for 30 s (T25 basic, IKA GmbH & Co. KG, Königswinter, Germany). After homogenization, the samples were centrifuged for 30 min at 4°C at 10,000 g (1580 R, GYROZEN, Gimpo, Korea). A 0.5 mL sample of centrifuged supernatant was combined with 1.5 mL of acetonitrile in a 2 mL tube and centrifuged for 10 min at 10,000 g at 4°C (1580 R, GYROZEN). The supernatant was analyzed by high-performance liquid chromatography (HPLC, 1580R, GYROZEN) after being filtered through a 0.2 m membrane filter.
Gas chromatography (GC) was used to assess the cholesterol content of minced samples (HP 5890 Series II, Hewlett Packard, Palo Alto, CA, USA). HP-5 (30 m×0.320 mm, 0.25 μm, Agilent, J & W Scientific, Santa Clara, CA, USA) was used as the analytical panel. The fatty acids composition of the breast and thigh meat was estimated using the method reported by O’fallon et al. (2007) with a minor modification. The assay was carried out using an Agilent 7890 series Gas Chromatograph-Flame Ionization Detector under the following conditions: Injector split mode with a split ratio of 25:1, and temperature 250°C. High purity air, high purity H2, and high purity He were used as carrier gases. The flow rate for H2 and air was 40 mL/min and 400 mL/min, respectively. Each peaks were identified using 37-FAME standard (Agilent), and a fatty acid was represented as a relative proportion of the total identified fatty acids.
The muscle fiber characteristics were determined using the method reported elsewhere (Choi et al., 2012) with slight modifications. Frozen muscles were cut into 10 μm thick transverse sections using a cryomicrotome (CM1860, Leica Biosystems, Washington, DC, USA) at –20°C. Each sample was mounted on 76×26×1 mm adhesive microscope slides (HistoBond®, Paul Marienfeld GmbH & Co. KG, Lauda-Königshofen, Germany), with a coverslip 22×22 mm, and coated with a drop of aqueous mounting medium (S3023, Dako, Carpinteria, CA, USA) with a 22×22 mm coverslip (100 Deckglaser, Menzel-Glaser, inseonmedics, Seoul, Korea). A fluorescent microscope (BX51, Olympus, Tokyo, Japan) with a DP72 digital camera was used to display and photograph all the samples (Olympus). Muscle fiber density (fiber number/ 4,000 μm2) were determined using Photoshop CC (Adobe, San Jose, CA, USA).
FE-SEM was carried out at Cheonnam National University, Yeosu Campus to observe the sarcomere structure and muscle fiber size in the extrudates. The extrudates were split into 1×1×0.2 cm dimensions. For analysis, each test extrudate was cut in both the transverse and longitudinal directions. Double-sided carbon tape was used to adhere the test samples to aluminum stubs. Meat cuts from different treatments were pre-fixed with 2.5% glutaraldehyde for 4 h at 4°C. Subsequently, one percent osmium tetroxide was used to post-fix the samples at 4°C for 2 h (dark condition). The samples were soaked thoroughly in PBS and washed in a gradient of ethanol (30% to 99%), air-drying each wash. For 1 min, a platinum coating was applied at 10 mA. Imaging was carried out using a Sigma 500 FE-SEM (Zeiss, Oberkochen, Germany).
Statistical analysis was conducted using the GLM procedure (SAS, 2003). A significance test between the results was performed using the Student-Newman-Keuls test as a multiple assay technique (p<0.05). The analysis results are presented as the SEM and the least square means.
Results and Discussion
Table 1 lists the pH, fat, and cholesterol content of A, B, W, and/broiler from the breast and thigh meat at 5 and 12 wk respectively. At 5 wk, there were no significant variations in pH, fat, or cholesterol content among the tested groups (W, A, and B) in the breast meat (p>0.05). On the other hand, at 12 wk, the pH and fat content were significantly higher in the broiler than A and B, while the native chicken strains had a significantly higher cholesterol content than the broilers (p<0.05). The lower pH in new native chicken strains (A and B) at 12 wk might be due to the faster energy metabolism, which probably enhances glycogen storage in the muscle (Fernandez et al., 2001). Typically, the composition of the body and muscle changes with the animal’s age; the protein and fat content increase, and the moisture content decreases (Vierck et al., 2020). Generally, the cholesterol content in slow-growing chickens is lower than other meat-type chickens (Ali et al., 2019; Jaturasitha et al., 2008), which does not agree with the present study. The higher cholesterol levels in the new native chickens might be due to the same diet without pasture and the ultimate deposition of additional saturated fatty acids (SFAs) than broilers in the breast at 12 wk (Molee et al., 2012). The cholesterol level, however, varies according to the contents of body fat, skin fat, and bone marrow (Demos and Mandigo, 1995).
In thigh meat, at 5 wk, the new native chicken strains had a significantly higher pH than W (p<0.05), which might be due to lower lactate production with a lower buffering capacity by the native chicken muscle type I in early stage of production that is dominant in thigh meat (Listrat et al., 2016). On the other hand, the pH of broiler meat was significantly higher than that of the new native chicken strains at 12 wk. This may be due to more aggressive behavior by the new native chicken strains, which induces high levels of stress, which, in turn, draws more glycogen into use. As a result, the postmortem glycolysis process is affected significantly, resulting in high lactic acid accumulation and a low pH in the meat (Jaturasitha et al., 2002). Genetics - breed lines, gender, how animals are kept, transport, lairage conditions and time - pre-slaughter tension, method of slaughter, technical parameters and postmortem handling, and meat storage time, all affect the pH of meat (Ristic and Damme, 2013). Furthermore, because the pH of meat varies according to the muscle type of the same animal, it is important to standardize the muscle area where the pH would be measured. This study analyzed the pH, fat, and cholesterol content in the two new lines of native chicken strains (A and B) with slaughter ages of 5 and 12 wk respectively (data are not shown). The pH of the breast meat was affected by age, whereas the fat and cholesterol contents were unaffected by the strain or age. Regarding muscle pH, older birds had a lower pH, which concurs with Glamoclija et al. (2015). On the other hand, the pH and fat content in thigh meat were affected by age. Thus, native chicken strains provide valuable information on the meat quality traits seeking consumer acceptance from the breast and thigh distinctively.
Table 2 lists the fatty acid compositions of A, B, W, and/broiler from the breast and thigh meat at 5 and 12 wk respectively. At 5 wk, the breast meat of the new strains contained a higher content of linoleic acid (C18:2), which is important for a similar flavor to cooked chicken meat flavor (Rikimaru and Takahashi, 2010). White semi-broiler (W) is traditionally used for making the unique cuisine, samgyetang, in Korea (Nam et al., 2010). The new native chicken strains, however, outperformed the broilers in terms of eicosatrienoic acid (C20:3), arachidonic acid (ARA), eicosapentaenoic acid (EPA), docosahexaenoic acid (DHA), and nervonic acid at 12 wk (p<0.05). This result is consistent with the findings reported by Rikimaru and Takahashi (2010), who analyzed the fatty acid compositions of Hinai-jidori chickens and broiler in Japan. Jayasena et al. (2013) reported that KNCs contained high levels of EPA and DHA compared to broilers whose contents resembles ω-3 polyunsaturated fatty acids (PUFAs). On the other hand, the new native chicken strains had significantly higher SFAs and PUFAs contents (p<0.05) than the broilers (Jayasena et al., 2013) at 12 wk. Moreover, the new native chicken strains had a lower ω-6/ω-3 PUFA ratio than the broiler chickens at 12 wk, which is desirable in foods of an animal origin (p<0.05). Regardless of the muscles, at 12 wk, however, the new native strains were enriched with the most favorable fatty acids. Omega-3 fatty acids compared to the broiler (p<0.05) has a key function in the human body. The lower ω-6/ω-3 PUFA ratio of the new native chicken meat was due to the higher total ω-3 PUFAs levels in the new native chicken strains and is associated with the breed or line effects with different genetic makeup (Ali et al., 2021; Enser et al., 2000). Compared to the broilers, the PUFA/SFA (P/S) ratio has a favorable balance between the ω-6 and ω-3 PUFA, and new native chicken strains had significantly higher levels than the broilers in the present study because of the higher PUFAs content. Thus, the breast meat from young new native chicken strain could be replaced with white semi-broiler for samgyetang and old new native chicken strains perform better than broiler because of the ARA, DHA, nervonic acid, and omega-3 fatty acids with a lower ω-6/ω-3 PUFA ratio.
At 5 wk, the thigh meat did not show any significant variations in fatty acid composition among the W, A, and B tested groups (p>0.05. On the other hand, the older (12 wk) native chicken strains had significantly higher levels of ARA, DHA, nervonic acid, and frequently omega-3 fatty acids than the broilers (p<0.05), which is consistent with Rikimaru and Takahashi (2010). New native chicken strains contained a higher total of SFAs than broiler (p<0.05). Nevertheless, compared to broilers, the ω-6/ω-3 PUFA ratio showed a similar trend to breast meat. These results suggest that the ARA content in new native chickens meat appears to be a characteristic feature of meat from new native chicken strains developed recently in Korea. The additional analysis assigned with two new native chickens showed that the age of the birds had greater effects on the fatty acid profile than the line of the birds. On the other hand, the impact of both varied between the breast and thigh meat. The ratio of ω-6/ω-3 PUFA in the breast and thigh was lower in older chickens (p<0.001), which is important for foods and was due to an increase in the ω-3 PUFAs content (Popova et al., 2016). The fatty acid composition could depend on age, line, and interactions within or between the breast and thigh at different slaughter ages (Popova et al., 2016).
Table 3 lists the antioxidant di-peptides in breast and thigh meat from two slaughter ages. Anserine is one of the predominating histidine di-peptides in new native chicken compared to broilers (Ali et al., 2019). The new native chicken strains had higher anserine levels than W or broilers at 5 and 12 wk, respectively (p<0.05), which is in line with Ali et al. (Ali et al., 2019), who compared the three new native chicken strains with broilers as well as commercial native chickens. In breast meat, at both slaughter ages, the carnosine content in W or broiler was significantly higher than in the new native chicken strains, which is in agreement with Jung et al. (2013). The effect of the meat type on the anserine content was the same as the effect of the meat type on the carnosine content. Because anserine acts as a physicochemical buffer against the proton output by anaerobic glycolysis in different muscle traits, the new native chickens had higher anserine levels than the broilers (Dunnett and Harris, 1995). White muscle tissue had a higher carnosine concentration than red muscle tissue, which tends to generate energy-rich phosphate ester under anaerobic conditions (Boldyrev et al., 2004). The anserine and carnosine content in thigh meat showed a similar fashion to breast meat. As a part of additional analysis, the carnosine and anserine contents in the breast of birds were influenced by their age rather than their lines. The carnosine levels were higher in the young birds, while the anserine levels were higher in the older birds (p<0.001). On the other hand, the carnosine levels in the thigh meat were unaffected by age, but the anserine levels increased with age (p<0.001). According to Chan et al. (1994), the muscle anserine content is affected by the breed, muscle type, and animal age.
Table 4 lists the muscle fiber density of the breast meat from W, and broilers with A and B at 5 and 12 wk respectively. Regardless of the slaughter age, A and B had a higher fiber density than the W or broiler. This study shows that a higher muscle fiber density in new native chicken strains was in good agreement with a study reported by Koomkrong et al. (2015) conducted on Thai native chickens and broilers. Different muscle types, ages, breeds, and muscle fiber sizes can influence the number of fibers in meat. Chicken and pigs have experienced improvements in both the number and size of muscle fibers due to selection for an increased growth rate (Chen et al., 2007). The total amount, density, and composition are essential histochemical attributes that influence the fresh or cooked meat during muscle to meat conversion (Joo and Kim, 2011). Intrinsic and extrinsic factors can control muscle fiber biochemical and structural characteristics independently to increase the production efficiency and enhance meat quality (Listrat et al., 2016). The morphological, contractile, and metabolic properties of these muscle fibers define the characteristics of muscle fibers (Joo et al., 2013). Furthermore, the number of muscle fibers is determined genetically before birth, and only the length and cross-sectional area of the muscle fibers increase with age (Wigmore and Stickland, 1983). The influences of the line and age in the two lines of the new native chickens at 5 and 12 wk respectively and were examined further. The findings show that age has a larger effect on the muscle fiber profile than the line (p<0.001). The muscle fiber density of the older native chickens was lower than that of the young birds (p<0.001). Furthermore, regarding the muscle fiber region, the genotype effect interacts with age (Chen et al., 2007). Therefore, breed and age can have distinct and different effects on carcass performance and meat quality in commercial broilers or white semi-broilers and indigenous chickens.
Item | 5 wk | SEM1) | 12 wk | SEM1) | ||||
---|---|---|---|---|---|---|---|---|
W* | A | B | Broiler* | A | B | |||
Muscle fiber density2) | 47.0b | 66.0a | 66.4a | 0.14 | 21.2c | 39.4a | 30.8b | 0.67 |
Figs. 1 and 2 present FE-SEM images of the size and compactness (cross-section) of the muscle structures with the degree of the sarcomere pattern (longitudinal) of the breast meat excised from W and/broilers, A, and B at 5 and 12 wk respectively. Regardless of the slaughter age, the muscle fiber size, which is positively correlated with the carcass weight, was larger in W or broilers than the new native chicken strains; A and B. A previous study showed that the imported breed chickens have a larger fiber size in the breast and thigh muscles than Thai native chickens (Jaturasitha et al., 2008). The larger body weight of chickens is based on the larger muscle fiber diameter and area and lower muscle fiber density (Chen et al., 2007). The new native chicken strains arrange the muscle fiber in a smaller pattern and more compactness, making the muscle tough. A denser muscle fiber was noted in the 5-wk-old birds. These structural differences at different lines and ages might be due to the differences in line, age, rate of rigor onset, and degree of sarcomere shortening (Smith and Fletcher, 1988). The cross-section of a muscle fiber increases more than that of the endomysium and perimysium connective tissue during breast meat production in modern broiler breeds (Dransfield and Sosnicki, 1999). This suggests that the muscles selected for rapid growth have outgrown their life support systems, resulting in muscle injury (Swatland, 1990). Furthermore, with a fixed focus (1 μm), more sarcomere patterns were noted in new native chicken strains than W or broilers for both ages and clearly defined as older birds. On the other hand, the meat tenderization in native chicken strains was attributed to a shorter sarcomere length (Van Laack et al., 2000).
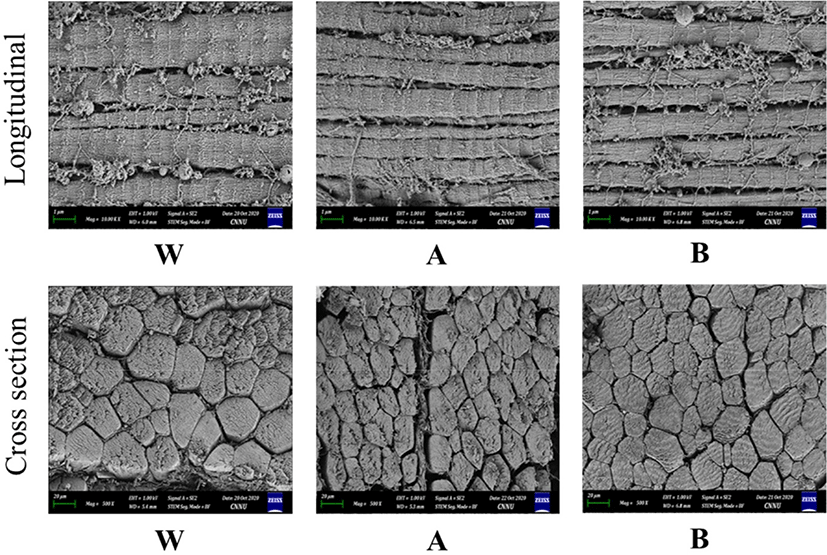
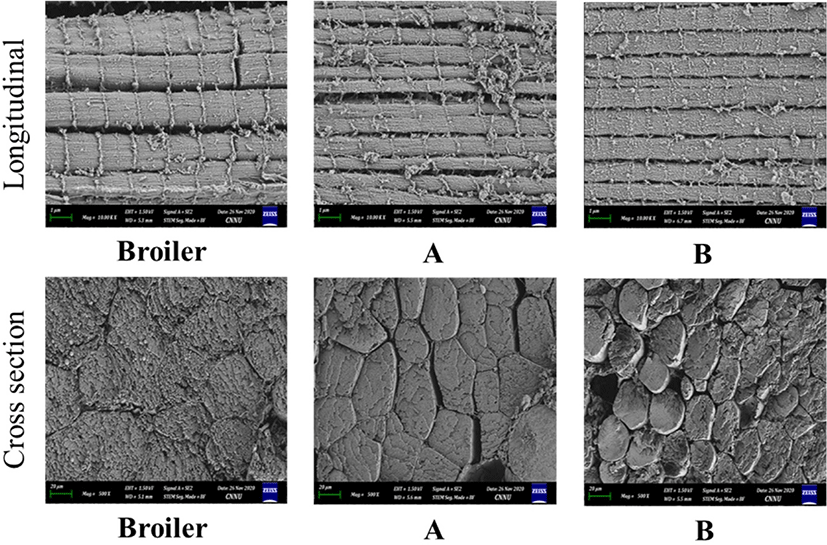
Conclusion
The new native chicken strains (A and B) had distinct quality features compared to white semi-broilers or broilers. The breast and thigh meat showed distinct meat quality traits. On the other hand, the meat from the new native chicken strains had some unique meat quality traits and exhibited more advantages over commercial birds (broilers or white semi-broilers), due primarily to the ARA content, which is related to the palatability of meat. In addition, the new native chicken strains also performed better in terms of omega-3 fatty acids, DHA, nervonic acid, ω-6/ω-3 PUFA ratio, and most abundant antioxidant dipeptide, anserine. Thus, the current investigation provides valuable information for chicken meat from new native strains, which will influence the consumption of different chicken meat with desirable characteristics. Further research will be needed to determine the relationship between those compounds and the palatability of new native chicken strains.