Introduction
Pesticides, consisting of single or multiple components, are used to increase agricultural product yield and quality by preventing and eradicating diseases (Aktar et al., 2009; Eddleston et al., 2008; Yan et al., 2018). Depending on the type of pest, activity site, and purpose, pesticides are classified as fungicides, herbicides, insecticides, or rodenticides. Biological or chemical pesticides affect the growth of living organisms and cause neurological, respiratory, skin, and pathological problems due to adverse effects or unwanted reactions (Azaroff and Neas, 1999; Hoppin et al., 2002). Therefore, safety regulations on the maximum pesticide residue levels in food or feed have been established worldwide (Handford et al., 2015; Jeong et al., 2018).
Fungicides are agents that protect against unwanted diseases caused by fungi. Flutriafol, propiconazole, and tebuconazole are common triazole fungicides used in agricultural products and forage crops (Toda et al., 2021). Tebuconazole [1-(4-chlorophenyl)-4,4-dimethyl-3-(1H-1,2,4-triazol-1-ylmethyl)-3-pentanol] is particularly effective against various foliar diseases in cereals, including wheat, barley, oats, and rye, and is registered for the control of leaf blight, leaf spot, and anthracnose diseases. Tebuconazole is used for the therapeutic and prophylactic control of diseased plants. It is a demethylation inhibitor that affects the cell wall of the fungus by inhibiting spore germination and mold growth, thereby interfering with the production of ergosterol (Duanis‐Assaf et al., 2022; Hu et al., 2019).
Pesticide residues are harmful to the growth and health of animals and plants (Chandra et al., 2021; Damalas and Eleftherohorinos, 2011). Chronic pesticide exposure can cause fibrosis in human and animal tissues (Jeong et al., 2021; Jeong et al., 2022; Park et al., 2021), thereby impairing the functioning of their organs. We assumed that tebuconazole fungicides can cause fibrosis in pigs. However, little is known about the effects of tebuconazole on pig tissues. Therefore, this study investigated the effects of tebuconazole residues on pork and pork products.
Materials and Methods
The pigs were purchased from the Taehung (Iksan, Korea). Twenty castrated male pigs (Landrace×Yorkshire, BW: 63.3±1.3 kg) were housed in separate pens (pen sizes: 2.1 m×1.4 m). The animals were divided into six groups according to the tebuconazole concentrations per BW: control (0 mg/kg bw/d; n=3), T1 (0.25 mg/kg bw/d; n=3), T2 (1.25 mg/kg bw/d; n=3), T3 (2.5 mg/kg bw/d; n=3), T4 (12.5 mg/kg bw/d; n=3), and T5 (25 mg/kg bw/d; n=3). Although some dose such as research papers on the pig residue test were collected, the purpose of this study was to investigate the residual based on the maximum daily intake. Therefore, the treatment levels were determined with the data detected above the quantitation limits (1–3). In pigs, tebuconazole dose selection was based on intake ratio, feed intake amount, dry matter weight, maximum residual amount, and maximum exposure (FAO and WHO, 2010; Li et al., 2017; Schmidt et al., 2016). Housing conditions were maintained as described previously (Jeong et al., 2021). Briefly, the light cycle, relative humidity, and temperature during the experimental period were 12 h, 55±5%, and 22±2°C, respectively. Diet and water were provided ad libitum, according to the Korean feeding standards for pigs. The animals were exposed to tebuconazole twice daily (09:00–10:00 am, 15:00–16:00 pm) for 4 wks. Blood samples were collected at 14 d and 28 d after tebuconazole exposure. After anesthesia and exsanguination, the pig tissues were quickly removed and immediately frozen in liquid nitrogen. The tissues were fixed in 10% neutral buffered formalin (NBF; Sigma-Aldrich) for histological analysis.
Blood samples were obtained from the jugular vein using a Vacutainer tube. The serum was centrifuged at 700×g for 20 min at 4°C and stored at −80°C. Total parameters, such as glucose (GLU), creatinine (CREA), blood urea nitrogen (BUN), blood urea nitrogen/creatinine ratio (BUN/CREA), phosphate (PHOS), calcium (CA), total protein (TP), albumin (ALB), globulin (GLOB), albumin/globulin ratio (ALB/GLOB), alanine aminotransferase (ALT), alkaline phosphatase (ALKP), gamma-glutamyl transpeptidase (GGT), total bilirubin (TBIL), cholesterol (CHOL), amylase (AMYL), and lipase (LIPA), were assessed according to the manufacturer’s protocol using a VetTest chemistry analyzer (IDEXX, Westbrook, ME, USA).
Tissue (2 g) and blood (2 mL) were mixed with distilled water (10 mL) for 10 min in a mixture of acetonitrile (20 mL) and sodium chloride (5 g) to quantify tebuconazole. The mixed samples were stirred for 10 s and shaken for 60 min. The tissue and blood extracts were centrifuged at 2,200×g for 5 min. The untreated and tebuconazole-treated samples (5 μL) were injected into a liquid chromatography-tandem mass spectrometer (QTRAP 4500 LC-MS/MS system, SCIEX, Framingham, MA, USA). After injection, the tebuconazole residue levels were estimated using the peak area. The limit of quantification was below 0.02 mg/kg. The conditions used were as described previously (Jeong et al., 2021).
The tissue samples fixed with 10% NBF were sequentially dehydrated using ethanol and xylene as follows: The tissues were embedded in paraffin, cut at 5 μm thickness, mounted, and placed on a hot plate at 40°C for 1 h. The slide sections were dewaxed with xylene, rehydrated with EtOH, and rinsed in tap water for 1 min. Histological connective tissues were stained using a trichrome stain kit (ab150686, Abcam, Cambridge, UK), according to the manufacturer’s instructions. The slices were observed using a microscope (under 200× magnification).
Results and Discussion
The final BW, average daily feed intake, and average daily weight gain were not significantly different between the control and treatment groups (Table 1). Thus, tebuconazole treatment at different concentrations for 28 d did not affect the growth performance of pigs. These results are similar to those of other previous studies (Jeong et al., 2021; Jeong et al., 2022; Lopez-Antia et al., 2021). Propiconazole and flutriafol were not significantly different between control and the treatment groups in pigs and chicks. However, in rats treated with similar concentrations of tebaconazole, the physical condition deteriorated and the growth ratio decreased (Ben Saad et al., 2020), whereas the liver weight and liver/body weight ratio increased (Ku et al., 2021).
Effect of tebuconazole exposure on the biochemical properties of pig serum were analyzed at 14 d (Table 2) and 28 d (Table 3). After treatment for 14 d, BUN, GGT, and CHOL levels were higher in the high-dose group than in the control group (p<0.05). However, ALKP levels were significantly lower in the T2 treatment group (p<0.05). After treatment for 28 d, most biochemical parameters, except ALT, were not significantly different among the groups. ALT levels were significantly different in the tebuconazole-treated pigs (p<0.05). The principal component analysis plot showed a difference between the samples collected at 14 d and 28 d (Fig. 1A). The scores for BUN/CREA ratio (VIPs=1.98), BUN (VIPs=1.85), GLU (VIPs=1.79), and GGT (VIPs=1.67) levels were high when separating groups based on the variable importance plots calculated by partial least squares discriminant analysis (Fig. 1B).
GLU, glucose; CREA, creatinine; BUN, blood urea nitrogen; PHOS, phosphate; CA, calcium; TP, total protein (TP=ALB+GLOB); ALB, albumin; GLOB, globulin; ALT, alanine aminotransferase; ALKP, alkalinephosphatase; GGT, gamma glutamyl transpeptidase; TBIL, total bilirubin; CHOL, cholesterol; AMYL, amylase; LIPA, lipase.
GLU, glucose; CREA, creatinine; BUN, blood urea nitrogen; PHOS, phosphate; CA, calcium; TP, total protein (TP=ALB+GLOB); ALB, albumin; GLOB, globulin; ALT, alanine aminotransferase; ALKP, alkalinephosphatase; GGT, gamma glutamyl transpeptidase; TBIL, total bilirubin; CHOL, cholesterol; AMYL, amylase; LIPA, lipase.
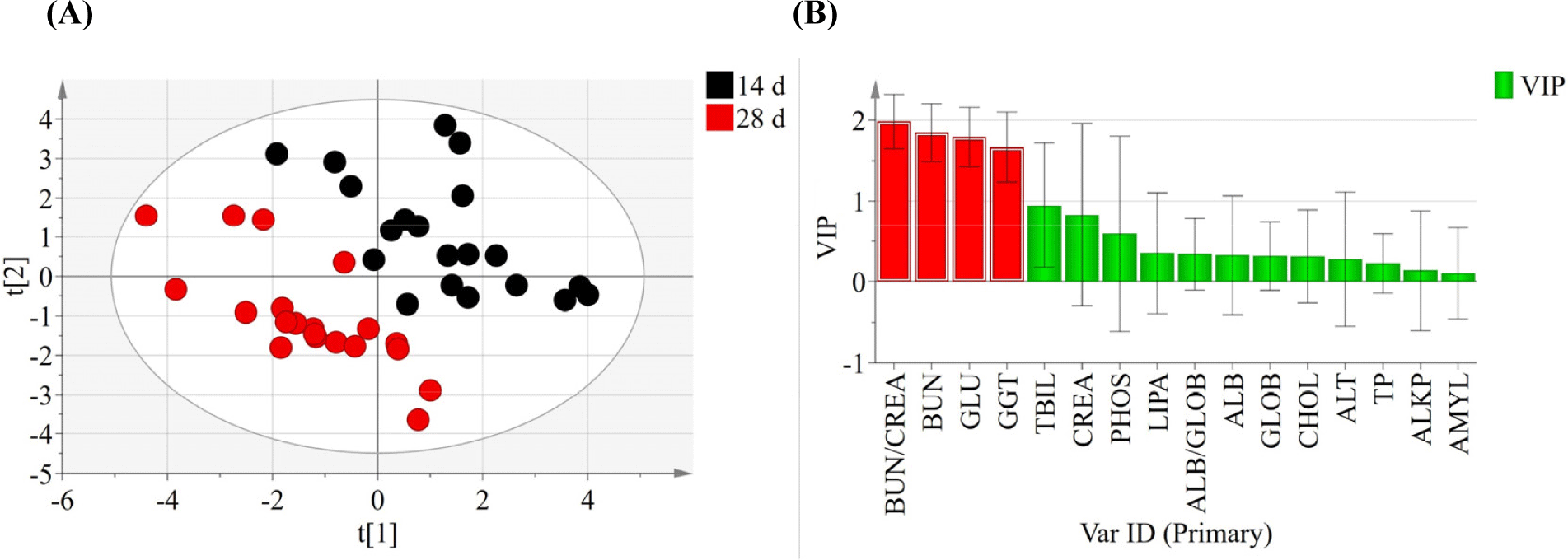
Blood provides useful information regarding the dysfunction and immune disorders in animal tissues. Biochemical changes provide critical information on animal physiology. In this study, pigs treated with tebuconazole showed significantly different BUN, ALKP, GGT, CHOL, and ALT levels at 14 d and 28 d. Previous studies have also reported that pesticide exposure increases BUN and CHOL levels (Amin and Hashem, 2012; Haghighizadeh et al., 2015; Ritu et al., 2013). Abnormal blood BUN levels cause glomerular disease, tissue breakdown, and urinary excretion in the kidneys. Urea produced by ammonia in the liver is a potential blood biomarker for kidney damage (Singh et al., 2011). CHOL is a major component of cell membranes and a precursor of stress hormones. They are involved in detoxification processes and maintain homeostasis under stress responses (Firat et al., 2011; Wang et al., 2022). GGT is ubiquitously found in unicellular and multicellular eukaryotes (Rawlings et al., 2010; Saini et al., 2021). GGT has been recognized as a marker for physiological diseases such as biliary hyperplasia and necrosis (Divers, 2015; Reuter et al., 2009). ALT regulates transamination and is a marker for functional damage in the liver and kidney (Firat et al., 2011; Ochiai et al., 2020; Sookoian and Pirola, 2012). Previous studies have also shown that triazole fungicides (i.e., propiconazole and flutriafol) increased the ALT levels in pigs (Jeong et al., 2021; Jeong et al., 2022). ALT levels increase ROS levels and oxidative damage (Patil et al., 2009; Singh et al., 2011). For example, insecticide and acaricide using dimethoate showed statistically significant serum levels of enzymes, including ALT, aspartate aminotransferase, and ALKP, when compared to control animals (Al-Awthan et al., 2014).
ALKP consists of isoenzyme groups and is a hydrolase of PHOS groups (Sharma et al., 2014). Total tract digestibility of in vitro caused by multi-enzyme addition (Recharla et al., 2019). Increased ALKP activity following pesticide exposure, is often an indicator of hepatobiliary changes in dogs. The ALKP value had no relationship with type and was not a marker of hepatotoxicity (Yokoyama et al., 2019). Although ALT, GGT, and ALKP levels were increased by copper-based pesticides (Arnal et al., 2011), organophosphorus pesticides containing propiconazole decreased ALKP levels in pigs (Jeong et al., 2021).
Thus, blood biochemical analysis offers critical information about the internal environment of organisms (Fırat et al., 2011). Based on this study, BUN/CREA ratio, BUN, GLU, and GGT are proposed as potential biomarkers for identifying tebuconazole exposure compared with the non-exposure group.
Linear and quadratic equations were used to determine the residual limits of dietary tebuconazole in pig tissues (Fig. 2). The quadratic equations for fat (r2=0.9862) and liver (r2=0.9976) showed significant differences among exposure concentrations. The linear equations for fat (r2=0.9807) and liver (r2=0.9813) were also determined. The residual tebuconazole levels increased in the liver and fat tissues. No residual tebuconazole was detected in the blood, kidney, and muscle.
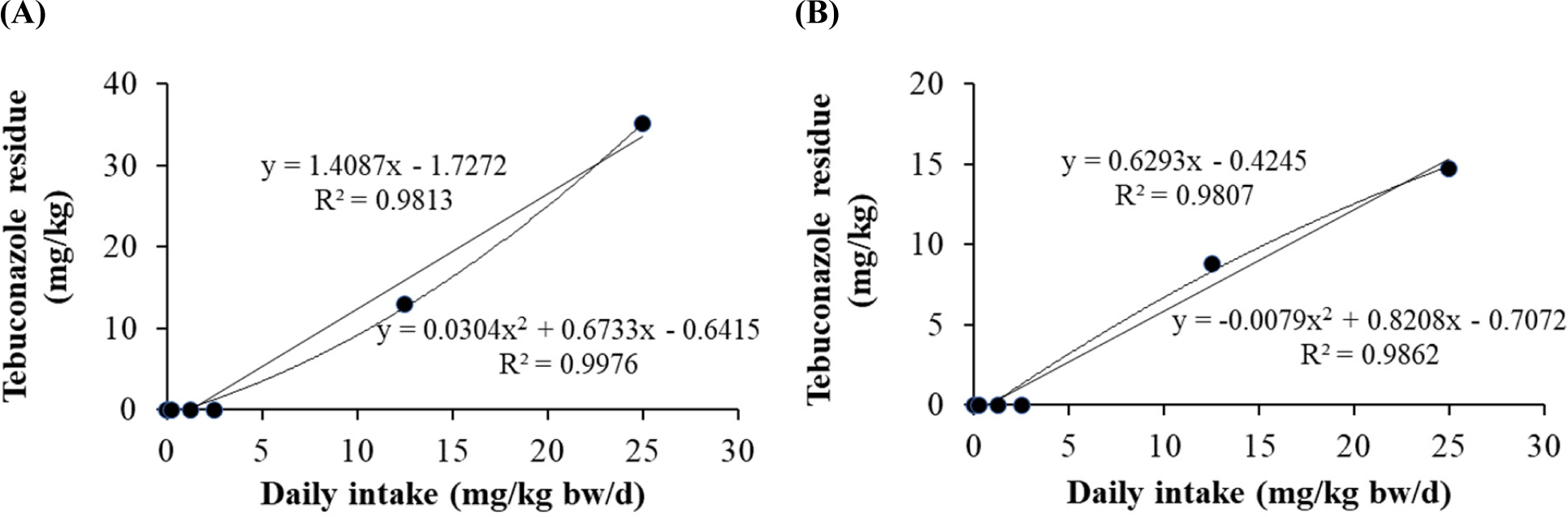
Our previous studies based on equation models suggested that triazole fungicides (propiconazole and flutriafol) showed different residual levels (Jeong et al., 2021; Jeong et al., 2022). In this study, despite acute exposures at high concentrations, the reason for no residual amount in blood, kidney, and muscle tissue is assumed to be the residual characteristics depending on individual tissues because they are excreted. Therefore, the residual values in pig tissues differed based on pesticide type. Together, prediction equations for the residual levels provide critical information for the safety regulation system.
Histological changes in tissue sections of fat, ileum, kidney, liver, and muscle exposed to tebuconazole were examined to distinguish collagen fibers by Masson’s trichrome staining (Fig. 3). Partial fibrotic changes were observed in the tebuconazole- exposed groups. In the control group, the morphological changes of the five tissues showed a normal structure and a small amount of blue staining. The hepatic portal areas, including the bile duct, branch of the hepatic artery, and portal vein, were stained, and the lobular boundary of the liver was more stained (blue) in the treatment groups than in the non-exposed group.
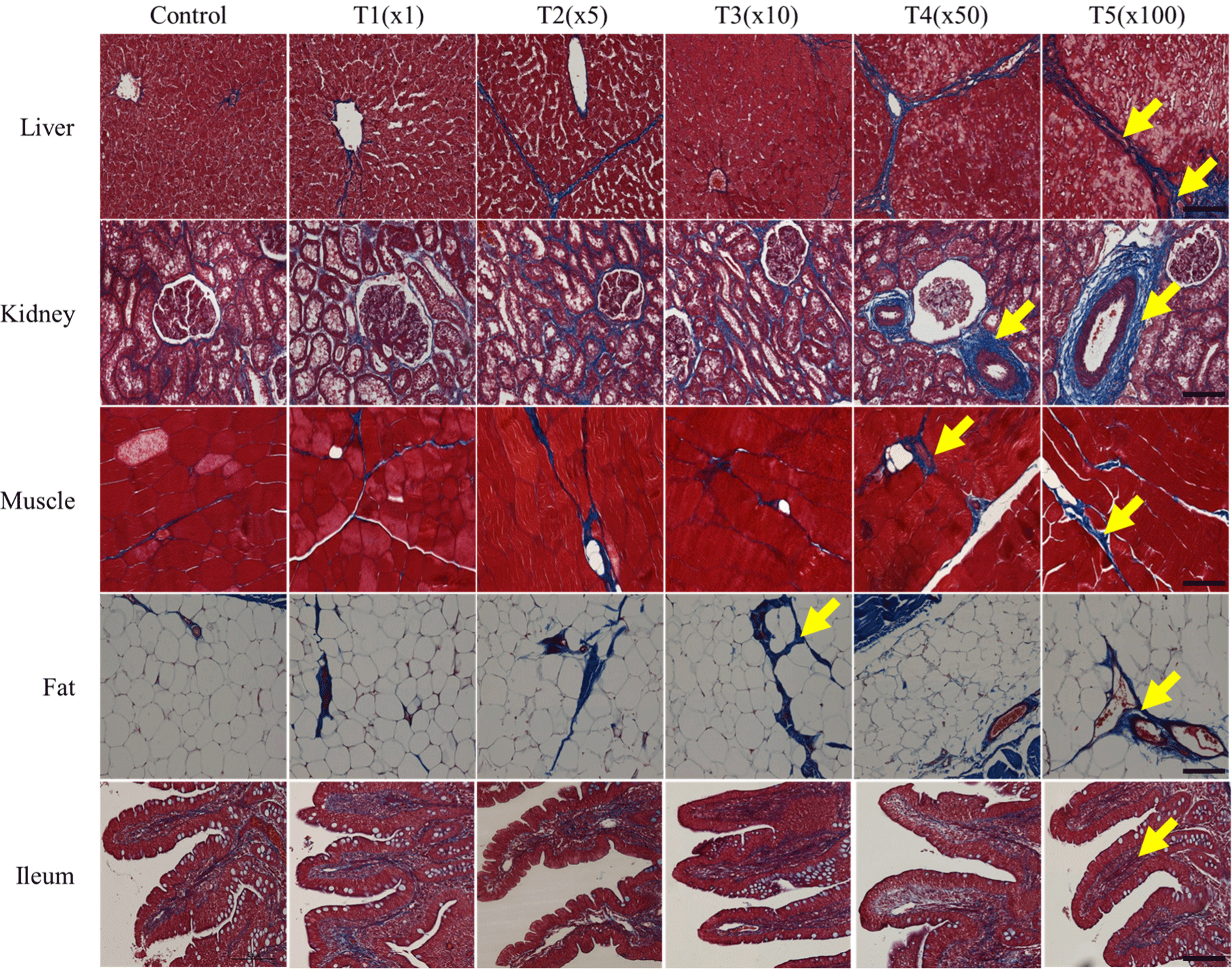
Generally, tebuconazole exposure induces collagen fiber formation. Likewise, in this study, tebuconazole exposure caused morphological changes in healthy kidney tissues, including glomeruli, tubules, and vessels. In particular, tebuconazole affected the fibrotic model, resulting in glomerulosclerosis and arteriosclerosis at different concentrations. Similarly, compared with the control, the connective tissues and veins in the fat tissues were expanded in the treatment groups. However, tebuconazole exposure did not dramatically affect ileum and muscle tissue. Thus, tebuconazole exposure causes collagen fibrosis in the liver, kidneys, and fat tissues of pigs.
The kidney is structurally and functionally composed of two parts: The glomerulus and the tubule. The glomerulus is a capillary circuit through which primary urine is filtered from blood (Arif and Nihalani, 2013). The tubules through which the primary urine flows are responsible for specific functions in reabsorption, secretion, water processing, and metabolism (Ferenbach and Bonventre, 2016). Many kidney diseases affect specific cell types or compartments of the kidneys. As pesticides are primarily excreted by the kidneys, fibrosis occurs around the tubules.
Hepatic fibrosis is induced by the excessive accumulation of collagen, which is characteristic of most types of chronic liver diseases. Chronic liver damage due to advanced liver fibrosis can lead to cirrhosis and portal hypertension and often requires liver transplantation (Bataller and Brenner, 2005). Liver and kidney fibrosis expand in vacuoles and proximal tubules, causing necrosis and hepatocellular damage. In this study, these histological changes were observed in the liver, kidneys, and fat tissues after tebuconazole treatment. Thus, tebuconazole induces the deterioration and degeneration of tissues.
Conclusion
In conclusion, our findings indicate that tebuconazole exposure affects the morphology of the pig liver, kidney, and fat, which causes fibrosis. After tebuconazole treatment, BUN, GGT, CHOL, and ALKP levels at 14 d and ALT levels at 28 d in the treatment groups showed significant differences compared with the control. In particular, the BUN/CREA ratio, BUN, GLU, and GGT are potential biomarkers for tebuconazole at 14 d and 28 d. The equations for tebuconazole residues in liver and fat tissues showed that pesticide was detected at more than 50 times the acceptable daily intake. Our study showed that the residual levels of tebuconazole in each tissue are different. Therefore, even though tebuconazole is not detected, pesticide cause physiological changes and provide information for establishing maximum residue limits in pork and pork products.