Introduction
Mutton is gradually becoming a superior substitute for the domestic market, as it is a good source of essential nutrients, high in protein and low in fat (Ramos et al., 2021). In the last few years, the consumption of high quality mutton products has risen dramatically. Chaka sheep as Qinghai Plateau wool and meat with semi-fine wool sheep is a breed of Tibetan sheep, which grows in the area of Chaka Salt Lake in Wulan County, is a cultivar of Chaka sheep (Chen et al., 2019a). In 1971, Wulan County introduced the British and New Zealand Romney-Marsh long-wool type of breeding sheep from Hudong Original Breeding Field of Qinghai Province and Daliuliu Original Breeding Field of Anhui Province to be cross-bred (Chen et al., 2019b). As the breed was improved and bred in the Chaka area, it was named “Chaka sheep”, which has been granted the protection of the Registration of Geographical Indications for agricultural products (Guo et al., 2022). Studies have shown that there are some differences in the nutritional composition, sensory quality, and processing quality of different parts of the muscle (Mohanty et al., 2010). Different parts of muscle have unique collagen content, structure, and properties (Zuo et al., 2016). Kohn et al. (2023) found significant differences in muscle fiber types and metabolic profiles in different parts of African black ostrich muscles.
Lipids, an important nutritional component in lamb, are critical biological small molecules that act as components of cell membranes, signal transduction, energy sources, and signaling pathway intermediates (Lugo Charriez et al., 2021). The lipid profile of meat is influenced by many factors, one of which is the type of muscle (Boselli et al., 2008). The lipid composition of tissues varies in different parts of the body (Yin et al., 2023). Li et al. (2022a) identified seventy-nine of 842 lipids in different muscles of Hu lambs. The content and type of lipids vary significantly in different meat products, and potential markers of lipids have gradually become one of the methods to analyze the quality and type of meat products. However, studies on the lipid profile and characteristics of different parts of the muscles of Chaka sheep are still incomplete. Thus, the lipidomic profile and its components of Chaka sheep need to be elucidated.
Therefore, this study determined and analyzed the nutrient composition, lipid content, and differential lipid molecules in the longissimus dorsi (LD), psoas major (PM), and biceps femoris (BF) of the Chaka sheep (Fig. 1). The aims of this research are to define the basic data about the composition and nutritional quality of three different muscles of the Chaka sheep and to provide insight into the utilization of lipidomics in the animal products industry.
Materials and Methods
The samples were obtained from Jintai Ranch, Chaka Town, Wulan County, Haixi Mongolian and Tibetan Autonomous Prefecture, Qinghai Province, China. We selected nine experimental sheep, all 1 year old, weighing about 45 kg, female. They were identified by experts as Chaka sheep. The Chaka sheep were manually slaughtered by puncturing the occipital atlantoaxial joint of the sheep with a sharp knife, severing the spinal nerves, then cutting the carotid artery to bleed out the blood, and then skinning the sheep, and after removing the head and hooves, 9 replicates each of LD (muscles of the back of the sheep between the 9th and 11th ribs), BF, and PM were collected. The LD, PM and BF of Chaka sheep were cut and packed into lyophilized vessels and then immediately stored in liquid nitrogen for lipidomic study, and the remaining meat samples were wrapped in cling film and placed in a thermal box and returned to the laboratory for nutrient analysis. Three replicates were set up for nutritional quality determination and six replicates for lipidomics.
An OPTO-LAB meat color analyzer (Konica Minolta, Tokyo, Japan) was employed to measure the color of the muscle. A PH-STAR carcass muscle pH direct tester (MATTHAUS, Portmeuse, Germany) was utilized for pH measurement of all samples. Crude protein (CP), total ash (Ash), ether extract (EE), and moisture content (MSTR) were measured according to Cohen’s methods (Cohen, 1971).
Conventional hematoxylin and eosin (H&E) staining was used to measure muscle fiber characteristics. Samples were scanned using a Leica RM-235 slide scanner (Leica, Nussloch, Germany) and the images were acquired by using CaseViewer 6.0 software (3D-Histech, Budapest, Hungary; Xu et al., 2023).
A Sykam S-433D amino acid analyzer (Sykam, Munich, Germany) was used for amino acid determination. After weighing 50 mg of dry meat powder from a single sample and hydrolysis with hydrochloric acid solution (36% hydrochloric acid: water=1:1, v/v) for 24 h, 2 mL of the diluted specimens were transferred to the vial condenser. 10 mL of sample diluents were poured in and stirred. The compounded sample was withdrawn with a hypodermic syringe, filtered using a needle filter, and injected into the vial to assay (Ma et al., 2021).
The fatty acids were determined by gas chromatography-mass spectrometry (GC-MS). 5 g of the sample was taken, drawn and filtered into the injection vial with a stirred syringe. After adding 8 mL of a sodium hydroxide-methanol solution at 20 g/L relative density, a condensor for fat degradation and fatty acid methyl esterification was attached, n-heptane was poured in, shaken for 2.5 min, and a sodium chloride aqueous buffer solution was poured in. Supernatant was collected, a volume of sodium sulfate hydroxide was added, stirred thoroughly, and supernatant was transferred to vials for automated analysis (Schiavon et al., 2016).
The combined technology of solid phase microextraction (SPME) and GC-MS was applied to determine the volatile aroma compounds. The muscle was minced and ground, 4 g meat was added to a 20 mL headspace bottle, 20% NaCl solution and 5 μL of 2-methyl-3-heptanone solution was mixed, stirred thoroughly, and water bath (85°C) for 55 min, then chilled to 55°C. The SPME fiber probe was inserted into the GC-MS injector connection, and the adsorber head was in place for extraction for 40 min. The SPME fiber probe was inserted into the GC-MS injector connection, and the adsorber head was in place during extraction for 40 min, and the fiber was withdrawn for desorbing into the GC-MS insertion port for 3 min by GC-MS. The chromatographic parameters were as follows: the carrier gas was helium, the flow rate was 1.0 mL/min, the injection port temperature was 250°C, the column temperature was started at 40°C, maintained for 5 min, and then increased to 230°C (5°C/min) for 8 min. The mass spectrometry conditions were as follows: the ion source temperature was 200°C, the ionization mode was EI, the electron energy was 70 eV, and the scanning mass range was 35–500 amu (He et al., 2020).
The samples were spiked with the appropriate amount of internal lipid standard, and then homogenized with 200 μL water and 240 μL methanol. Then 800 μL of methyl t-butyl ether was added to the mixture. The mixture was sonicated for 20 min at 55°C and then allowed to stand for 30 min at room temperature. The upper layer of organic solvent was removed by centrifugation at 14,000×g for 15 min. The samples were dried under nitrogen.
A CSH C18 column (1.7 μm, 2.1 mm×100 mm, Waters, Milford, MA, USA) was applied for LC separation. Lipid extracts were dissolved in 200 μL of 90% isopropanol-acetonitrile and incubated at 14,000 g for 15 min. Finally, 3 μL of the specimen was injected. The first mobile phase was 40% acetonitrile-isopropanol (1:9, v/v) at a flow rate of 300 μL per min. This was maintained for 3.5 min, followed by a linear increase to 75% acetonitrile-isopropanol (1:9, v/v) in 9 min. A linear increase to 99% acetonitrile-isopropanol for 6 min and then equilibration at 40% acetonitrile-isopropanol for 5 min.
The mass spectra were collected by the Q-Exactive Plus in the positive mode and in the negative mode, respectively. The ESI parameters were adjusted and optimized for all experiments. The experimental parameters were as follows: the source temperature was 300°C, the capillary temperature was 350°C, the ion spray voltage was 3,000 V, and the instrument scan range was 200–1,800 m/z (Tang et al., 2023).
Results of the study were presented as mean±SD in this assay with three replicates for each sample. SPSS 22.0 (IBM, Armonk, NY, USA) was used to analyze the regular nutritional composition data statistically. Orthogonal partial least squares discriminant analysis (OPLS-DA), variable importance in projection (VIP)>1, p-value<0.05 was defined as the criterion for significant differences.
Results and Discussion
There was no significant difference in the results of routine nutrient content measurements (Table 1) at pH45min, moisture, ash, and EE, but the CIE a*, CIE b*, and CIE L* of PM were significantly higher than LD and BF (p<0.05). The CIE a* is associated with meat hemoglobin content, CIE b* is negatively correlated with muscle freshness, and CIE L* is related to muscle whiteness (Liang et al., 2023). Therefore, we hypothesized that PM has the highest hemoglobin content and LD has the best freshness, which may be more popular with consumers in terms of meat color. The rank order of CP levels in three parts of Chaka sheep was: LD>BF>PM.
Previous studies showed that muscle fiber properties, such as type, size and total number of fibers in different parts were different, the higher muscle fiber density, the higher economic value of meat (Qin et al., 2020). The results of myofiber measurements are shown in Fig. 2, the total area of myofibers did not differ significantly between LD, PM, and BF, the total number of myofibers was ranked from most to least: PM>LD>BF, the density of myofibers was ranked: PM>LD>BF, the diameter of myofibers was the largest in BF. Our study demonstrated significant differences in total myofiber number, myofiber density, and myofiber diameter in LD, PM, and BF (p<0.05). The myofiber density in LD (1,557.93 n/mm2) and PM (1,588.74 n/mm2) were significantly higher than in BF (781.11 n/mm2), so it may suggested that LD and PM have a higher commercial value than BF.
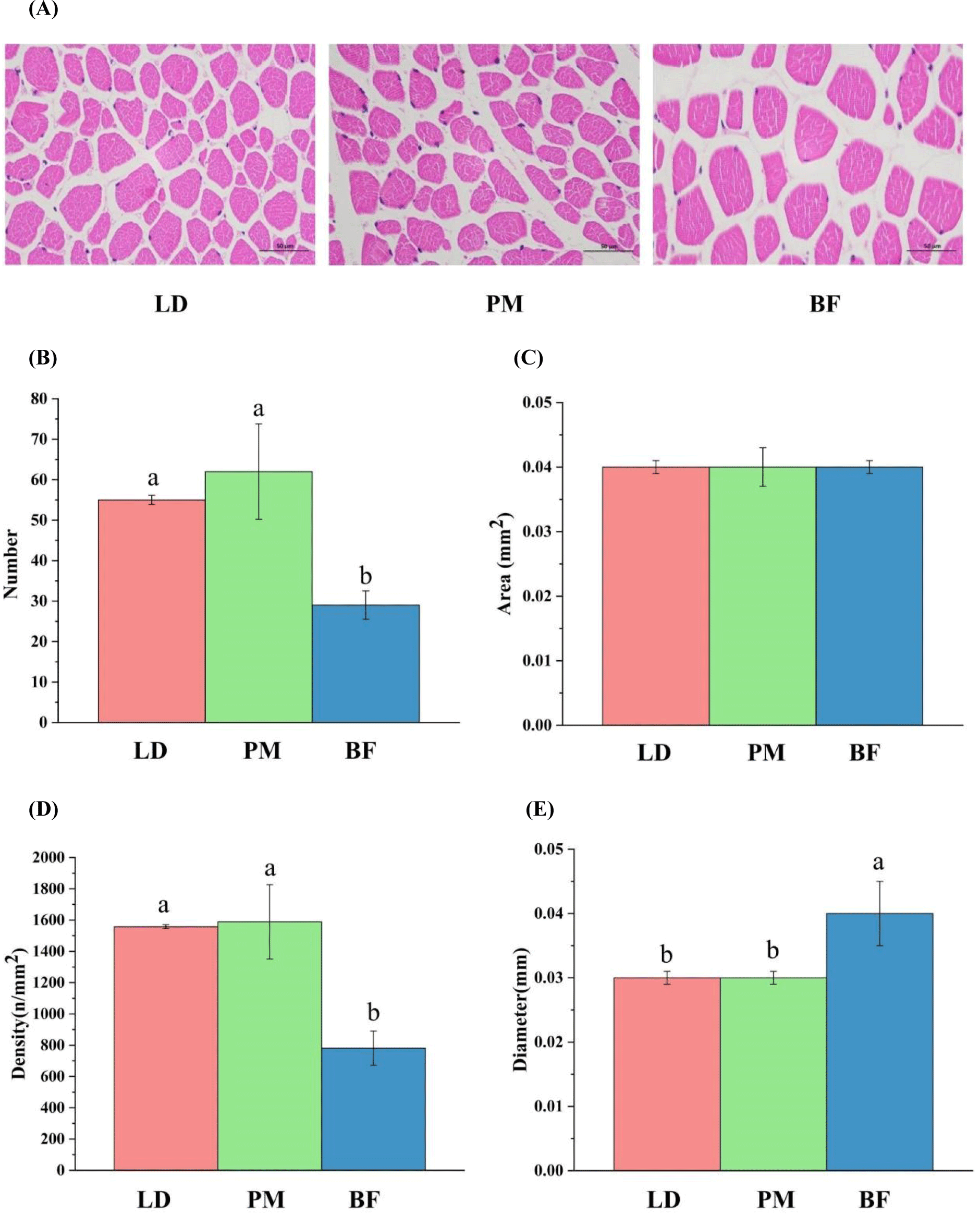
The results of amino acid measurement are presented in Table 2, the total amino acid and essential amino acid levels in different parts of Chaka sheep were not significant, but our previous study has indicated that there were differences in CP content. This inconsistency may be caused by calculating CP based on nitrogen content. However, in addition to protein, meat also includes other nitrogenous compounds such as phospholipids (PLs; Gao et al., 2022), so the level of CP was not exactly equal to TAA. There were significant differences in the levels of aspartic acid, glycine, histidine, and proline in different parts of the muscle. The contents of aspartic acid and histidine were the highest in LD, followed by BF, and the lowest in PM. The contents of glycine and proline were in the following order high to low: BF>LD>PM. Studies have indicated that the free precursor amino acids affect the flavor of the meat, which is essential in evaluating the quality of the meat (Tian et al., 2021), glycine often imparts a sweet flavor to mutton, aspartate gives the meat and umami taste, and favorited by consumers (Ribeiro de Araújo Cordeiro et al., 2020). Histidine is essential for producing histamine, a chemical messenger essential for immune reactions, digestion, and sleep/wake cycles (Zhang et al., 2022a). LD had the highest levels of aspartic acid and histidine, and BF had the highest levels of total glycine and proline, from which we hypothesized that the flavors of LD and BF might be more likely to be preferred by consumers.
Fatty acids are closely related to the eating quality of meat by determining the melting point and quality of meat fats (Dai et al., 2024). The contents of fatty acids in different parts of the muscle are shown in Table 3. A study has shown that intramuscular fat content was positively correlated with SFA (Zhang et al., 2019), which was consistent with the composition of crude fat in PM, LD, and BF in our findings. The content of linoleic acid (C18:2n6c) was, in decreasing order, ranked as follows: PM>BF>LD. Haag’s study found that eicosapentaenoic acid (EPA) and docosahexaenoic acid (DHA) are metabolic byproducts of linoleic acid and linolenic acid, which are vital to the human body in the maintenance of cardiovascular health, brain function, and the reduction of pain in rheumatoid arthritis (Haag, 2003; Oh, 2005). Therefore, we hypothesized that the higher linoleic acid in PM would favor the production and accumulation of EPA and DHA. The total of ω-6 polyunsaturated fatty acid (PUFA) was ranked as: PM>BF>LD. Studies have found that ω-6 PUFA cannot be produced by the body, but must be consumed in the diet, and meat is an essential source of ω-6 PUFA. It is recommended that people should get these nutrients through a balanced diet to maintain the body’s ω-6 PUFA level (Simopoulos, 2008). Overall, our results indicated that there were differences in the fatty acid content of muscles from Chaka sheep, the PM exhibited the highest nutritional value in the fatty acids profile.
As presented in Table 4, aldehyde, alcoholic, ketonic, carboxylic, esteric, and other volatile aroma compounds have been identified in Chaka sheep muscle. There were marked differences in the alcohols, ketones, and esters of the Chaka sheep, with PM having the highest levels of total alcohols, ketones, and esters. Alcohols are derived from the breakdown of lipids and have a high olfactory threshold and a generally clear odor similar to that found in fruits and vegetables. Ketones, a category of volatile organic compounds with floral, fruity, and creamy aromas, have an impact on the overall flavor of meat products (Ge et al., 2023). Ester compounds, most of which have an aromatic flavor, are formed by the interesterification of acids and alcohols (Yang et al., 2024). We found that the PM exhibited the highest nutritional value in the fatty acids profile, so we speculated that the higher fatty acid oxidation may lead to the accumulation of flavor substances in PM.
Lipids play an essential role in the production of the odor and the flavor of meat via autoxidation or thermal oxidation (Li et al., 2021). A detailed classification of the categories is crucial for the analysis of the metabolic pathways that underlie the transformation of lipids (Enser et al., 1999). A total of 2,639 lipid molecule species were detected in the Chaka sheep. The lipids were composed of 42 categories (Fig. 3), and the percentages of content, in descending order, were: triacylglycerol (TG: 18.91%), phosphatidylcholine (PC: 18.34%), phosphatidylethanolamine (PE: 14.25%), cardiolipid (CL: 6.14%), diacylglycerol (DG: 5.99%), monosaccharide ceramide (Hex1Cer: 5.53%), phosphatidylserine (PS: 4.96%), sphingomyelin (SM: 3.68%), ceramide (Cer: 3.64%) and others. Intramuscular fat is composed of PLs, TGs, and cholesterol, and the balance between triglyceride synthesis, breakdown, and absorption is reflected in IMF content (Roy et al., 2024). The effect of the lipid content on aroma retention is greater than the influence of the lipid type (Ayed et al., 2018). TGs and PLs have been shown to be the predominant lipids for predominant lipids for binding and generating flavor compounds (Fu et al., 2022). Liu’s study suggested that the highest types and concentrations of TG may contribute primarily to aroma retention in roasted mutton (Liu et al., 2022). Therefore, our hypothesis was that the high TG levels in Chaka sheep might be correlated with the taste of the meat. PC, PE, and CL being the major components of PLs. Zhou’s study found that the different PL forms (PC and PE) not only can regulate membrane fluidity and permeability but also are important components of PLs (Zhou et al., 2014). PLs, which accounted for almost half of the total number of molecules of lipid classes, were predominant in these three samples in our study. A study showed that PLs are abundant in PUFAs and are an essential resource of PUFAs (Zhou et al., 2013), and the flavor of meat is associated with the composition of PLs (Wu and Wang, 2019). Wang et al. (2009) found that the PLs were the main factor in forming the flavor of duck meat products, which also was one of the major determinants of the taste of duck meat products. Therefore, we hypothesized that the flavor of Chaka sheep would be associated with the levels of PLs.
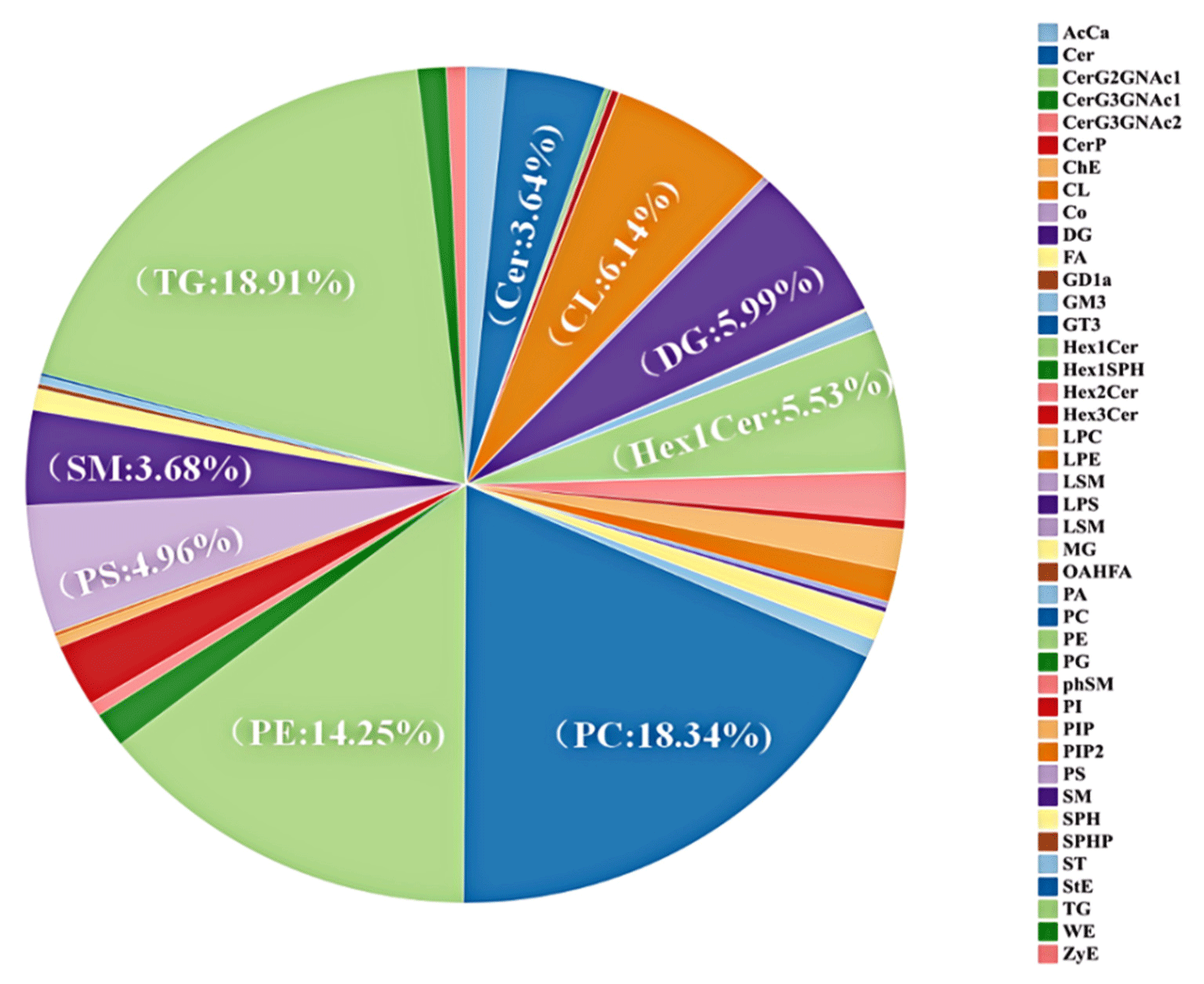
The total number of lipid molecules in the different parts of the Chaka sheep is summarized in Fig. 4. The comparative results were as follows: PM>BF>LD. The difference between the groups was not significant. The composition of lipid subclasses in different parts of the Chaka sheep is shown in Fig. 5. PC, PE, phosphatidylinositol (PI), PS, sphingosine (SPH), TG, and WE were major subclasses lipids in Chaka, and all of them were present at levels >1,000 μg/g. TG levels varied significantly among the three comparison groups, with the highest levels in the BF group, followed by the PM group, and the lowest levels in the LD group (p<0.05). PC, PE, PI, PS, and SPH were belong to PLs. PC and PE are the major PL classes in porcine intramuscular fat, and PEs are the predominant glycerophospholipid in ruminant cell membranes (Fernandez et al., 1999). In conclusion, PLs were the highest total percentage of Chaka sheep, and PLs are also essential constituents of cell membranes. Hazel’s study indicated that long-chain PUFA is more highly mobilized at low temperatures, and the higher the PL content, the higher the long-chain PUFA content, which may correlate with better plateau adaptation in yak (Hazel and Williams, 1990). Lin’s study showed that fish muscle PLs were sensitive to decreasing temperature (Lin et al., 2019). Therefore, we hypothesized that the higher levels of PLs molecules in the muscles of Chaka sheep would facilitate their adaptation to the extreme environment of the Tibetan Plateau, which is characterized by high levels of cold and hypoxia.
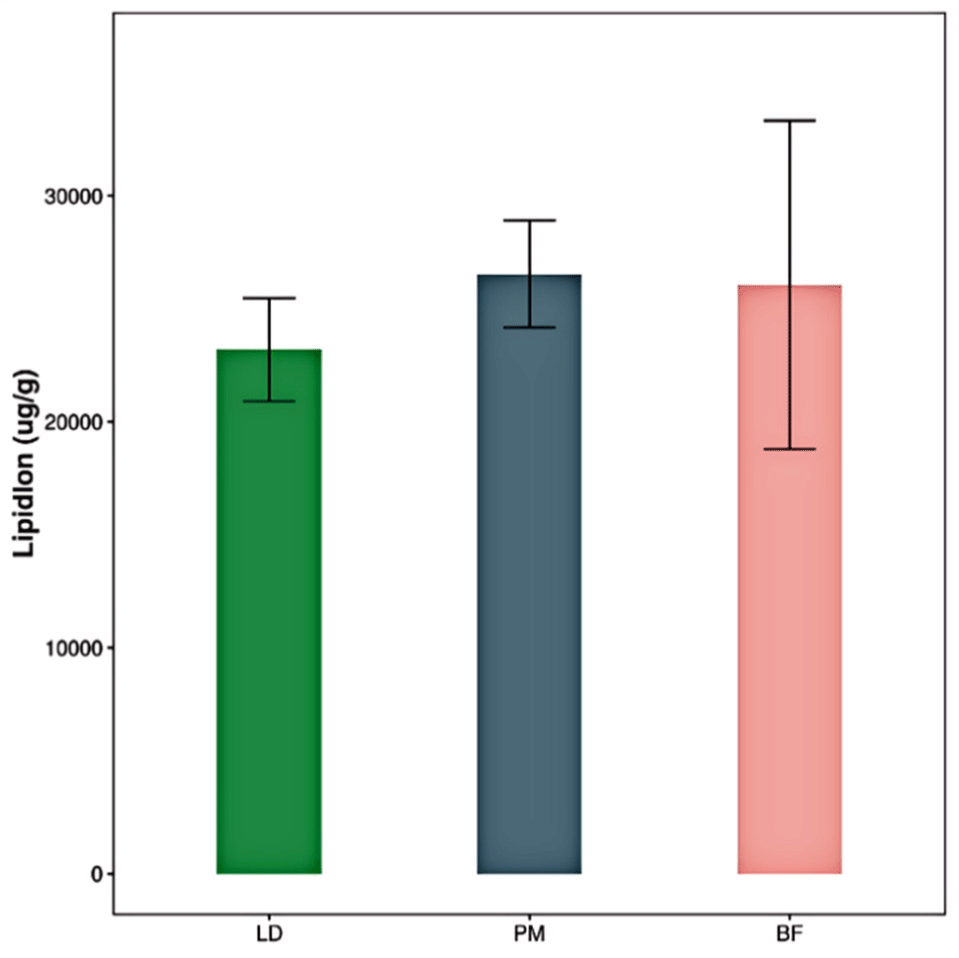
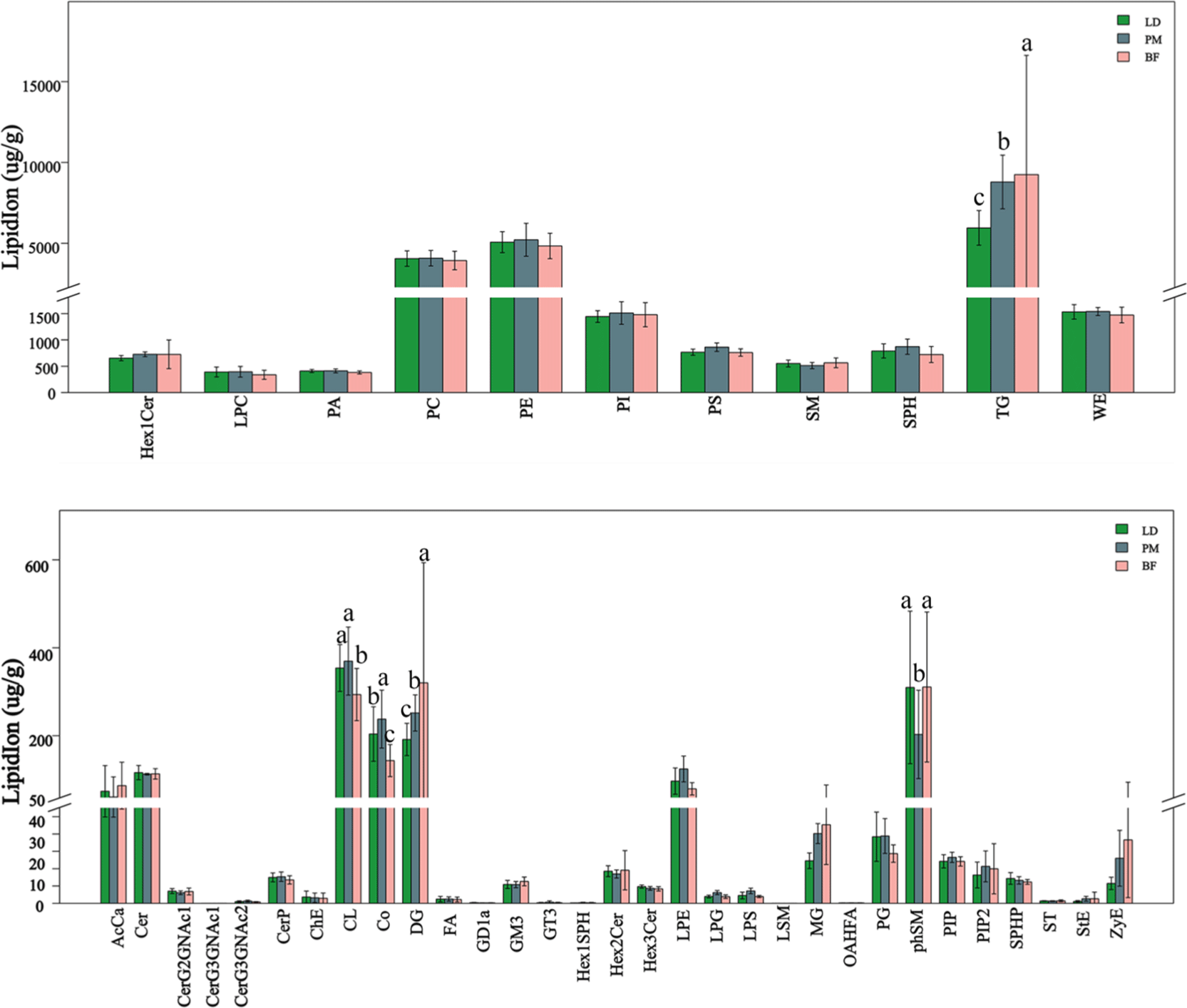
To study the significant differences in lipids between different parts of Chaka sheep, OPLS-DA model was used to evaluate differential lipids. The OPLS-DA model maximizes the between-group differences in the horizontal coordinate, while the within-group variation is reflected in the orthogonal principal components in the vertical coordinate, and it is clear in Fig. 6 that the OPLS-DA model can distinguish the two groups of samples, and the model evaluation parameter Q2<0.5, indicating that the model has a great deal of stability, and the test results are real and reliable (Zhang et al., 2022b).
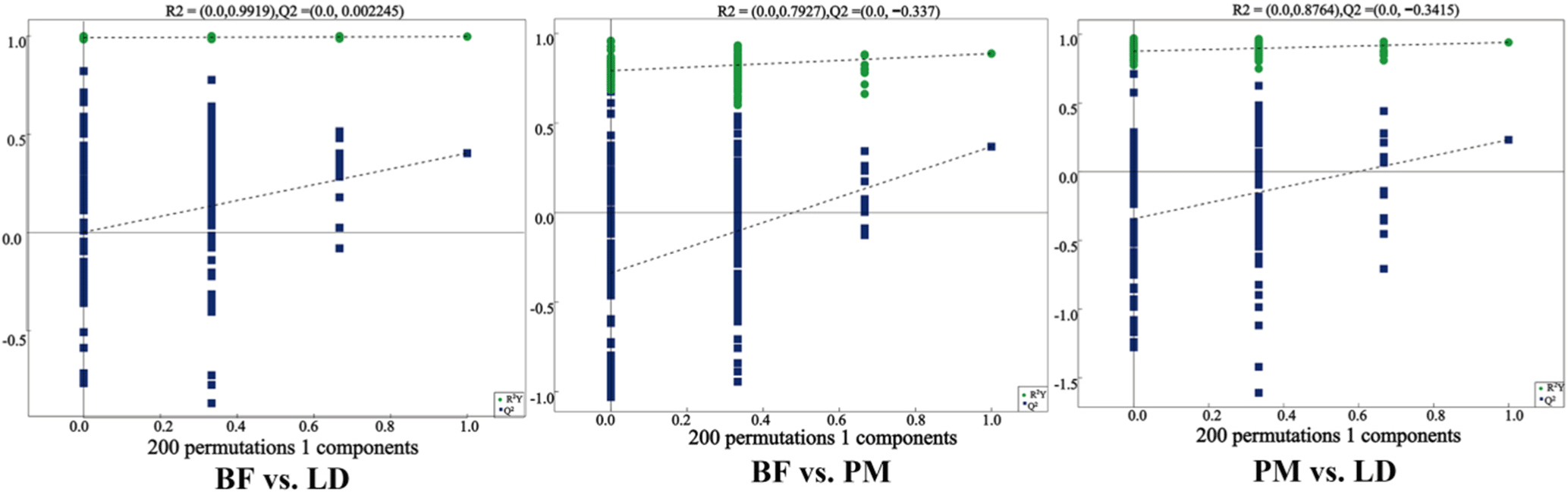
The significantly different lipid molecules (VIP>1, p<0.05) screened in this experiment were visualized in the form of bubble plots in Fig. 7. Bubbles in graph represent significantly different lipid species, and the vertical coordinates show each subclass of lipid, marked by different colors, with the size of the bubbles representing the significance of the differences. In BF, TG, PS, PI, PG, PE, PC, lysophosphatidylethanolamine (LPE), lysophosphatidylcholine (LPC), and Hex1Cer were the highly significantly different lipid molecules compared with LD. In BF vs. PM, TG, PS, PE, PC, LPE, LPC, and Hex1Cer were the main significantly different lipid molecules. In PM vs. LD, TG, SM, PS, PI, PC, LPC, Hex1Cer, and DG were the main lipids with highly significant differences. PS, PE, PC, LPC, LPE in PLs, TG in triglycerides, and Hex1Cer in SMs were the three major co-differentiating lipid molecules. In Sun’s study, PLs were found to be the main factor distinguishing the muscle lipidome between cattle-yak and yak (Sun et al., 2018; Vahmani et al., 2020), and our findings were consistent with these results. Jia’s study mainly detected AcCa, Cer, DG, LPC, PC, PE, SM, and TG, less ChE, LPE, MG, PI, and SPH, and no Co, Hex1Cer, Hex2Cer, LSM, PG in Tan sheep meat (Jia et al., 2021). However, in our study, we detected lipid molecules such as Co, Hex1Cer, Hex2Cer, LSM, and PG. These differences may be related to diet, feeding habits, and other factors (Dannenberger et al., 2017). The top 20 differential lipids in the different comparison groups are shown in the Supplementary Table S1.
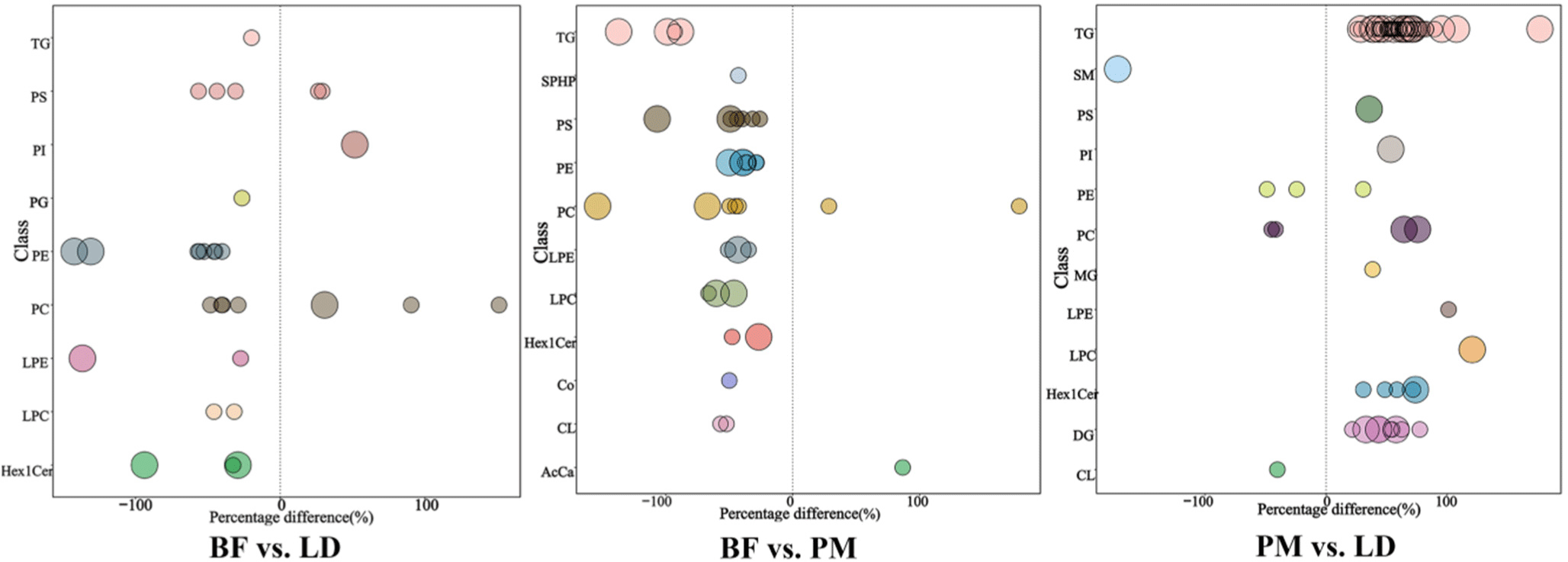
We used venn diagrams (Fig. 8) to show specific differential lipid molecules, and the results of the study showed that the numbers of significantly different lipid molecules between the different comparison groups were: 30 (BF vs. LD), 38 (BF vs. PM), and 88 (PM vs. LD). Among them, PE (18:2/18:2) and PC (25:0/11:3) were LD group-associated differential lipids. PS (20:3e/20:4), LPC (18:3), PE (8:1e/12:3), TG (18:0e/16:0/18:1), TG (18:0/18:0/18:0), TG (18:0e/18:0/18:1), and TG (18:0e/18:1/18:1) were PM group-associated differential lipids. LPC (16:0), LPC (18:1), LPE (18:1), PC (15:0/22:6), PE (18:1/18:1), Hex1Cer (d24:1/18:1), and PC (10:0e/6:0) were BF group-associated differential lipids. Dashdorj et al. (2015) found that PE (16:0/20:4), PE (18:0/19:1), and PE (18:1/22:5) were the top three lipid species, suggesting that they are the major contributors to shank/flank muscle liposome differentiation. In our study, PE (18:2/18:2) was mainly found in LD, PE (8:1e/12:3) was predominantly detected in PM, and PE (18:1/18:1) was mostly identified in BF. It can be concluded that PE is an important biomarker for the differentiation of the different parts of the muscle. Li et al. (2022b) showed that PLs containing PC and PE may be key lipids in the formation of aromatic substances in roasted lamb. Zhou et al. (2014) showed that PC is more easily oxidized to aldehydes due to its high content of unsaturated fatty acids. Guo’s study showed that PC and PE are prone to the formation of the aroma compounds (E,E)-2,4-decadienal, 1-octen-3-one and (E)-2-decene (Guo et al., 2021). Our study found significant differences in the content of the flavor compounds alcohols, ketones and esters among the three comparison groups. Therefore, we hypothesized that the cause of the differences in flavor compounds is related to the lipids PE (18:2/18:2), PE (8:1e/12:3), PE (18:1/18:1), PC (25:0/11:3), and PC (15:0/22:6). In addition, TG had a distinct influence on the volatile components and on the oral release of the most important hydrophilic volatile components. The fatty acid species contained in TG were found to affect the oxidation of volatile compounds (Frank et al., 2017). We found that volatile flavor alcohols, aldehydes, and esters were the most abundant in PM, while TG (18:0e/16:0/18:1), TG (18:0/18:0/18:0), TG (18:0e/18:0/18:1), and TG (18:0e/18:1/18:1) were the characteristic lipid molecules in PM. Alcohols have a higher taste threshold and less impact on the taste of meat compared to aldehydes (Huang et al., 2022). Studies have shown that aldehydes, usually derived from C18 PUFA such as linoleic and linolenic acids and C20 arachidonic acid, are the major products of lipid oxidation. Aldehydes have a low olfactory threshold and are important contributors to flavor (Varlet et al., 2007). Aldehydes were the highest percentage of flavor substances in our study and were significantly higher in the PM group than in the LD and BF groups. Therefore, we hypothesized that TG-like lipids are associated with the formation of aldehydes.
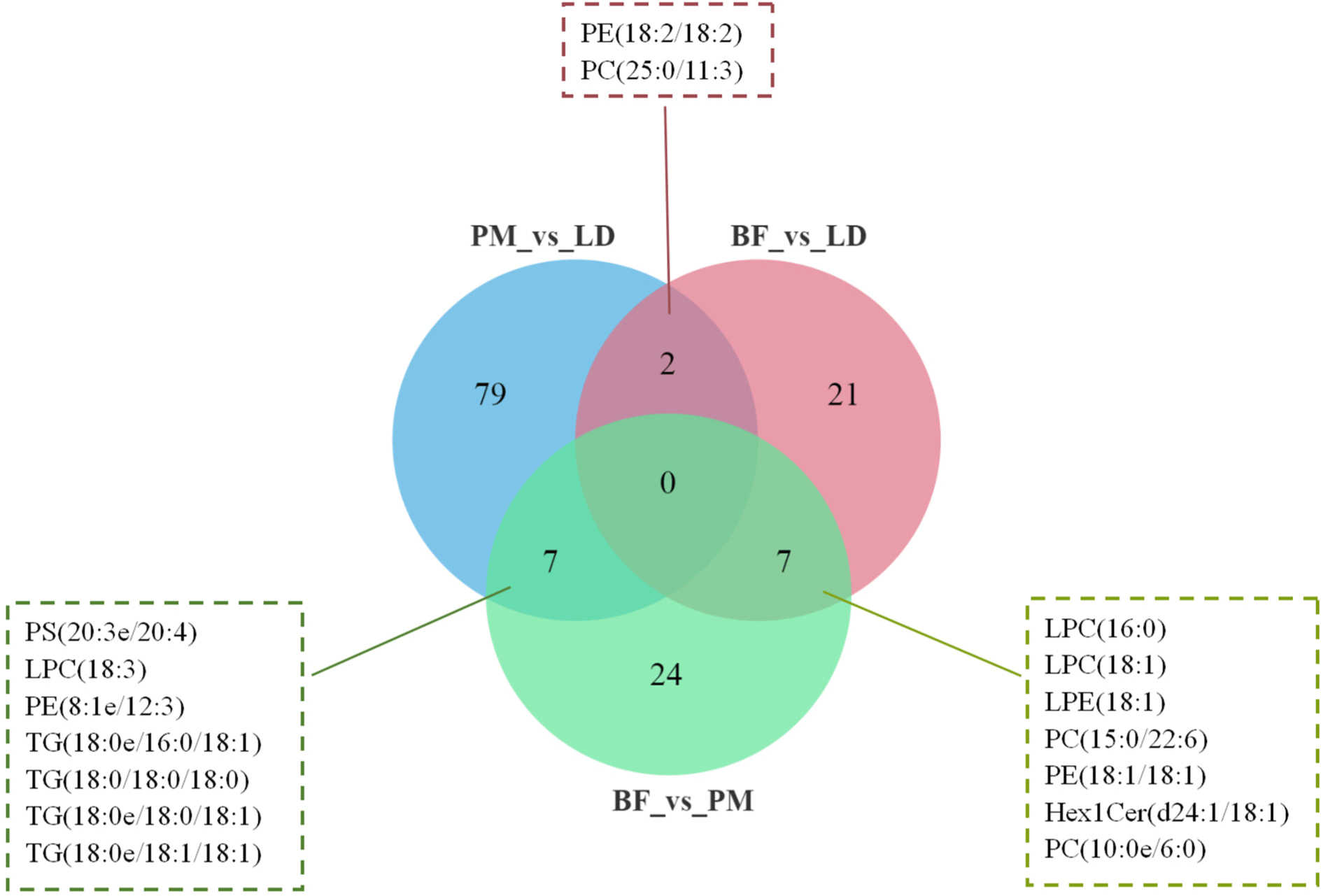
To evaluate the plausibility of differential lipids and to visualize more clearly the association between specimens and the differential expression patterns of lipids in different samples, we conducted hierarchical clustering (Fig. 9) using the expression levels of highly differential lipids (VIP>1, p-value<0.05) for each group of specimens. Differential lipids were analysed by clustering and heatmaps demonstrated significant differences in lipid profiles between the BF, LD and PM, indicating significant differences in lipid molecules in different parts of the muscle of Chaka sheep.
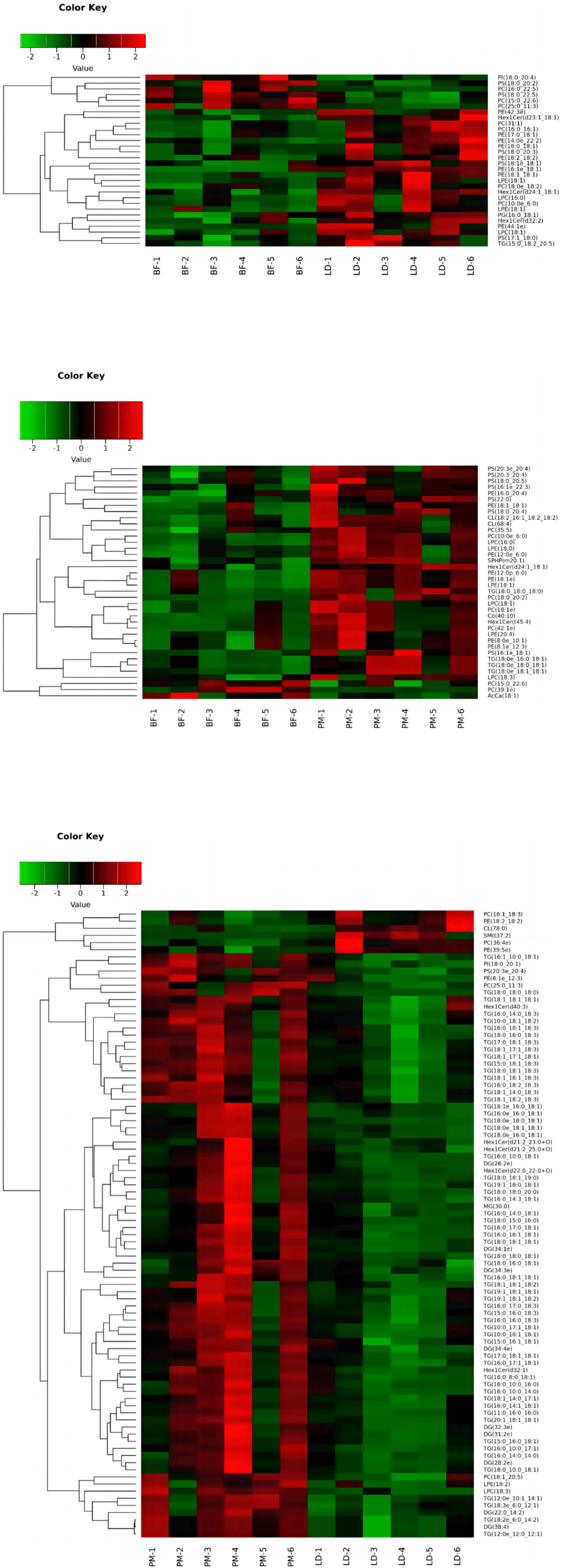
To show the relationship of lipid coregulation more intuitively, the chord diagram (Fig. 10) was utilized to show the correlation between lipid sub-classes, where the beginning point of the link in the inner circle represented the significantly different lipid molecules, and the bows on the outer circles indicated the lipid sub-classes. In BF vs. LD, our study reflected a strong correlation within lipid subclasses PC, PE, and Hex1Cer. By comparing the BF group with the PM group, we obtained some intramolecular correlations of lipid subclasses such as PC, PE, PS, TG, and CL. By comparing PM and LD, our results suggested that there were strong intramolecular correlations between the glycerol ester lipid subclass molecules TG and DG.
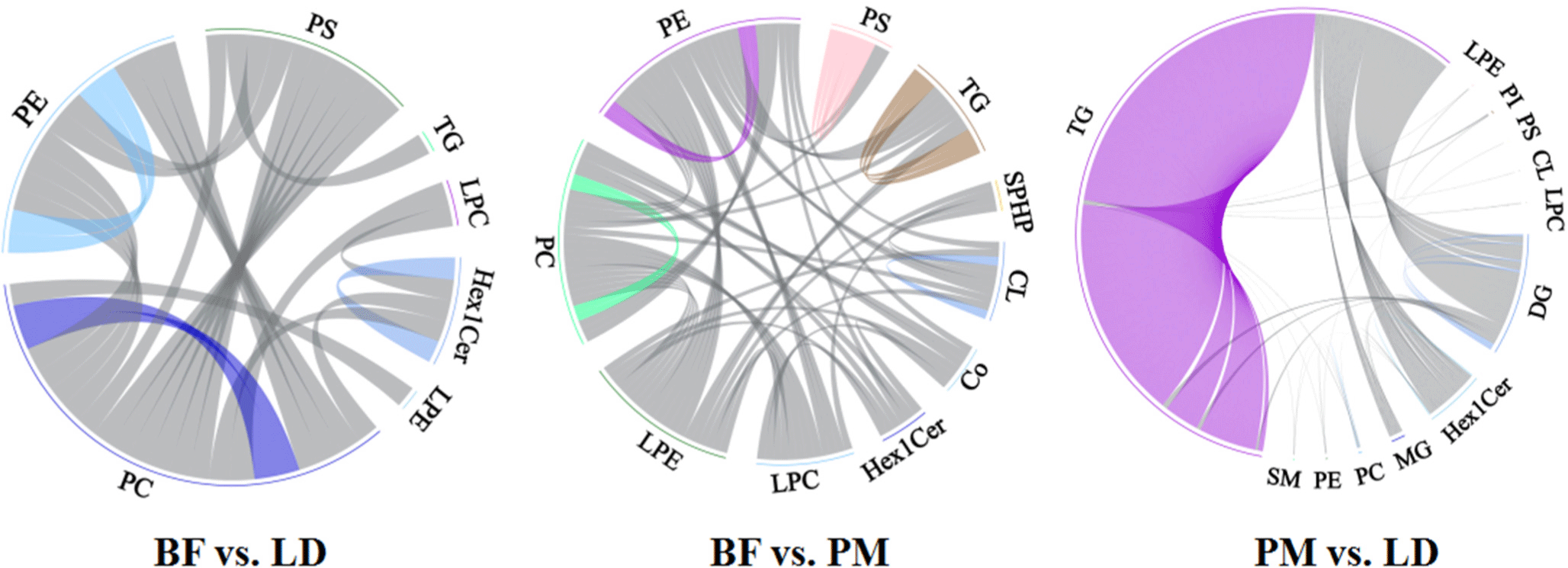
McMaster’s study showed that the majority of PE is synthesized from ethanolamines, while PE is a choline source. After the synthesis of PE, it can be converted to PC via sequential methylation steps (McMaster, 2018), which further validates the correlation between PC and PE in this study. Zhou’s study found that there was a strong correlation between PS and PI (Zhou et al., 2021). Hou et al. (2020) found a correlation between the expression of PSs and PIs in subcutaneous adipose tissue. However in our study, we did not find a strong correlation between PI and other lipid molecules, the relevance of other lipid molecules needs to be further verified.
Conclusion
In our study, we found differences in the composition of nutrients and lipid profile in three parts of the muscles of the Chaka sheep. Specifically, the LD had the highest CP content and the highest levels of aspartic acid and histidine, the BF had the highest contents of glycine and proline, and the PM had the highest abundance of ω-6 PUFA, linoleic acid, and volatile flavor compounds. PLs were the predominant lipids, which accounted for almost half of the total lipids. The lipid profiles were different in the LD, PM and BF of the Chaka sheep. There were obvious intermolecular relationships between PC, PE, Hex1Cer, PS, TG, DG, and CL. This work is aimed at characterizing the nutritional composition and lipid profile of the Chaka sheep. Our findings may open new horizons in the utilization of the Chaka sheep for nutritional or biomedical products, thus enhancing the commercial value of these products.